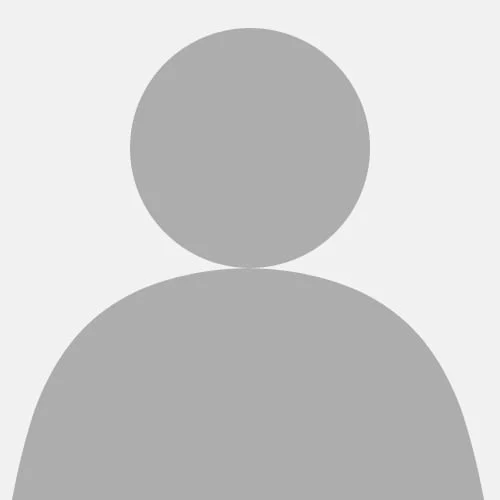
Dani Kennedy
Our project is centred around creating a prototype model that measures seawater after interaction with biomass-filled sorbent bags within a container (batch). The prototype will down-scale the cooling seawater downstream from a nuclear power facility (cooling water pool) with the addition of sorption material. The composition of seawater and numerous sensors positioned after the sample will contribute to the analysis of uranium that has been sorbed onto the biomass in the contacted bags in the batch. The understanding that certain biomass species attract uranium particulates exposes a possibility of scaling the prototype to nuclear power stations in the UK. The detection of uranium contents in seawater via a self-designed: optical filter (covering a large spectrum where uranium presents the best absorption), photodiode detection of excited uranium emitted photons and transistor amplification circuit. This research has led to an exciting challenge of U emission lifetimes in seawater due to the formation of Uranyl Calcium Carbonate that may be analysed in detail. Discoveries made could aid in formulating new and exciting nuclear fuel extraction methods that are sustainable and fully renewable.
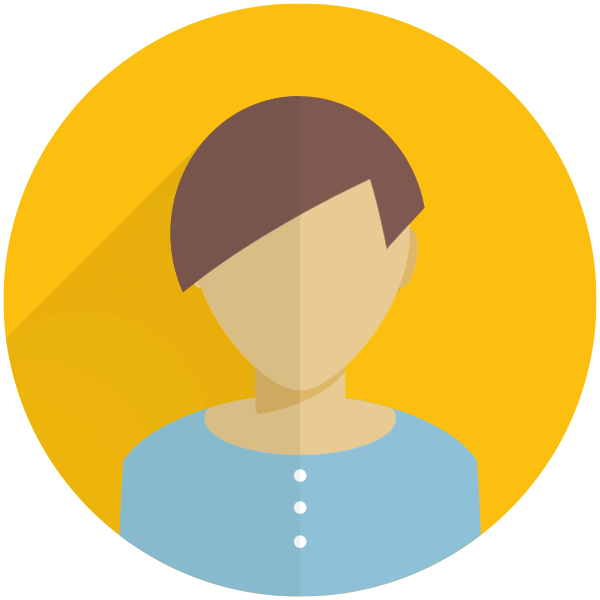
Dani Kennedy, Stephen Byrne, Ryan McAtasney
Nuclear energy is a growing sector around the world due to the demand for new renewable energy sources. The conversion of nuclear energy is completed using steam turbines and a cold source, often in the form of cold water. In order to run sustainably the conversion process also requires the utilisation of uranium to be successfully implemented. Uranium exists in miniscule quantities in seawater but can be recovered and re-worked into the system to produce a fully sustainable process. In nuclear energy, molten salt reactors (MSRs) are used to produce heat but also transporting it to the power plant. An efficient MSR consumes a minimum amount of fuel (i.e., uranium) whilst still producing a maximum amount of thermal and electrical energy. The best way to achieve this is through strict neutron economy to favour fission rather than capture through the use of fast neutrons.
In 2020, 16% of the UK's electricity was supplied using nuclear power plants. There are 13 operational sites in the UK which are highly regulated in terms of their energy output, both thermal and electrical. The majority of UK sites are AGR (advanced gas cooled reactors), but this will eventually become phased out due to the shift towards the use of PWRs (pressurised water reactors). The system being produced in this project, is being designed with the intention for application in a range of reactor types. Key aspects for the prototype are centred around keeping price low and easy implmentation, therefore, the compactness and portability of the device are critical.
Figure 1 : Process of nuclear energy conversion using a coolant source
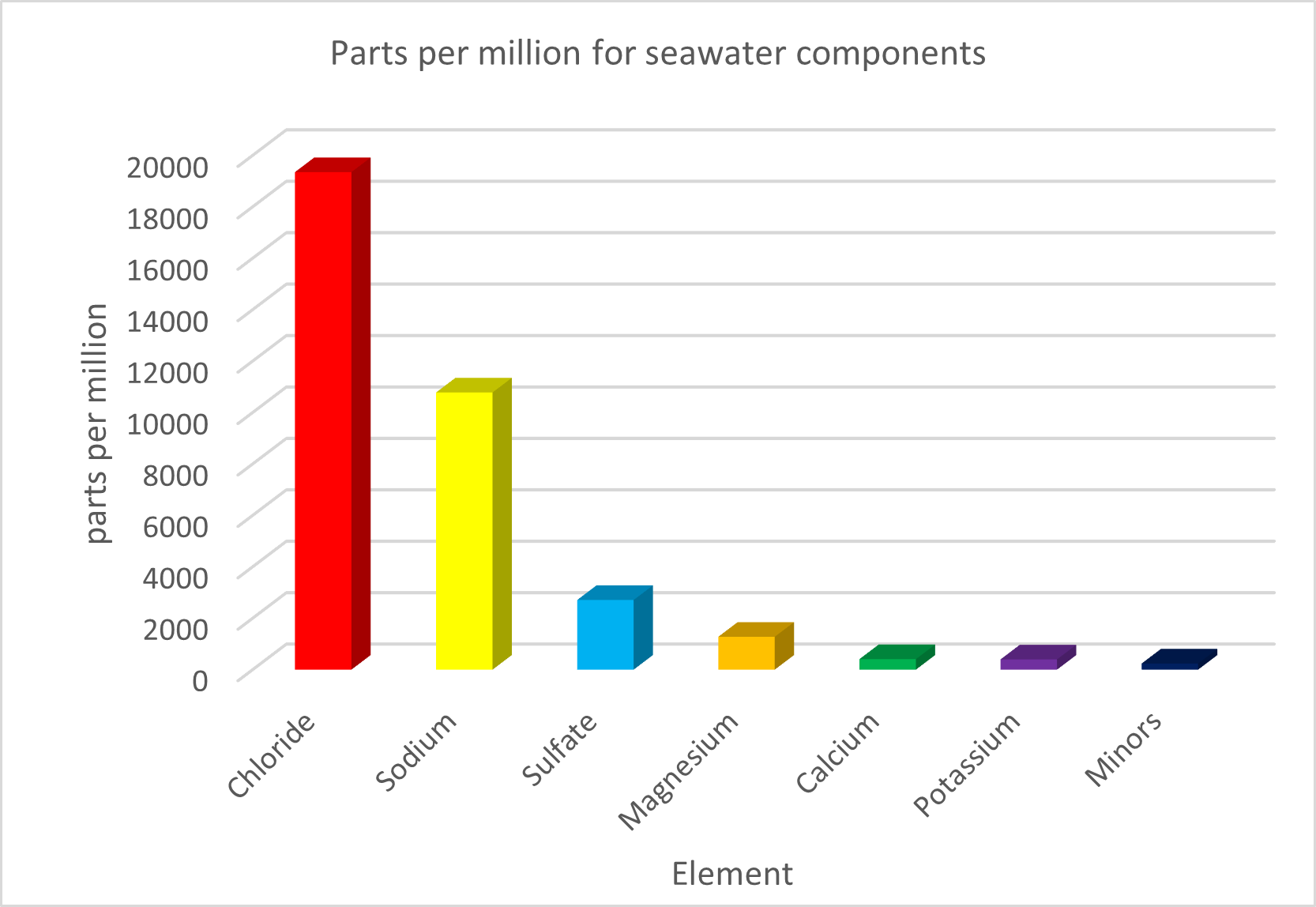
Figure 2: Constituents of seawater, measured in parts per million (ppm)
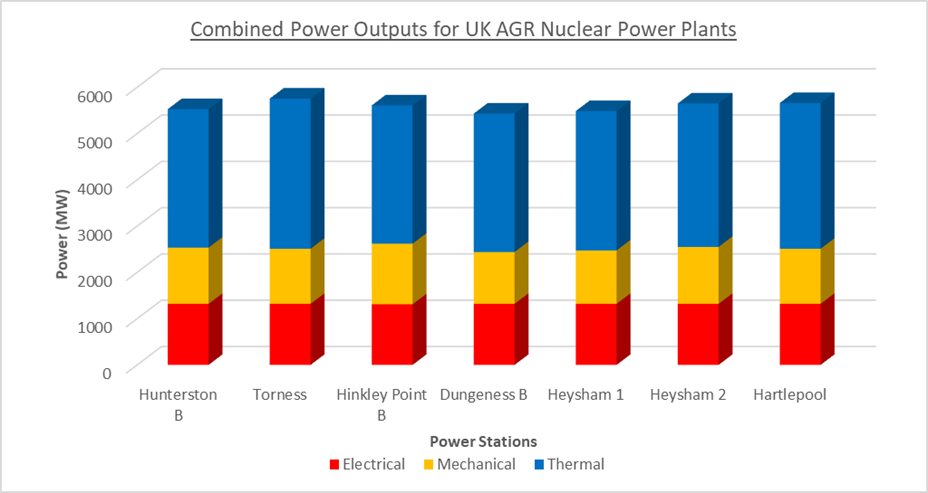
Figure 3 : Stacked graph showing the power outputs for different UK nuclear power stations
Figure 4 : CAD model of the laboratory setup required to achieve downhill flow
- Excitation Source
- Excitation Path guidance
- Photon detectors
- Analysis circuitry
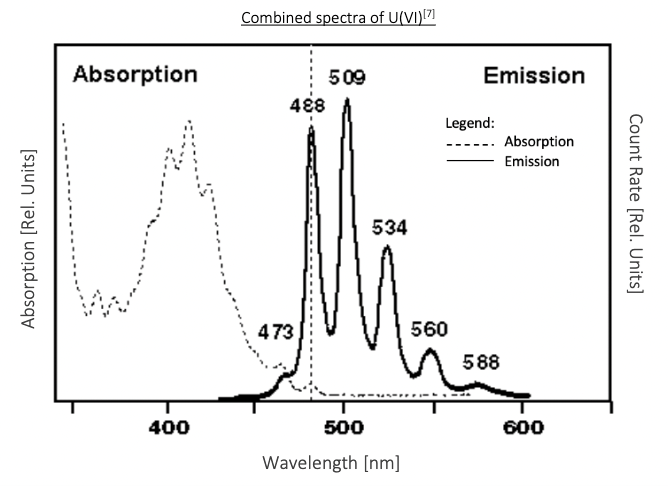
Figure 5 : Excitation and Emission Peaks for Uranium
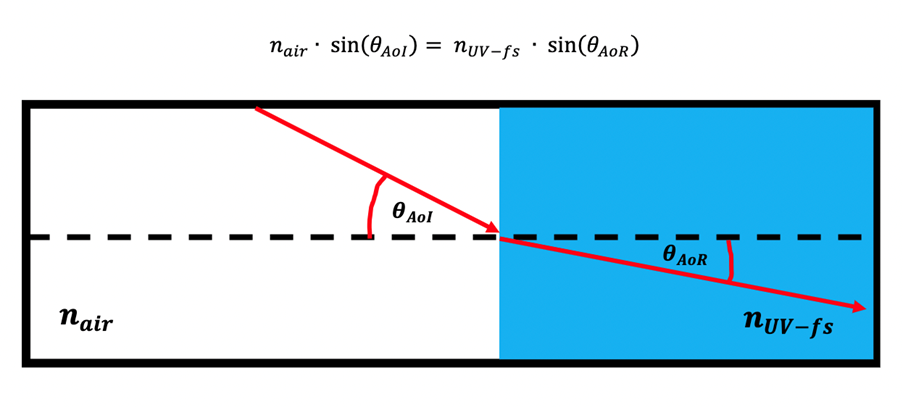
Figure 6 : Principles of Snell's Law
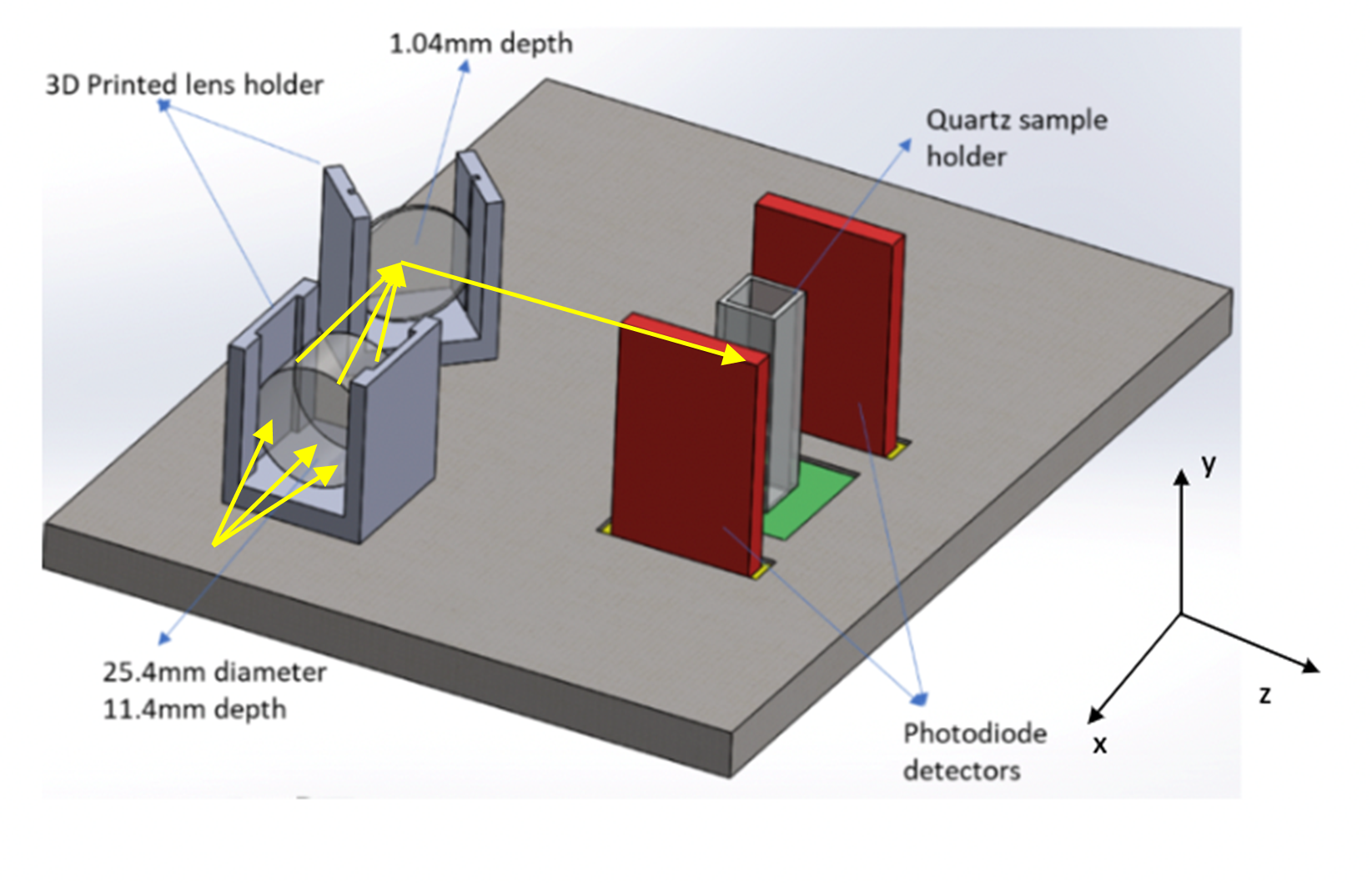
Figure 7 : Lens setup used in laboratory, created in CAD
Vout = 5V - (Rx * Itot)
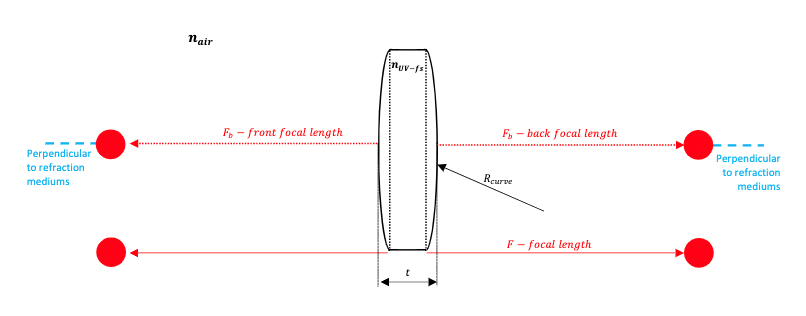
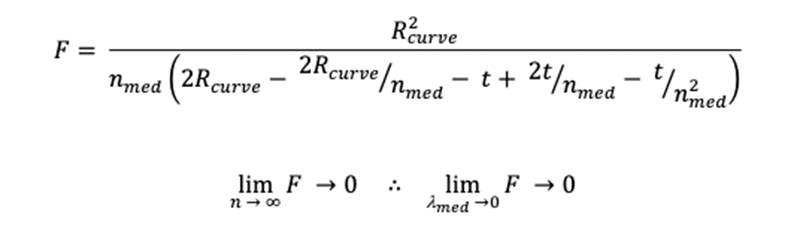
Figure 8 : Determination of lens focal point
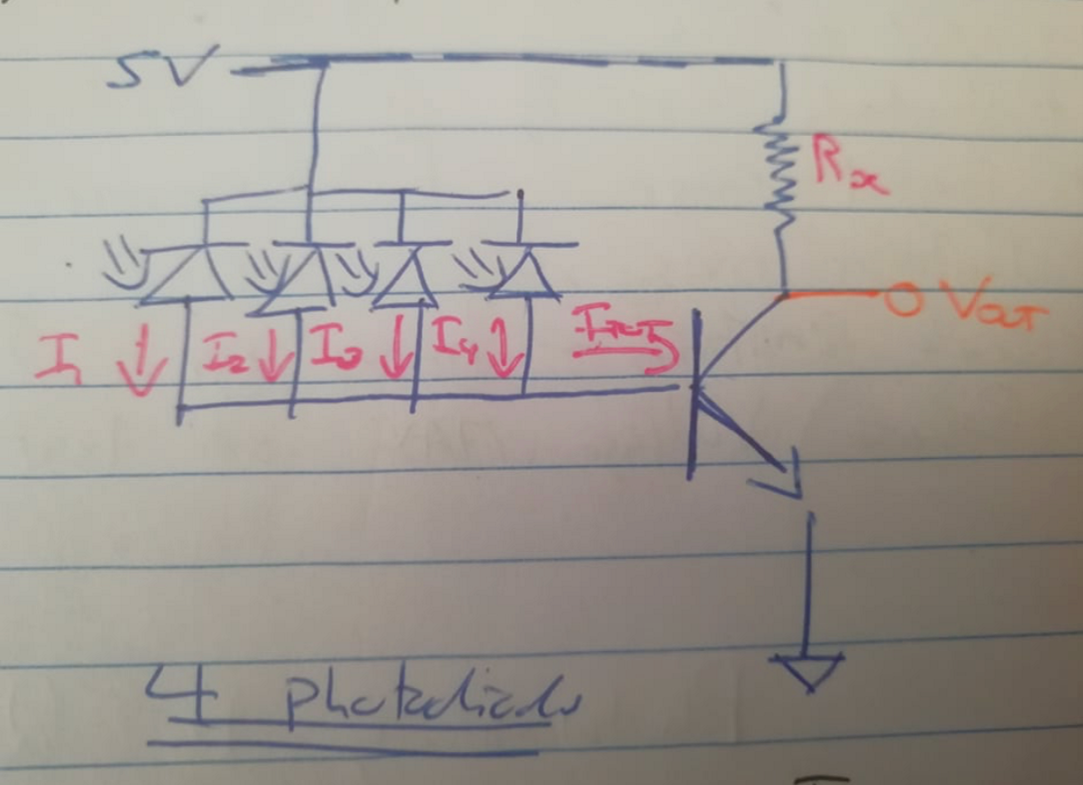
Figure 9 : Circuitry for the implementation of four pulsing photodiodes in parallel
- Calibration: The calibration process is essential for ensuring that the electrodes are behaving as expected, hence making sure that the readings being observed are accurate. Also, electrodes have been observed to become less effective over time and the resistance changes, so it is important to check regularly for this. The entire system should be periodically recalibrated to ensure that values being recorded are always correct.
- pH: The pH of seawater ranges between 7.5 to 8.5 dependent on its local salinity and levels will increase with salinity until the water reaches calcium carbonate saturation. Studies into the removal of uranium from aqueous mediums have concluded that pH affects uranium biosorption by both microorganisms and biowastes such as orange peel. M.A Mahmoud found that percent of uranium removal from seawater onto orange peel was at its’ lowest at low pH’s (1-3) before increasing until a maximum at a pH of 4. This is due to the hydrogen ions competing with uranium ions for adsorption sites within the orange peel. However, after pH 4 the percent of removal was found to gradually decrease from 95.50% to 75.45% at a pH of 8. This is a consequence of the formation of stable complexes with carbonates such as UO2CO3 and [UOZCO3]2- in more alkaline conditions.
- Oxygen Potential: The amount of dissolved oxygen in seawater greatly depends on the types of life forms present as well as the photosynthetic activity. The oxygen content is of interest in this study because it will inform as to whether or not the growth of bioorganic life is increasing, and this may have an effect on the uranium content within the seawater. In the laboratory setting there is unlikely to be much fluctuation, however upon scaling up this variation and subsequent effects will be more pronounced.
- Salinity: Typically, a conductivity sensor is used to measure salinity because a direct link can be drawn between the amount of electrical current being detected and the salt content present in a medium. Seawater is not uniformly saline throughout the world, with the main determining factor being the relative evaporation and precipitation of an area. The depletion of salt dilutes the concentration of uranium in the seawater which is significant for the real-world application with regards to ideal locations/depths to place biomass sorbents. There is also an implication for the collection of seawater in this study, ensuring that it is not diluted by recent precipitation.
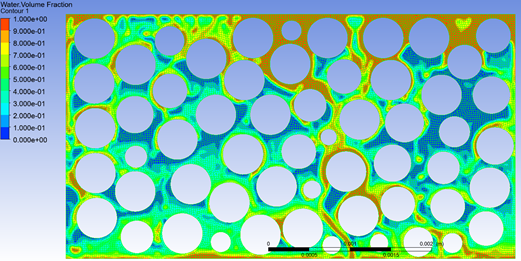
Figure 10 : Porosity study for sorbent bag, undertaken using CFD
Figure 11 : Water tank simualtion, undertaken using CFD
- Turbidity: A turbidity sensor is used in order to ensure that the teabag structure encasing the biomass remains intact as a tear would increase the turbidity of the water. An increase in turbidity above a pre-determined threshold triggers an alarm notifying about the potential issue which can then be resolved. In an experiment in the Kinta River, Selamat et al found a strong correlation of 0.915 between the concentration of uranium with turbidity. This is a further reason to monitor turbidity in the study.
- Level: In order to prevent damage to the probes on the sensory unit, they must be submerged in water/encased in solution at all times. Therefore, an additional risk aversion measure is taken with the installation of a level sensor external to the box. This device will send push notification to mobile devices through Arduino, alerting the group to potential issues so they can be adjusted accordingly.
-
- LED array instead of xenon lamp
-
- Photodiodes for detection instead of more expensive methods such as a PMT
-
- Electing to 3D print holders, box for minimal cost rather than purchasing
-
- Continuous modification of fluorometer design, swapping out expensive mirrors
-
- Using readily available departmental apparatus wherever possible
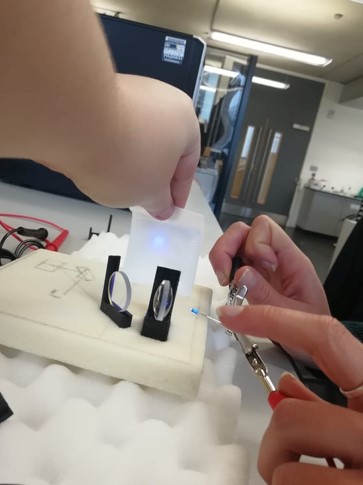
Figure 12 : Light convergence through lenses; prototype setup on foam
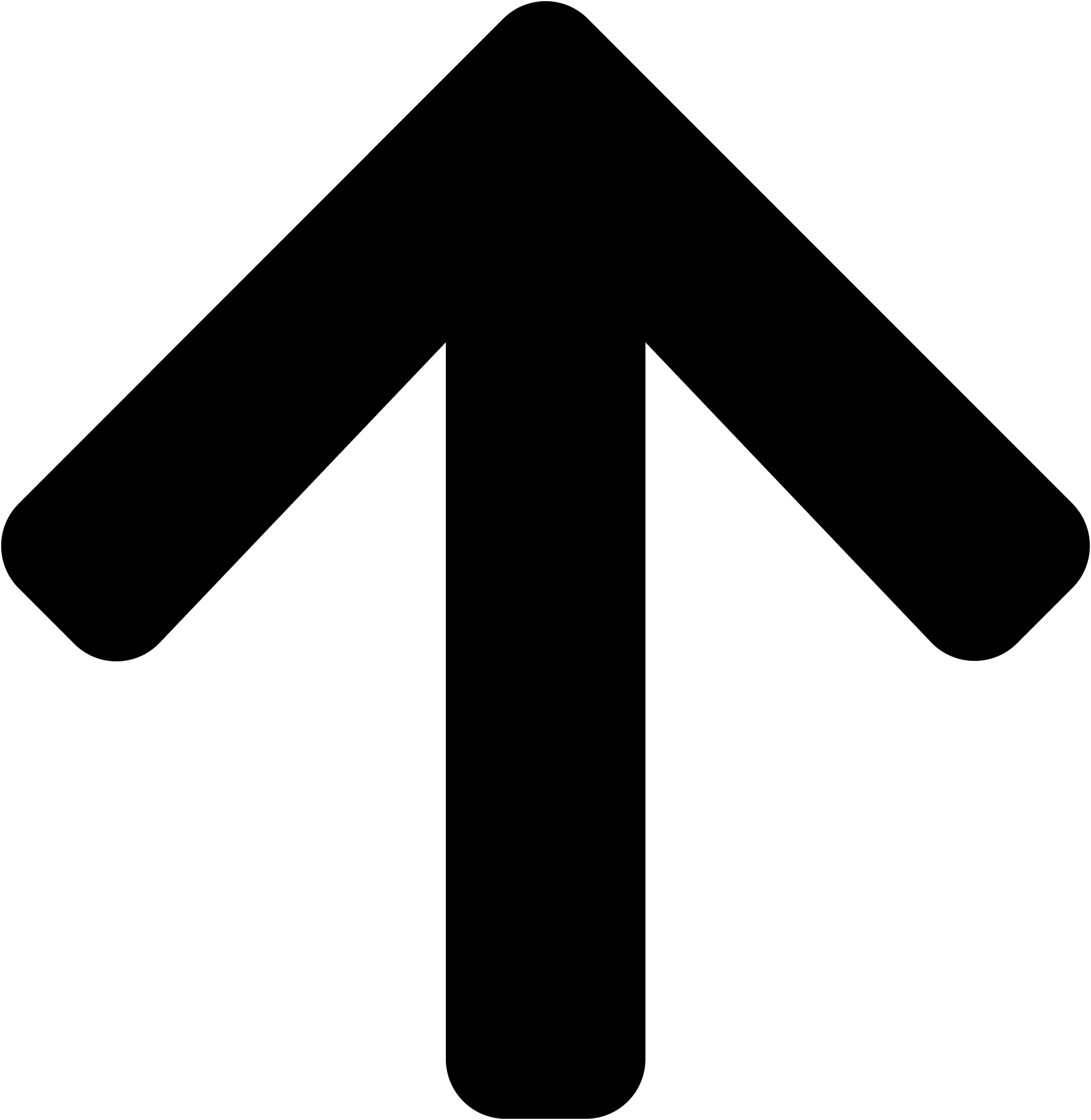
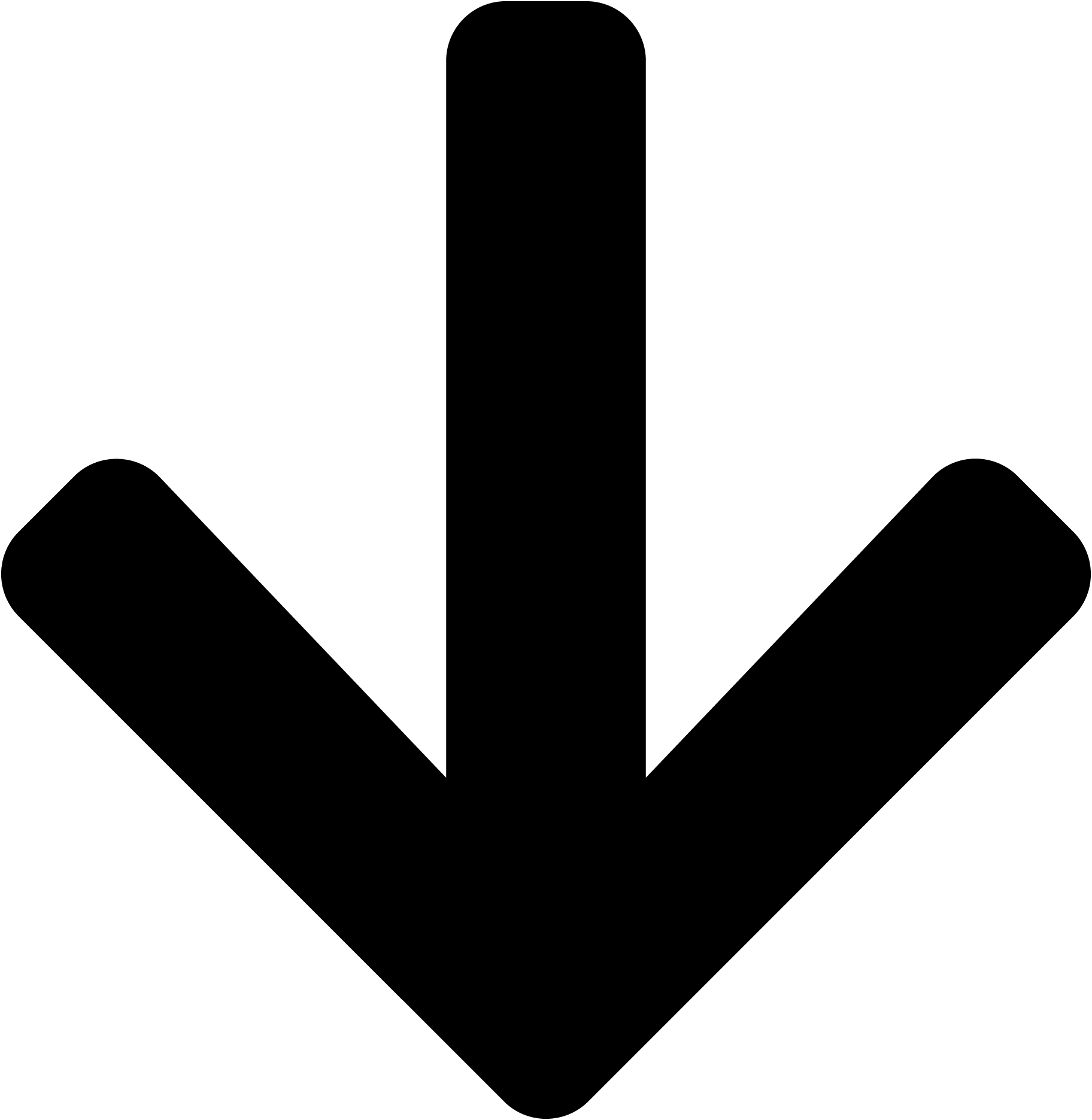
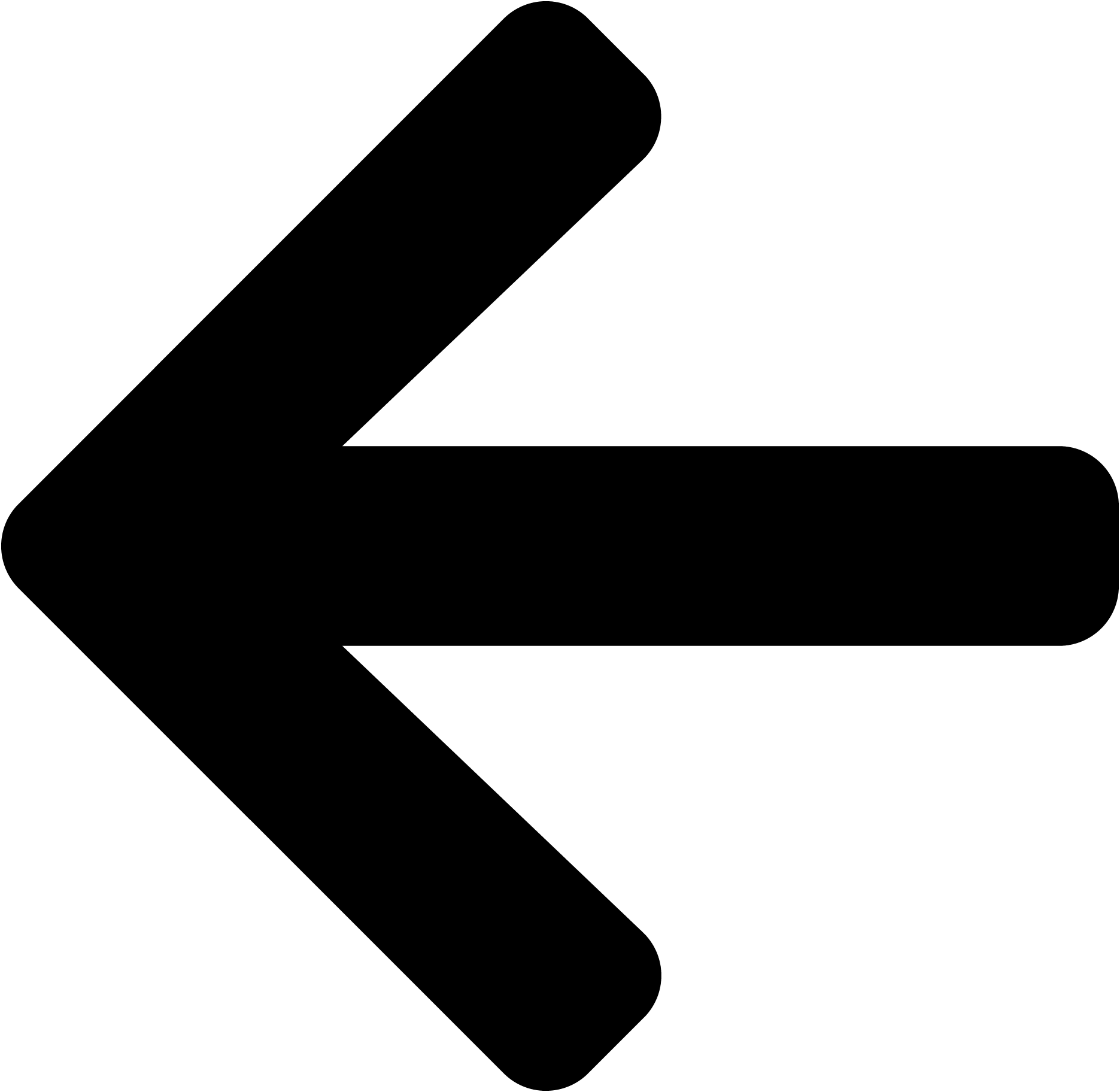
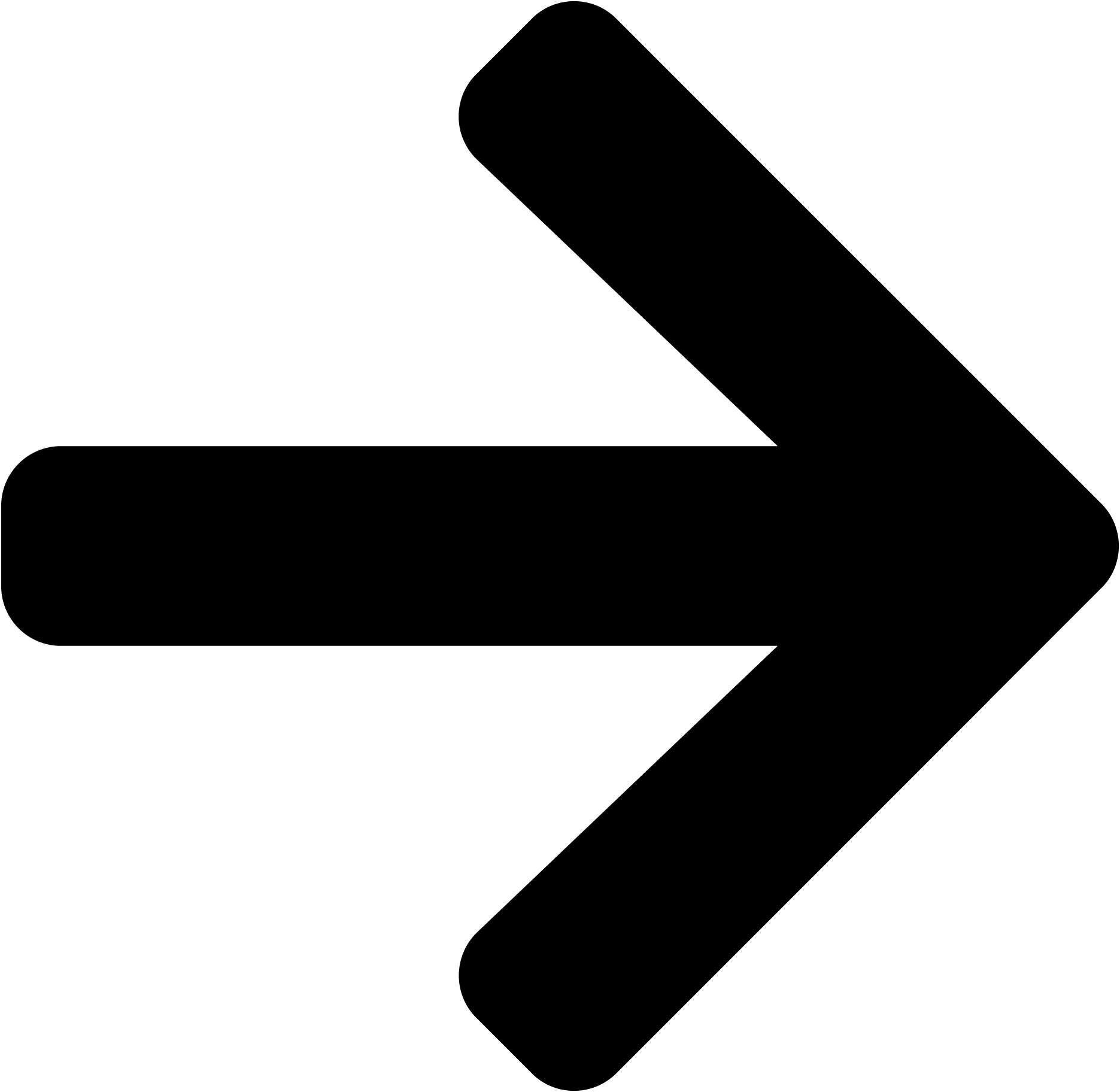
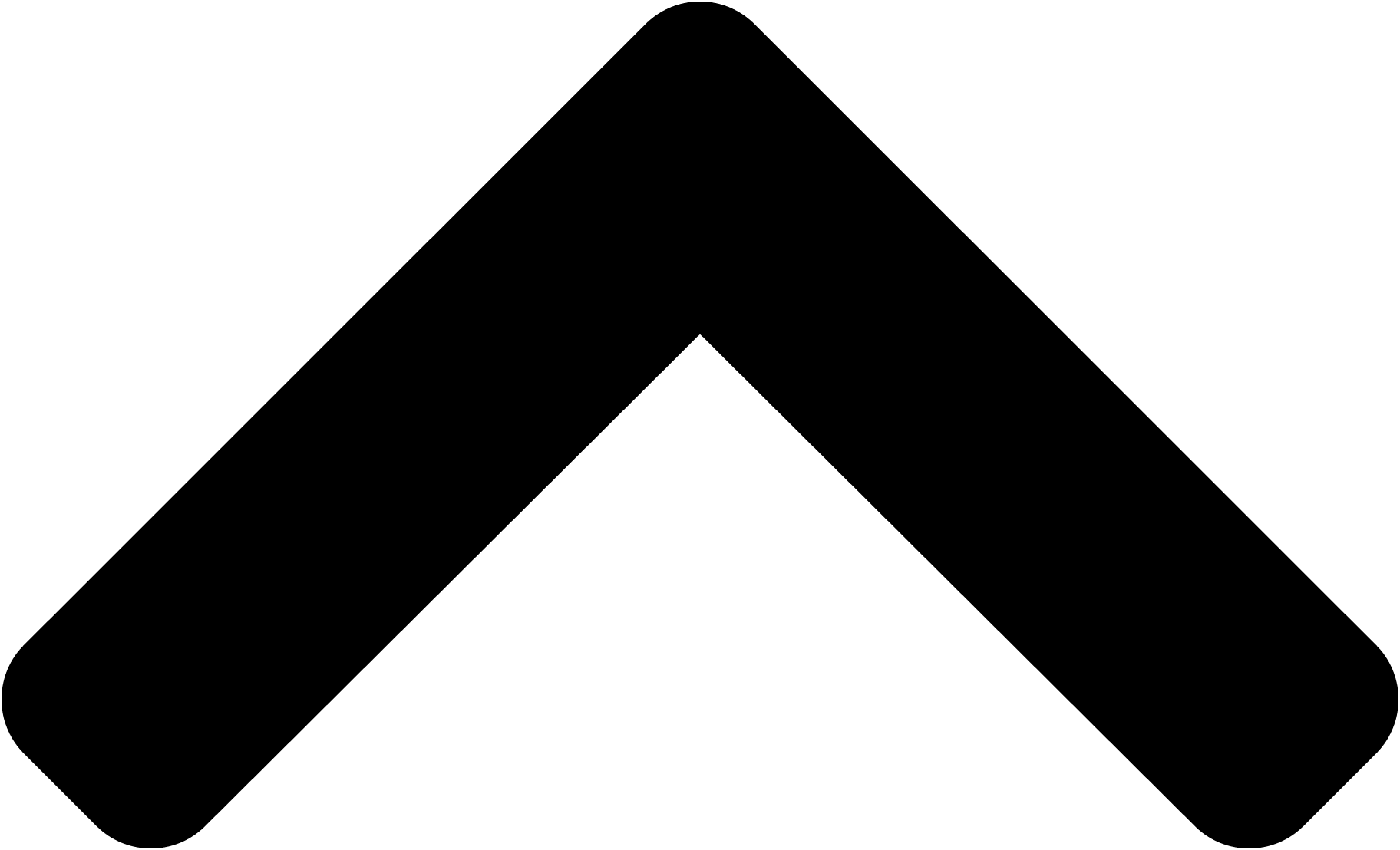
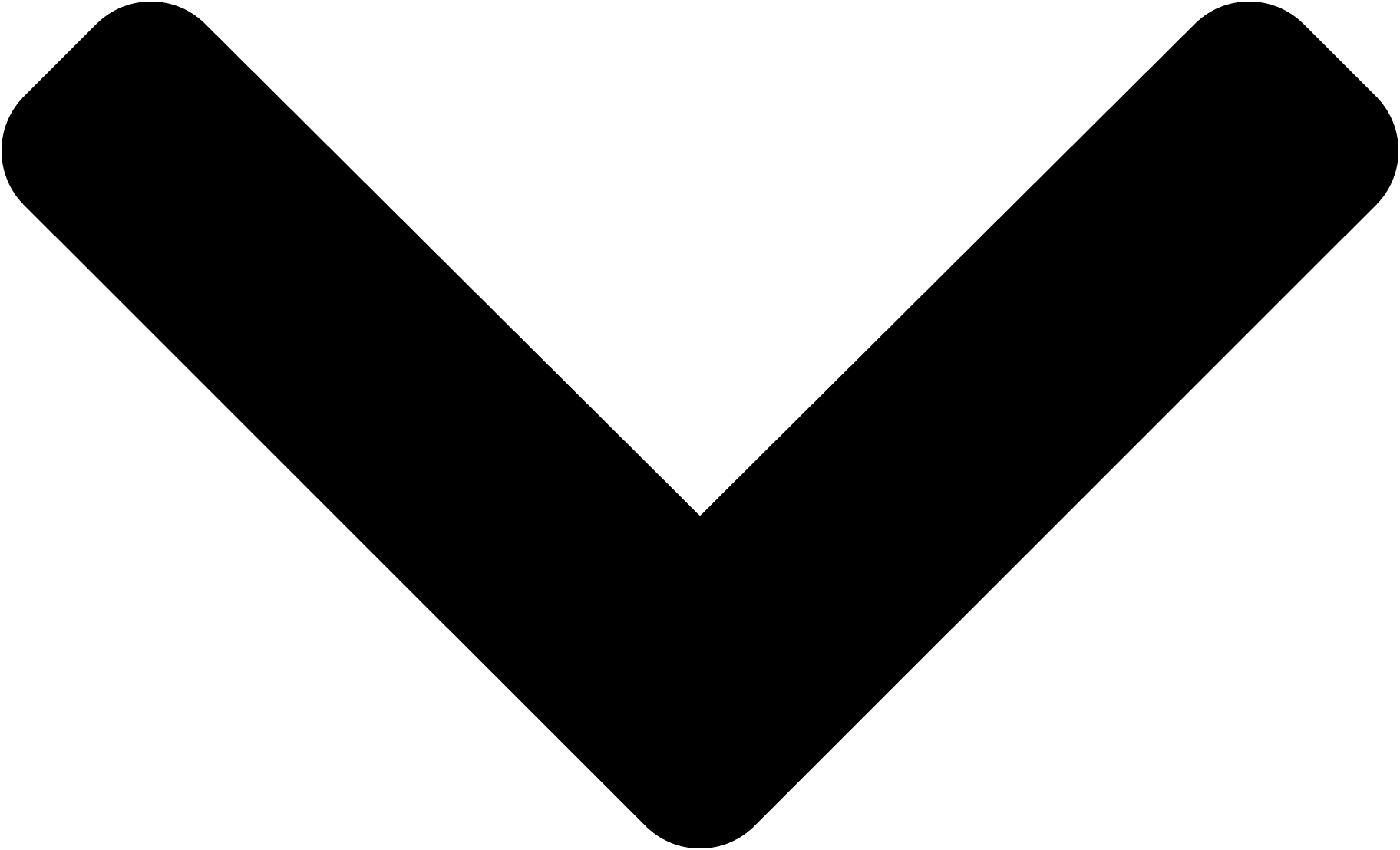
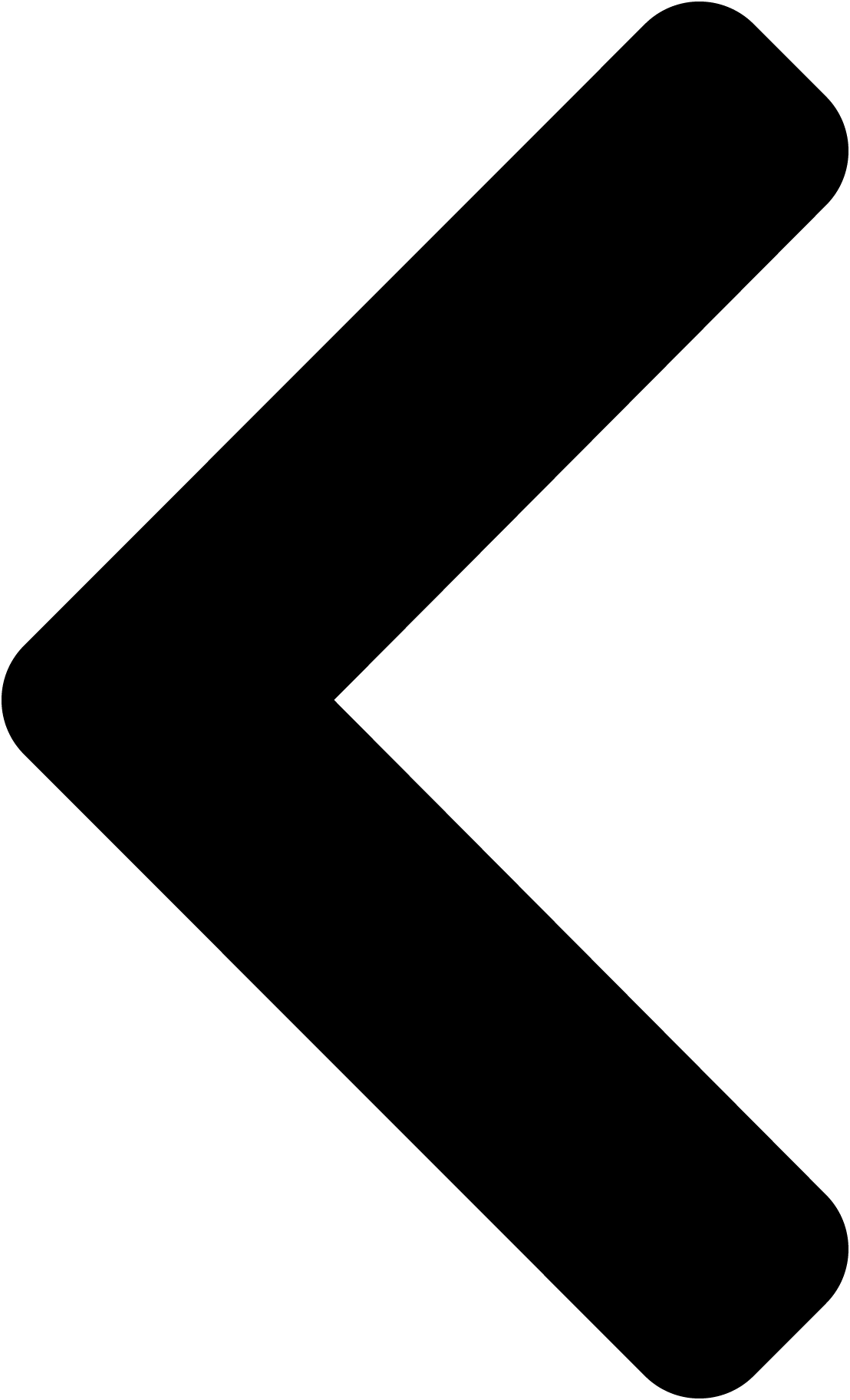
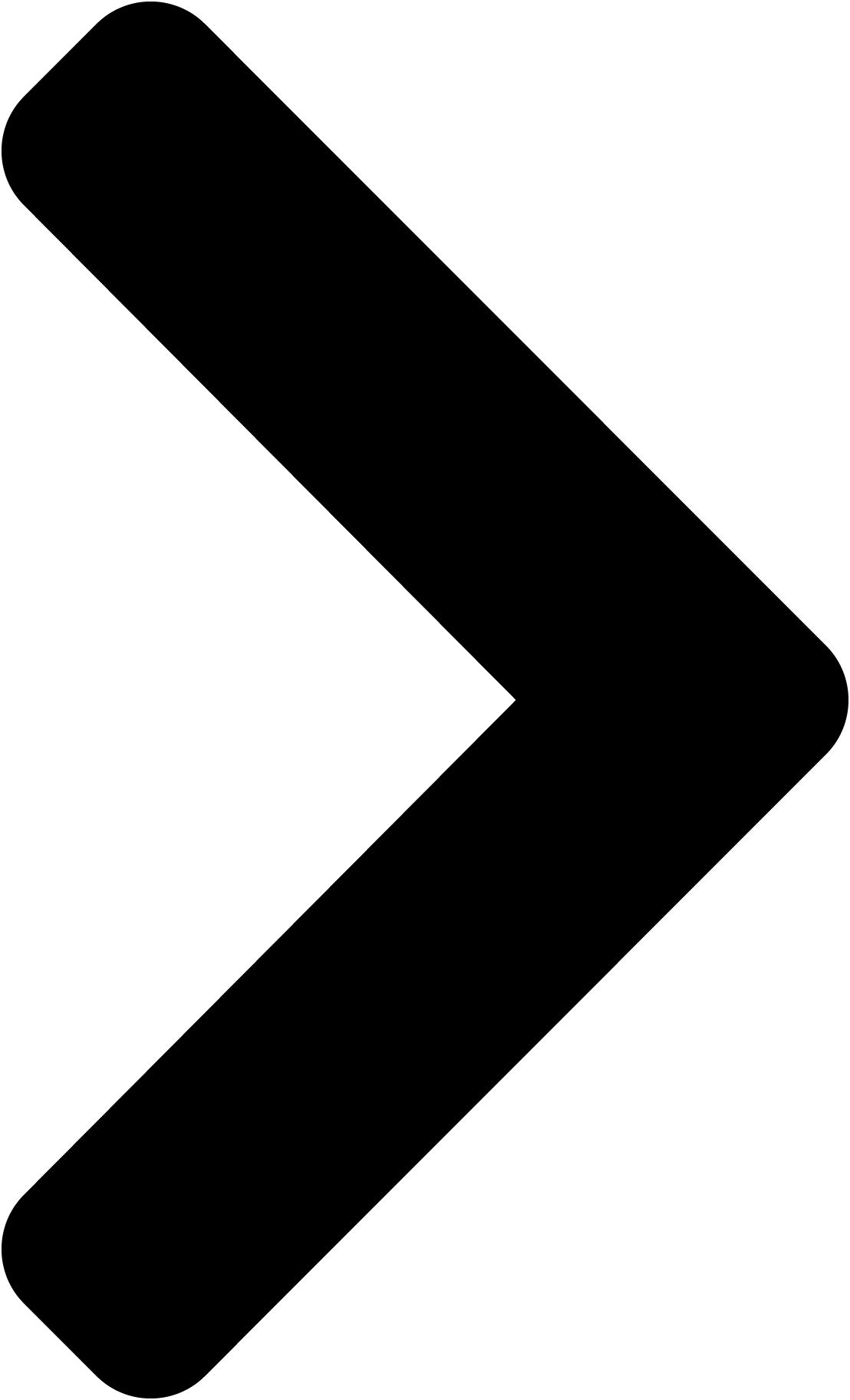
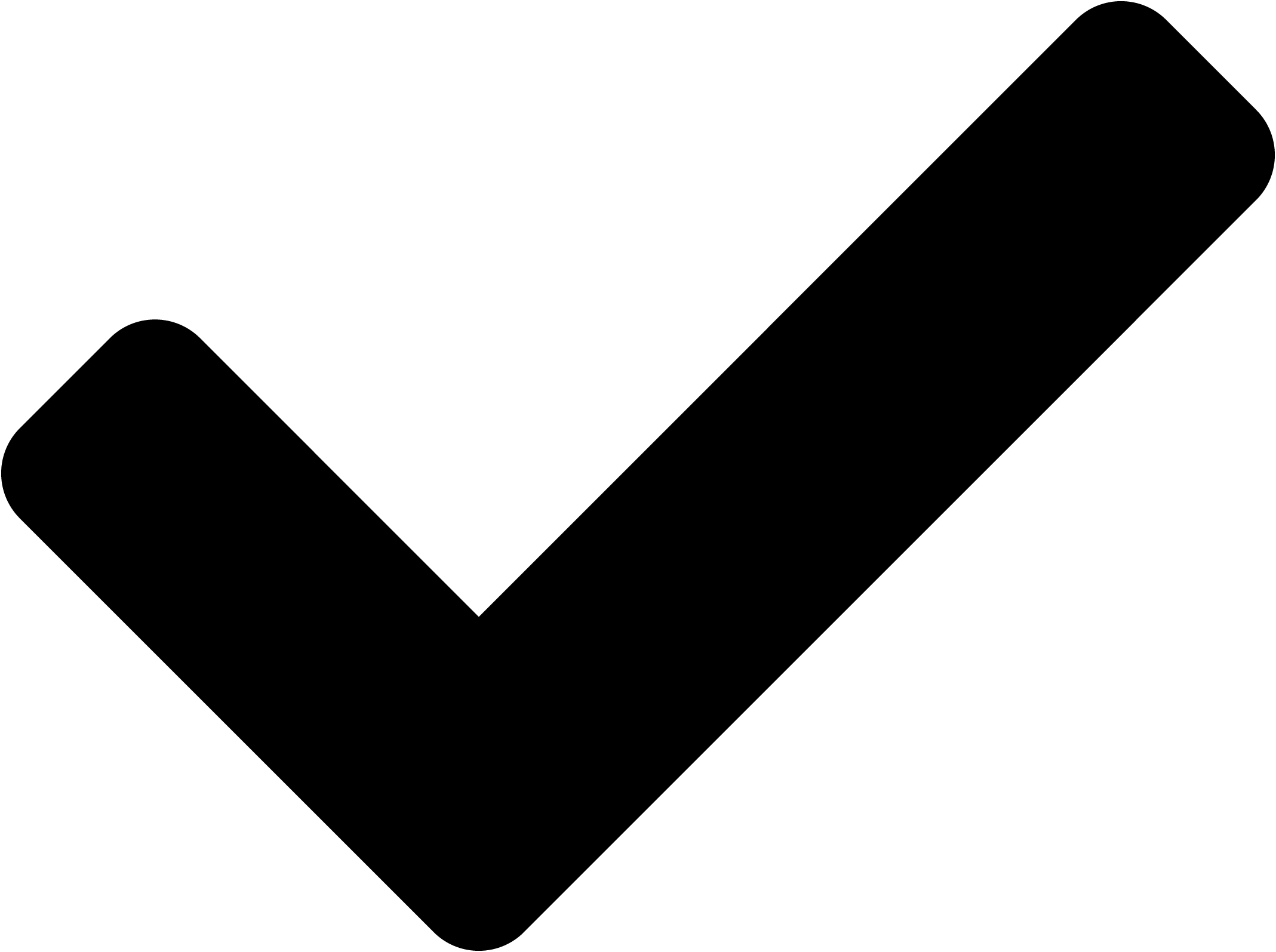
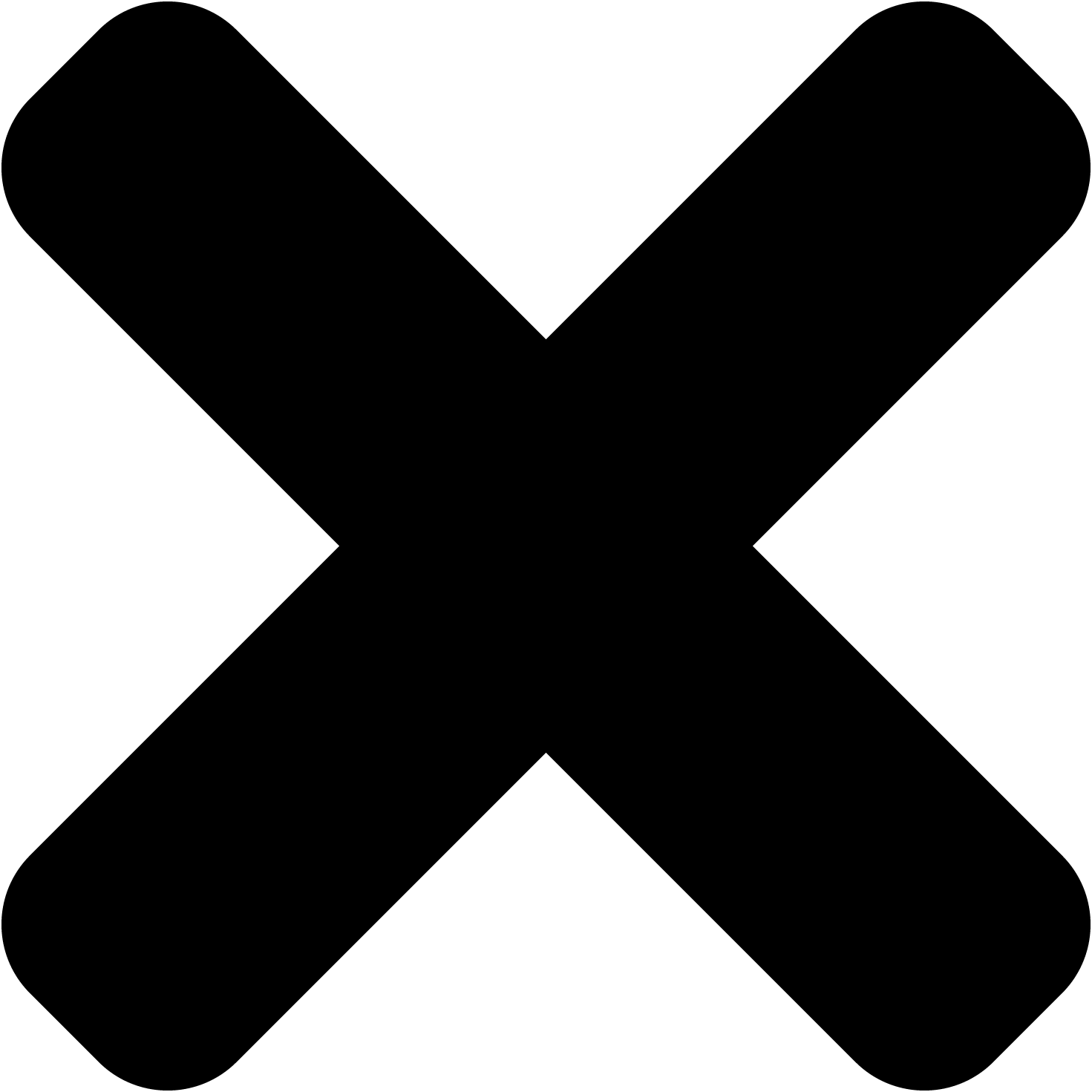
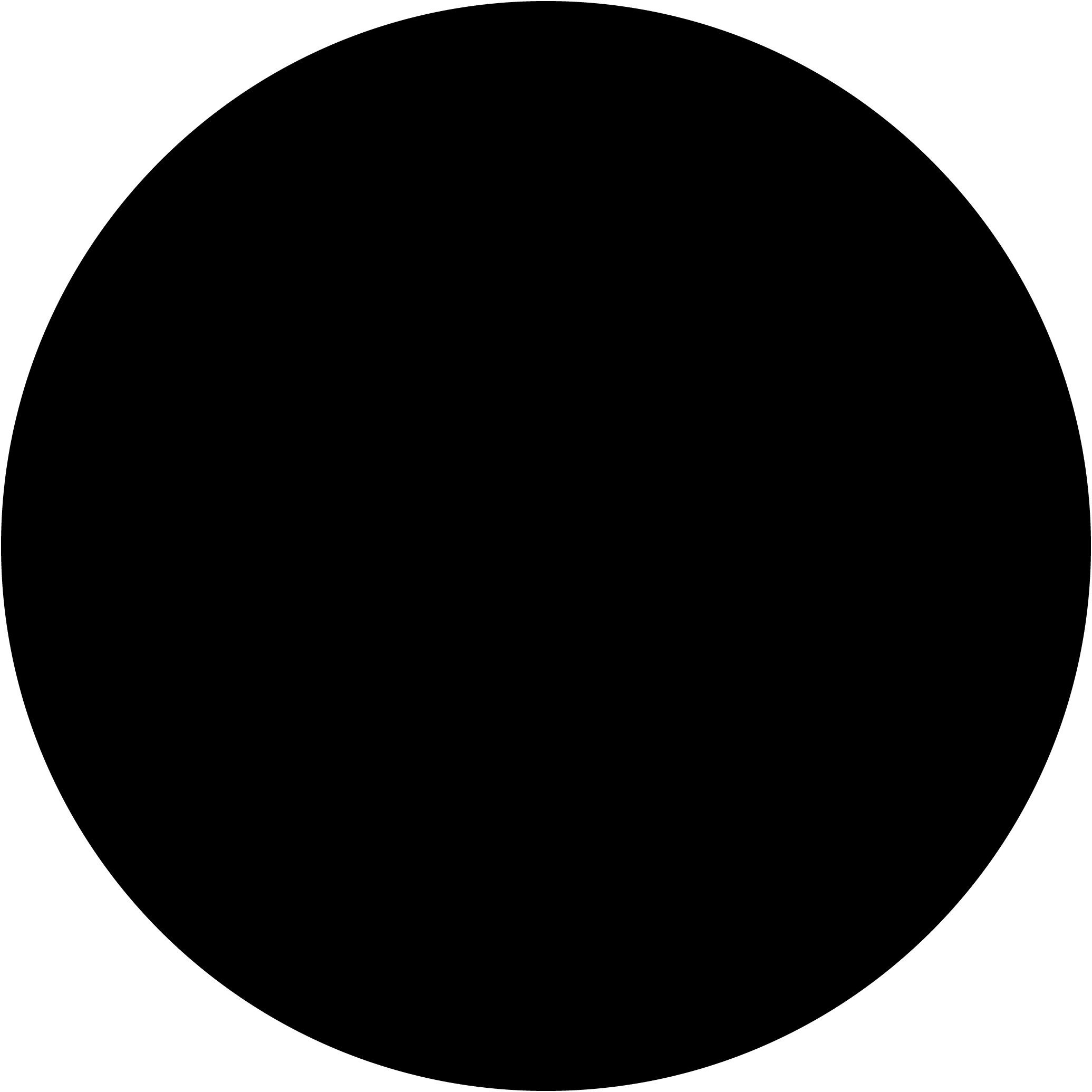
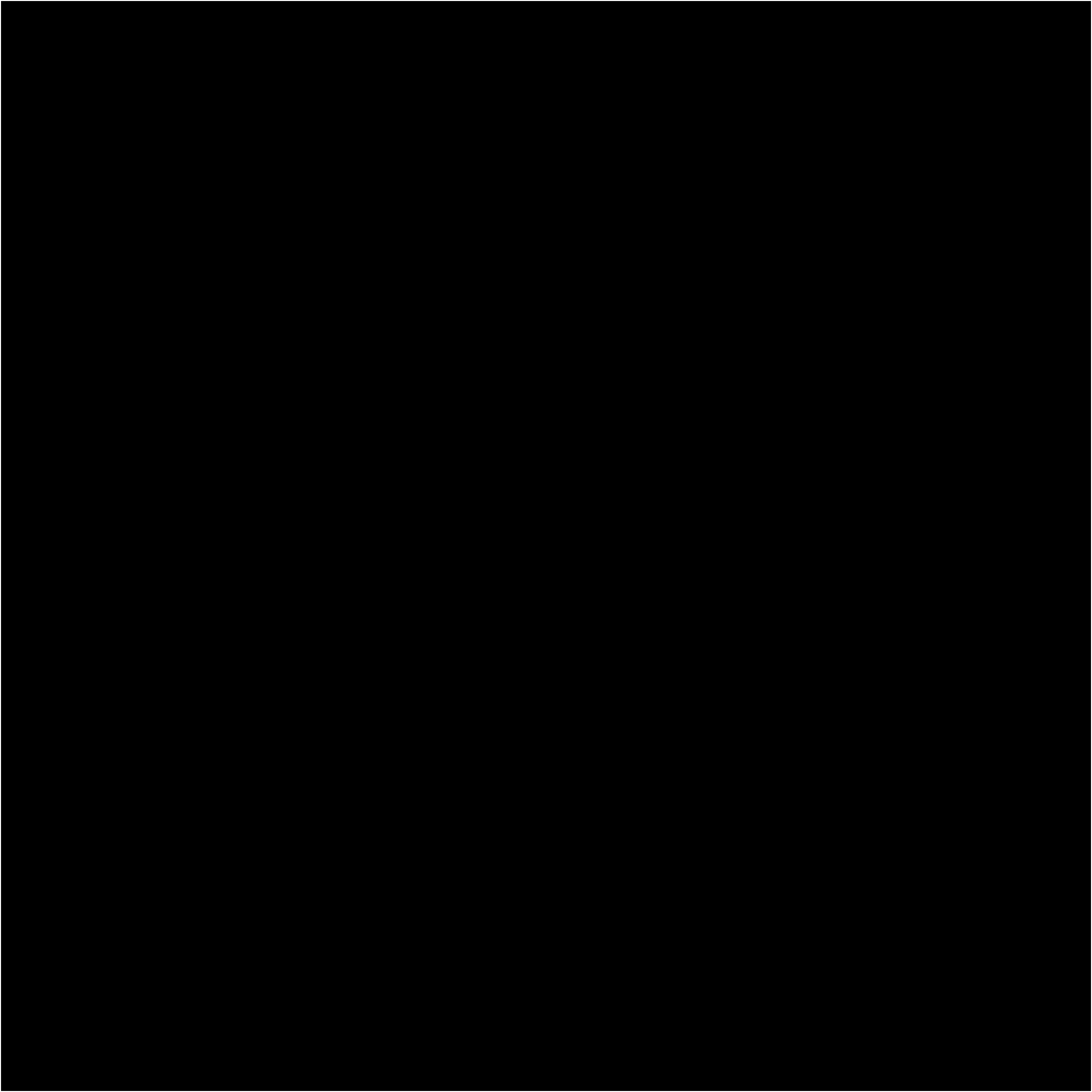
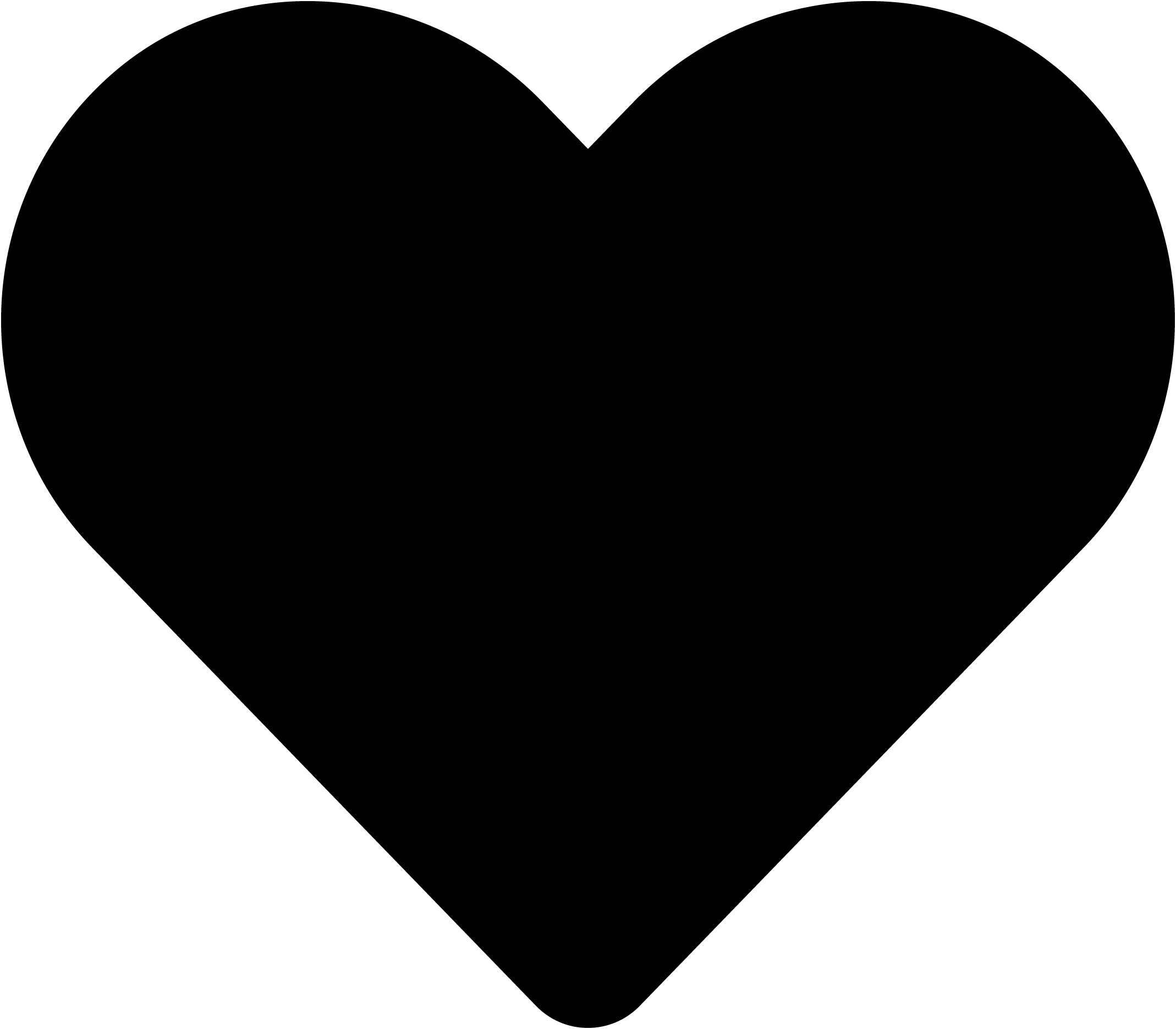
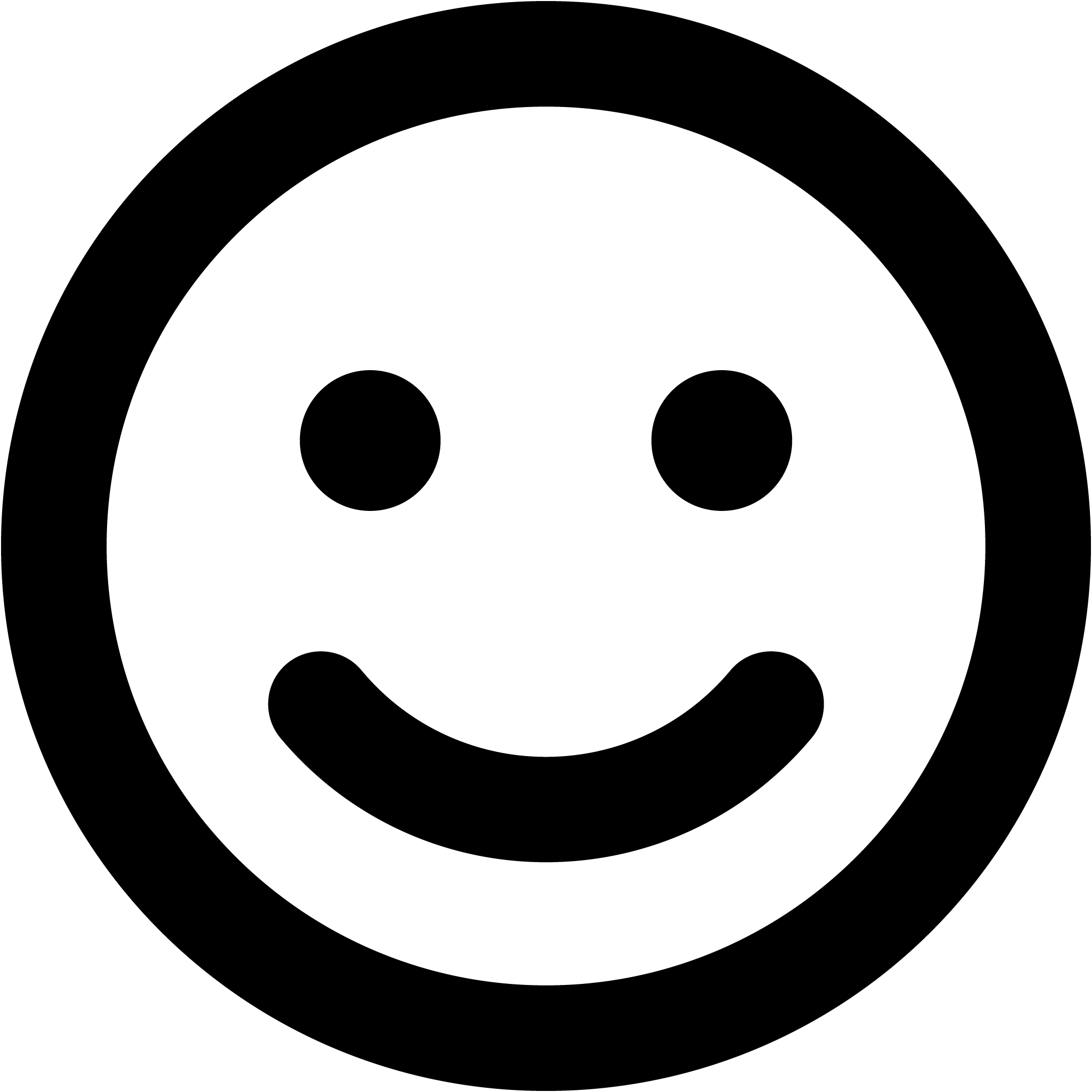
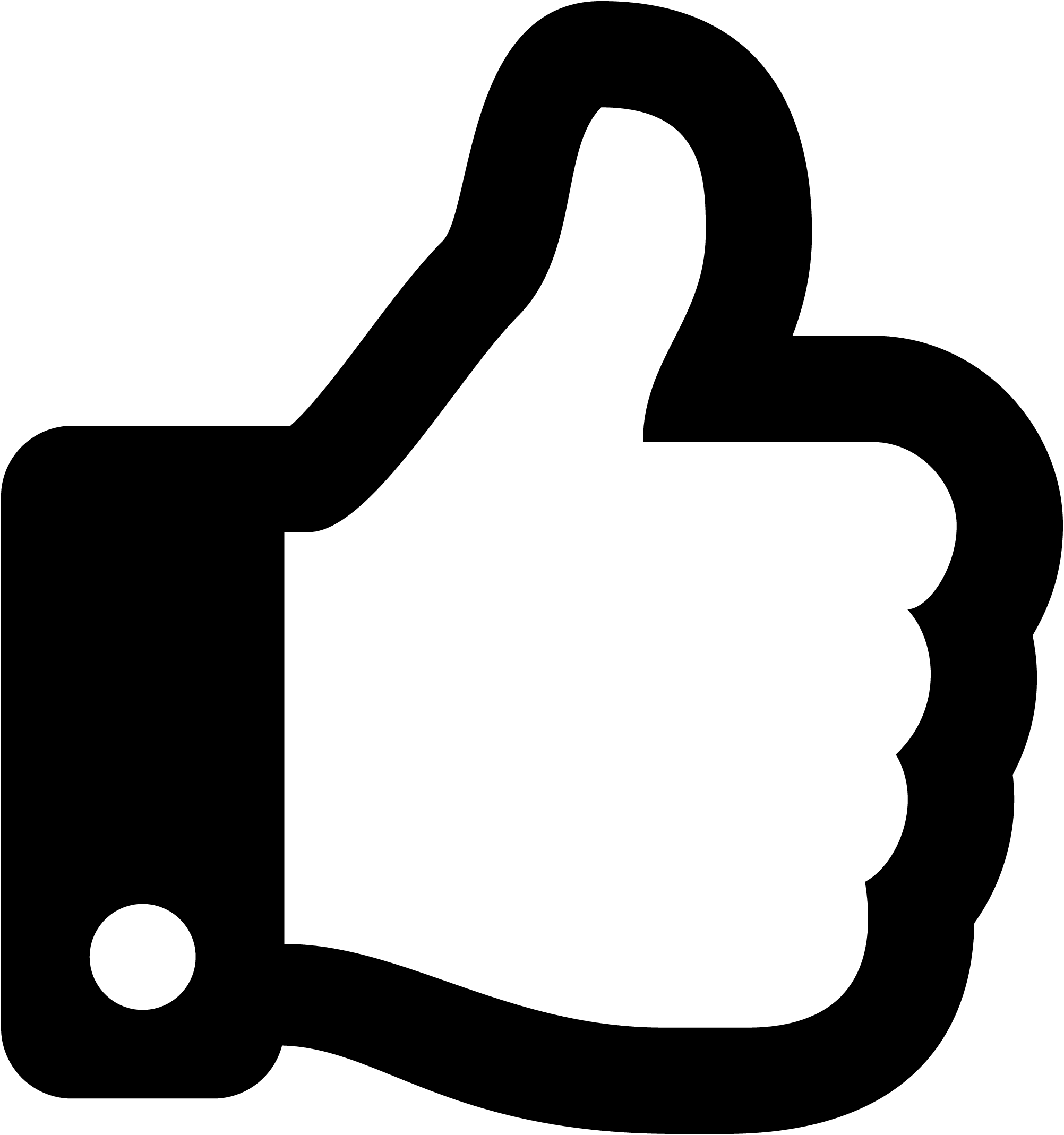
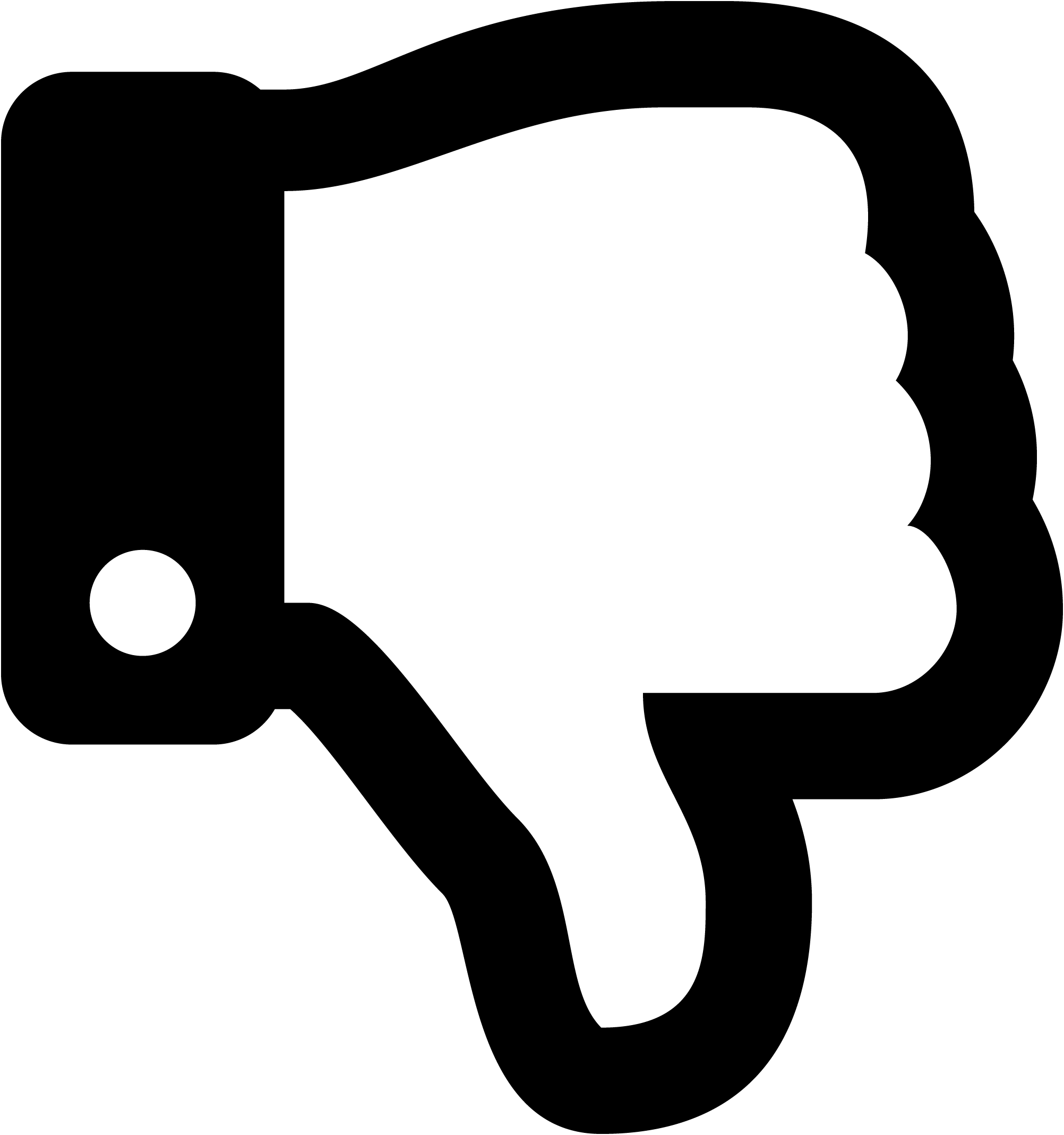
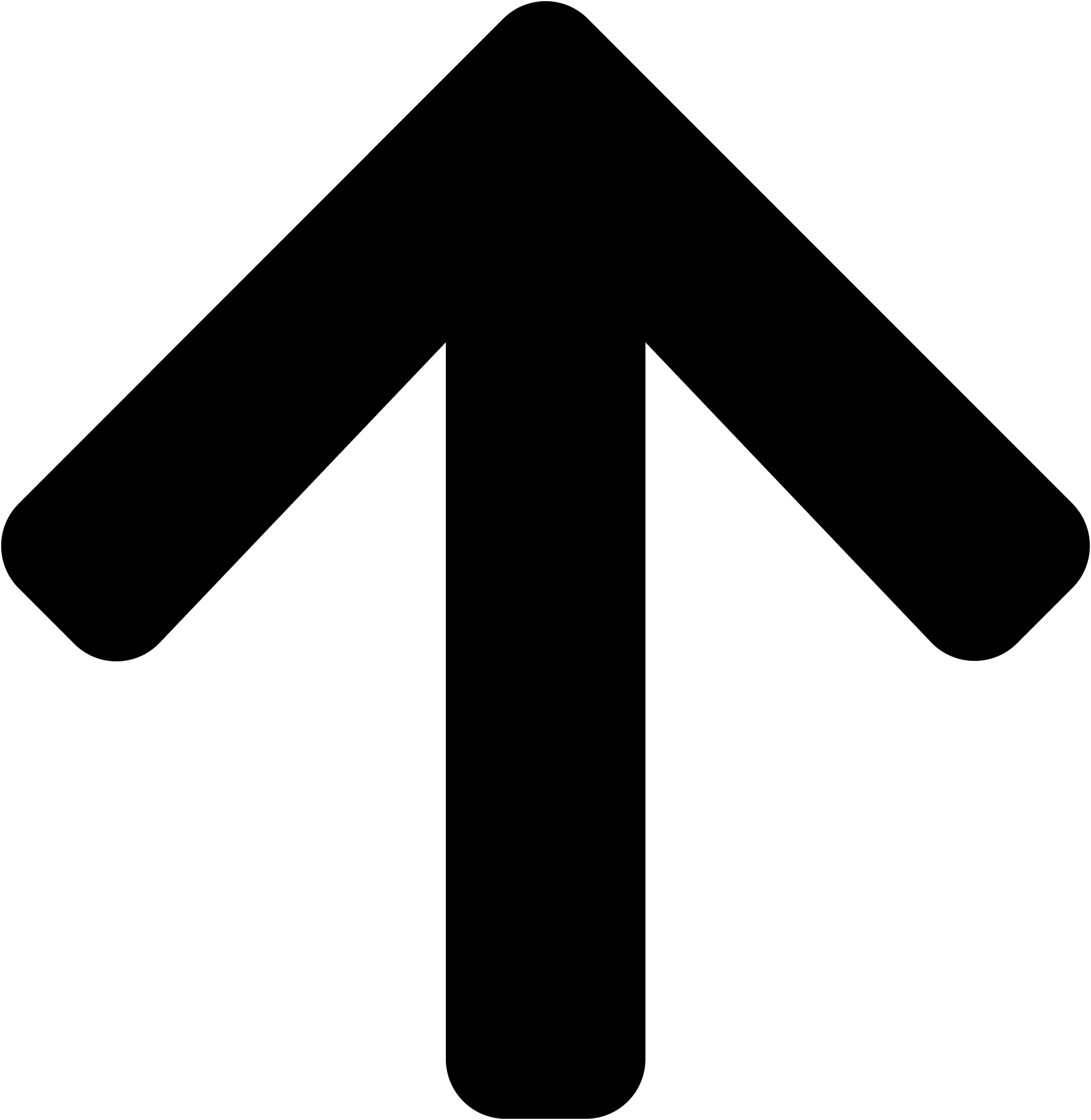
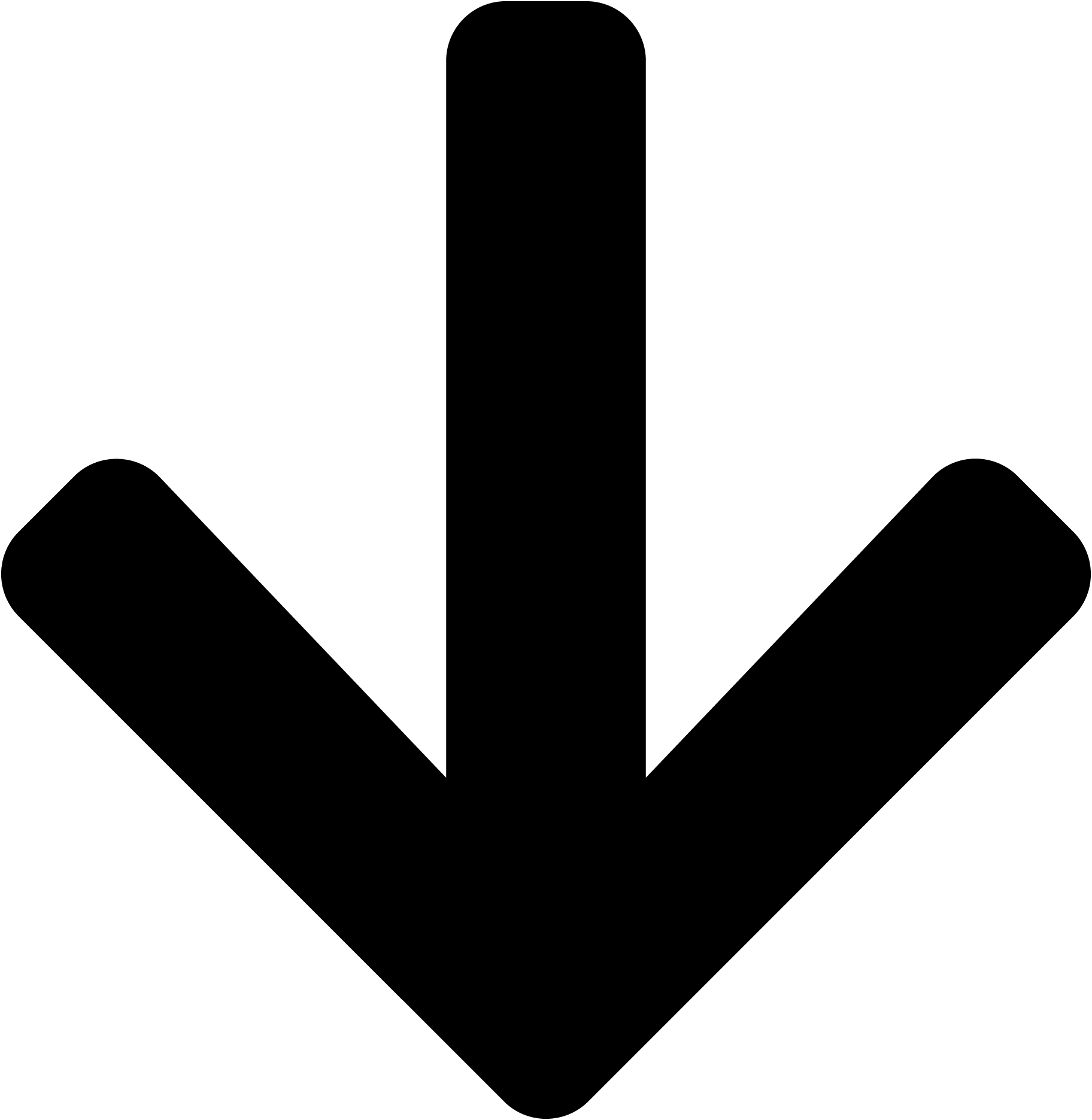
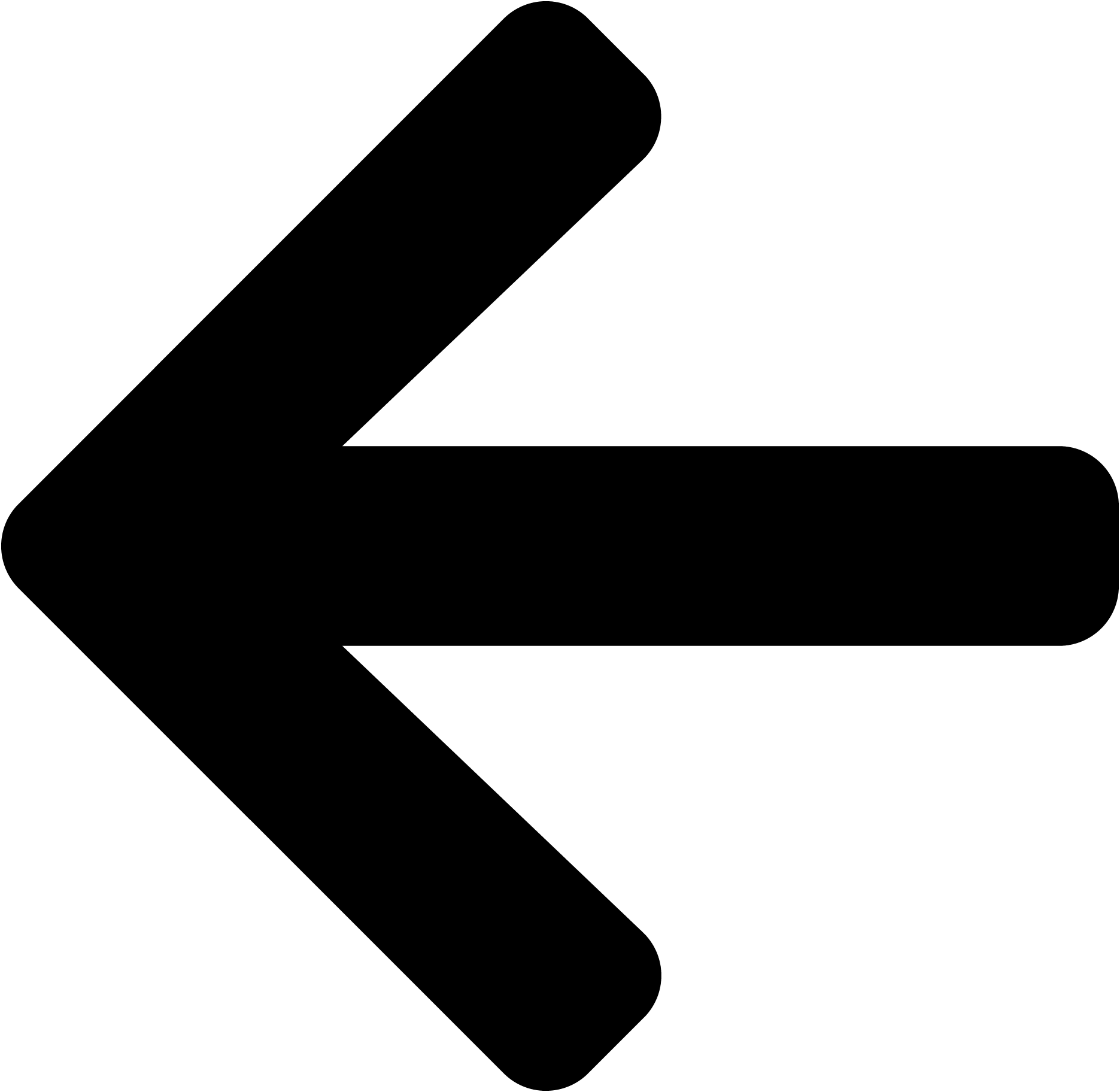
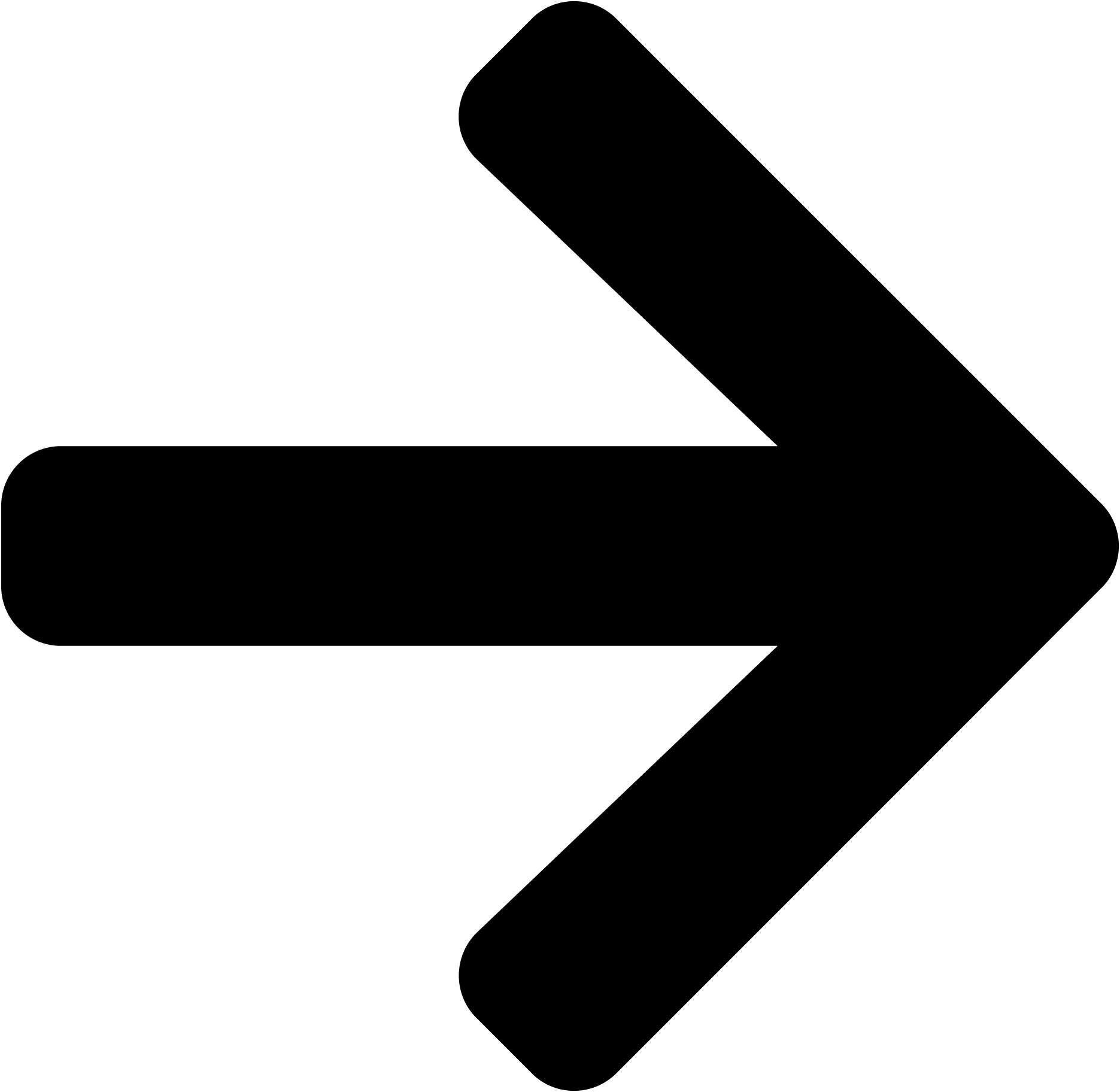
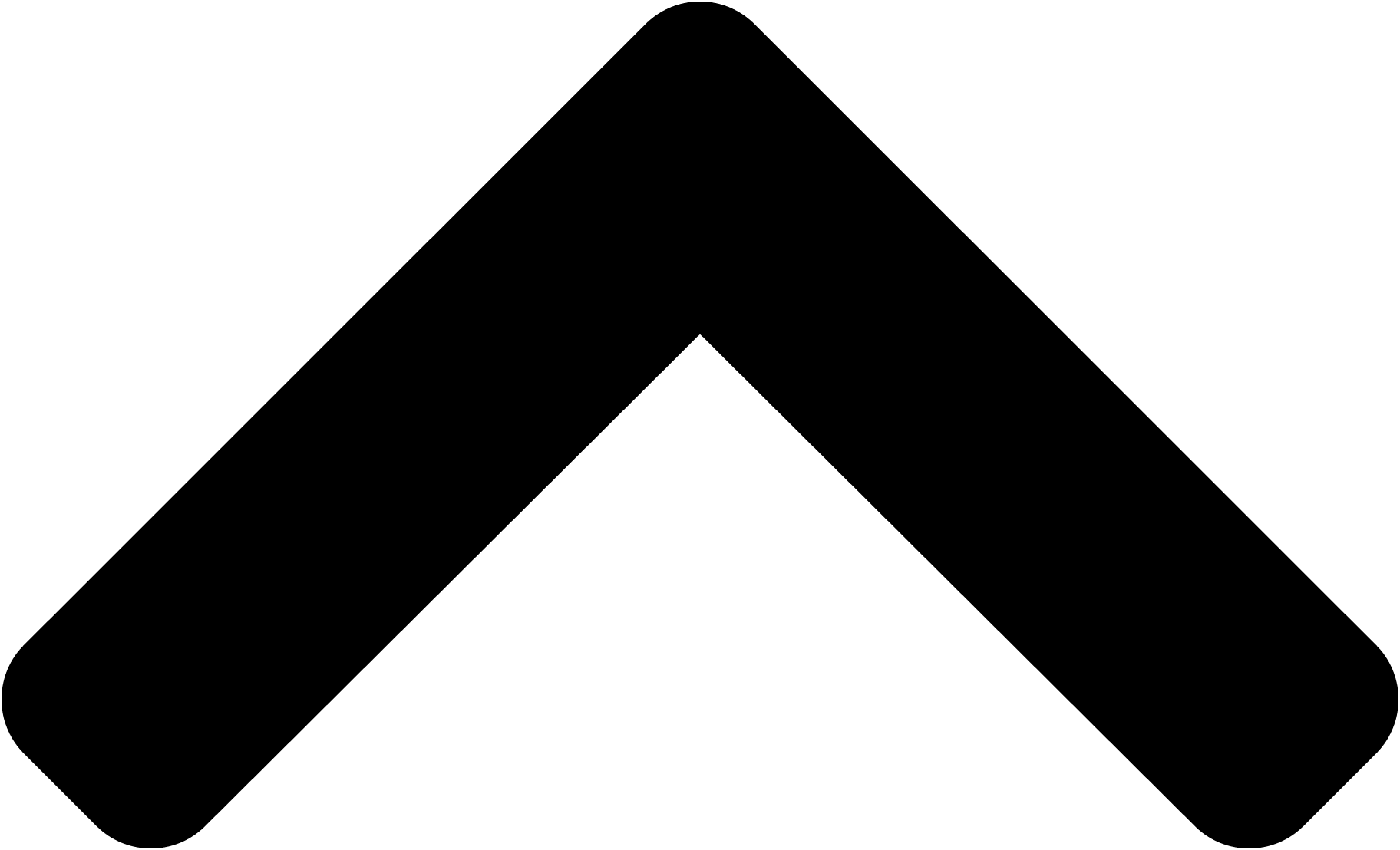
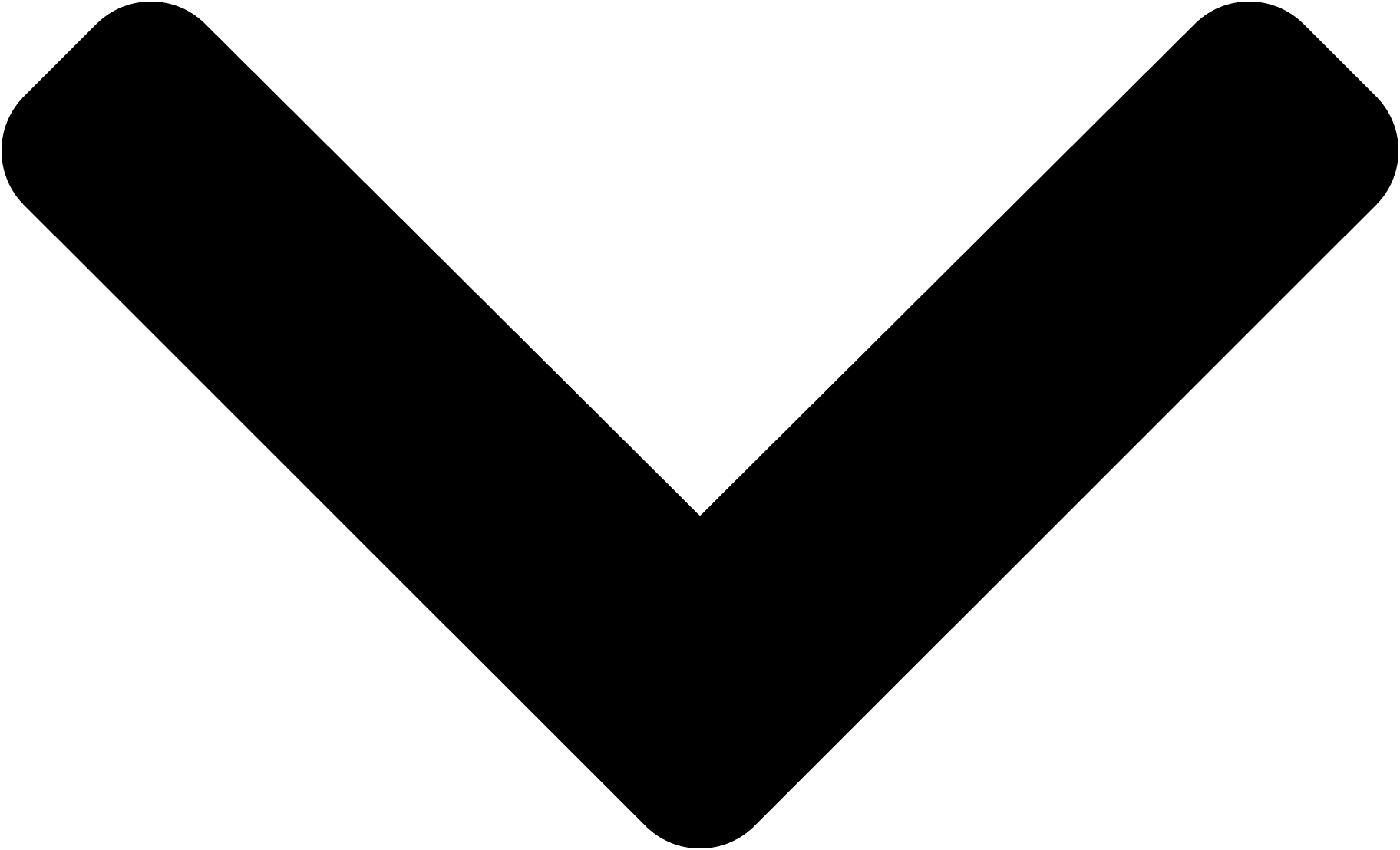
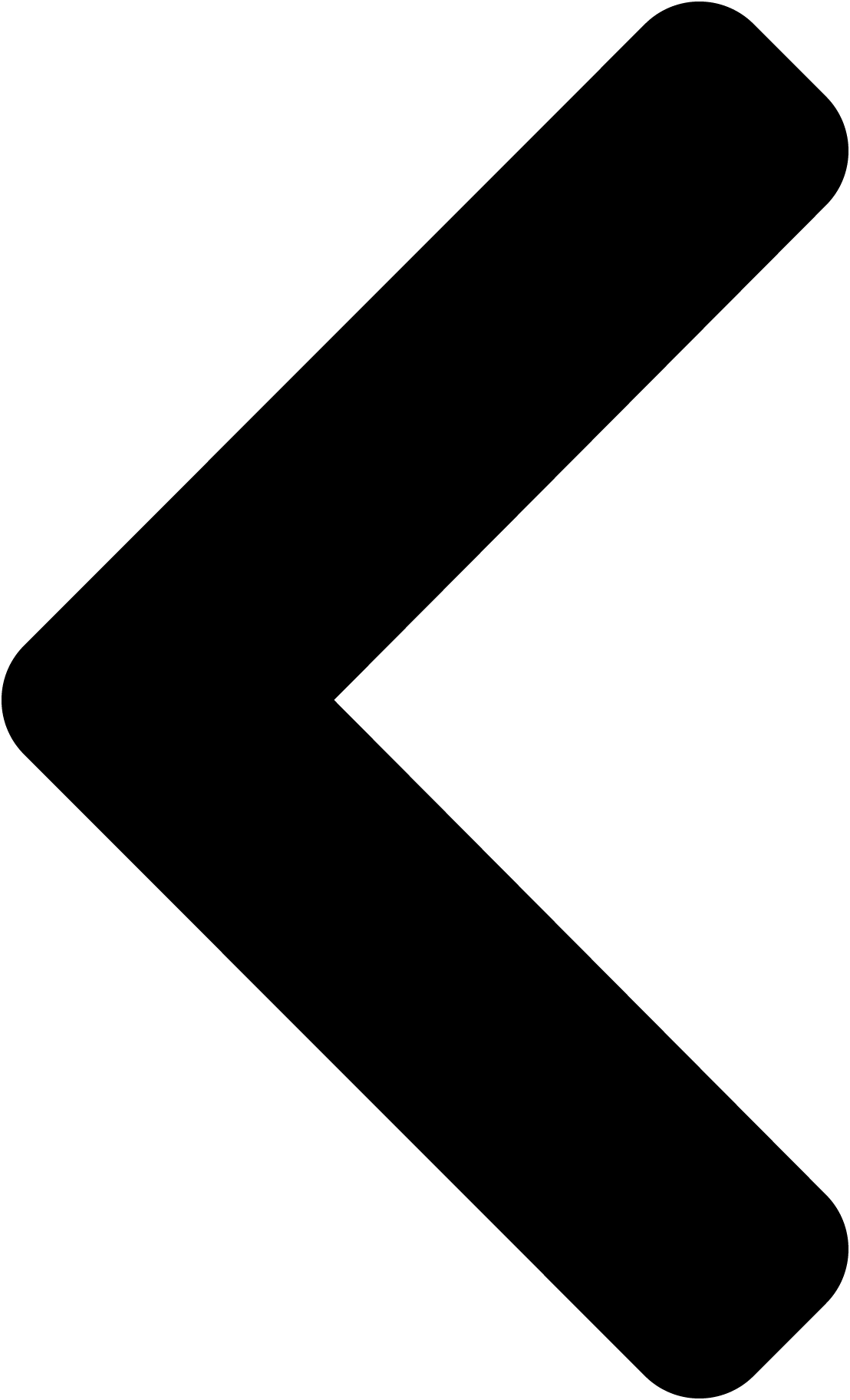
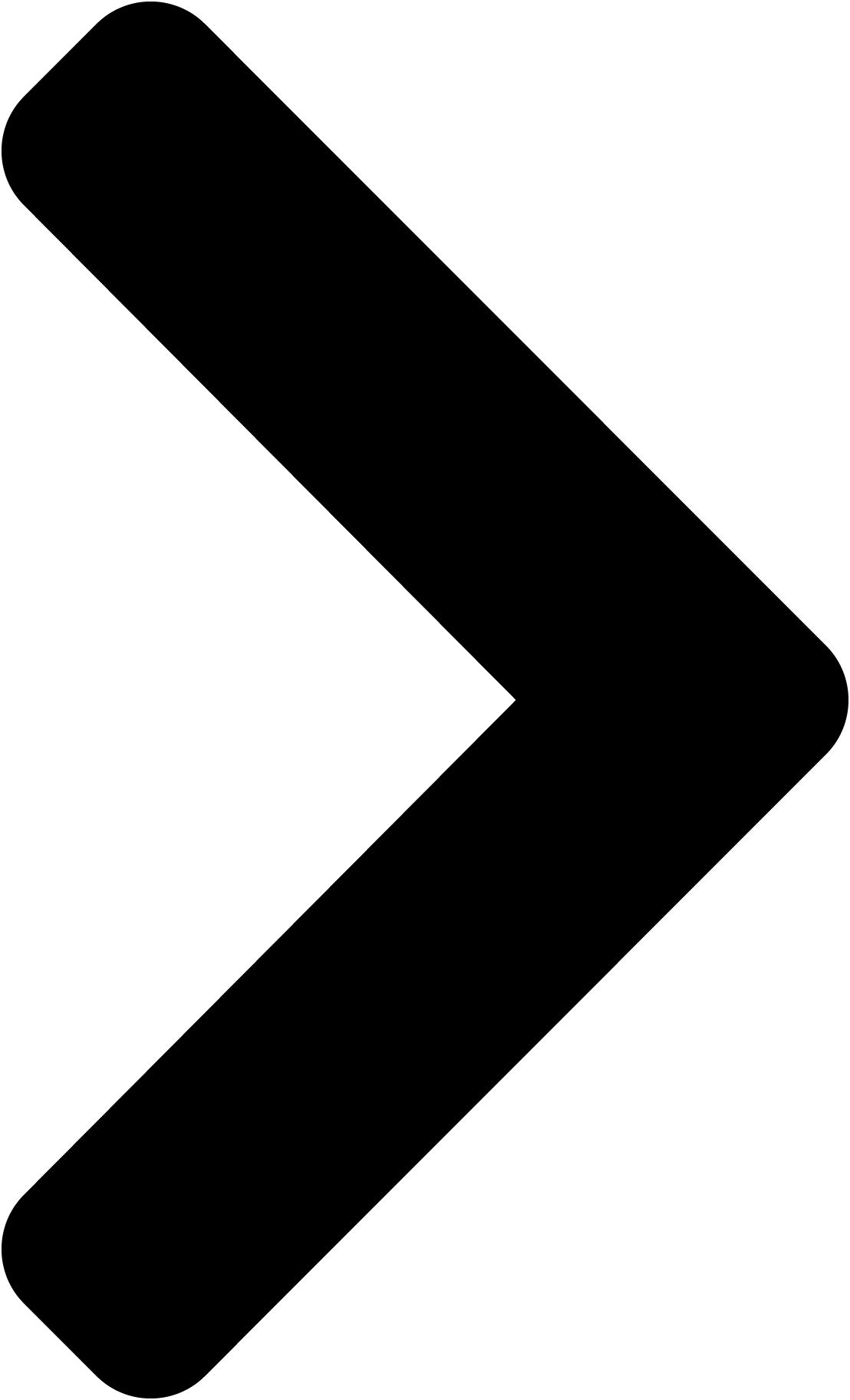
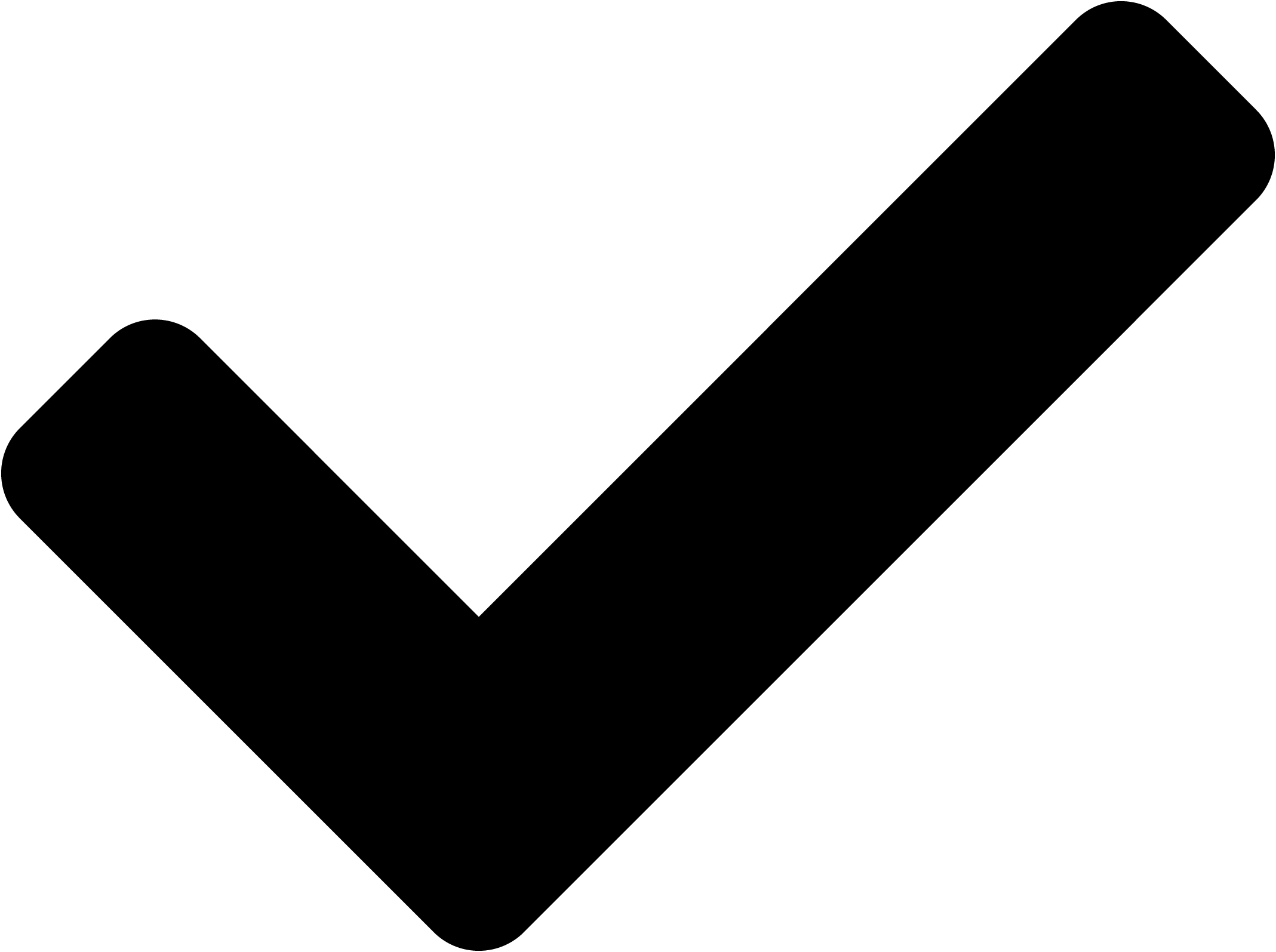
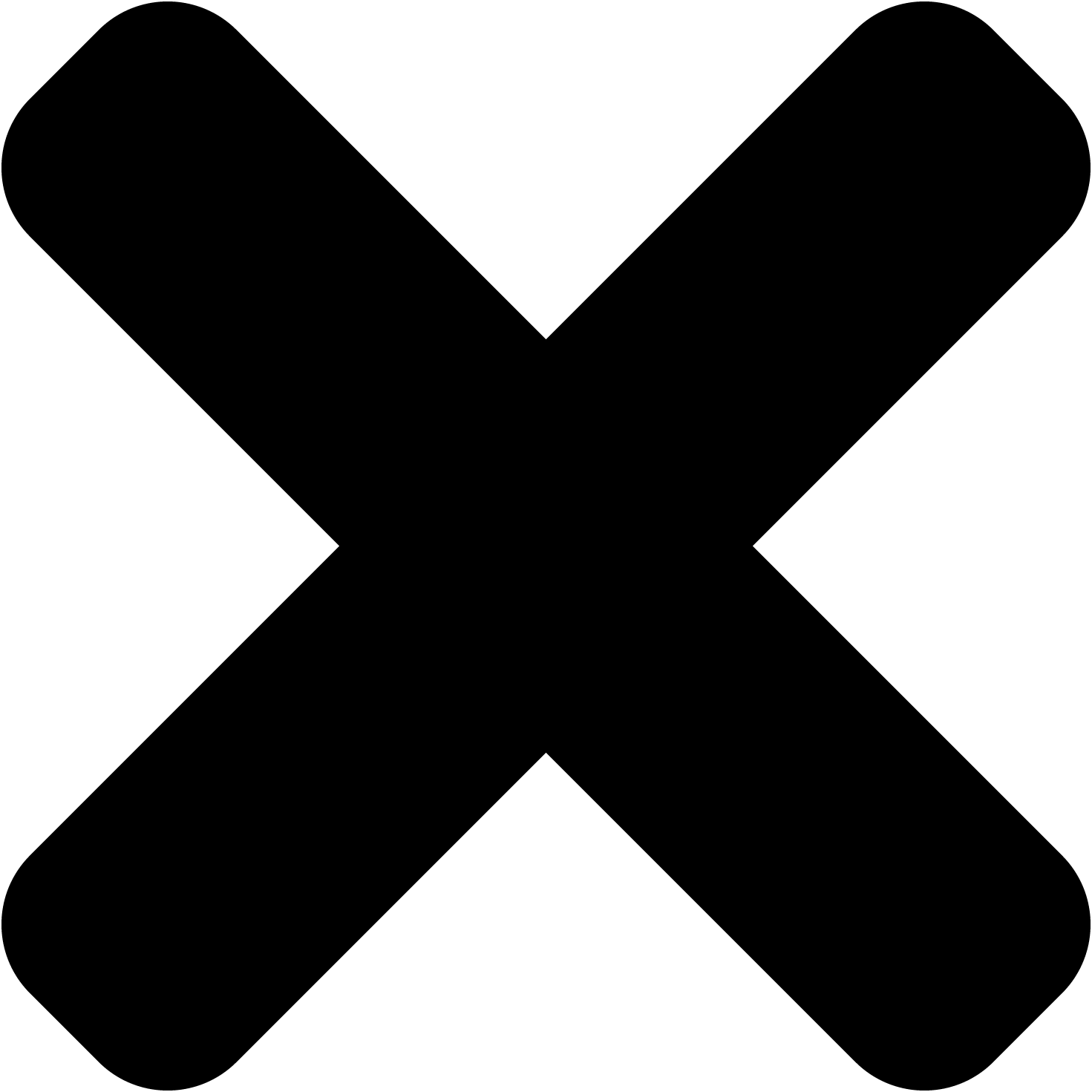
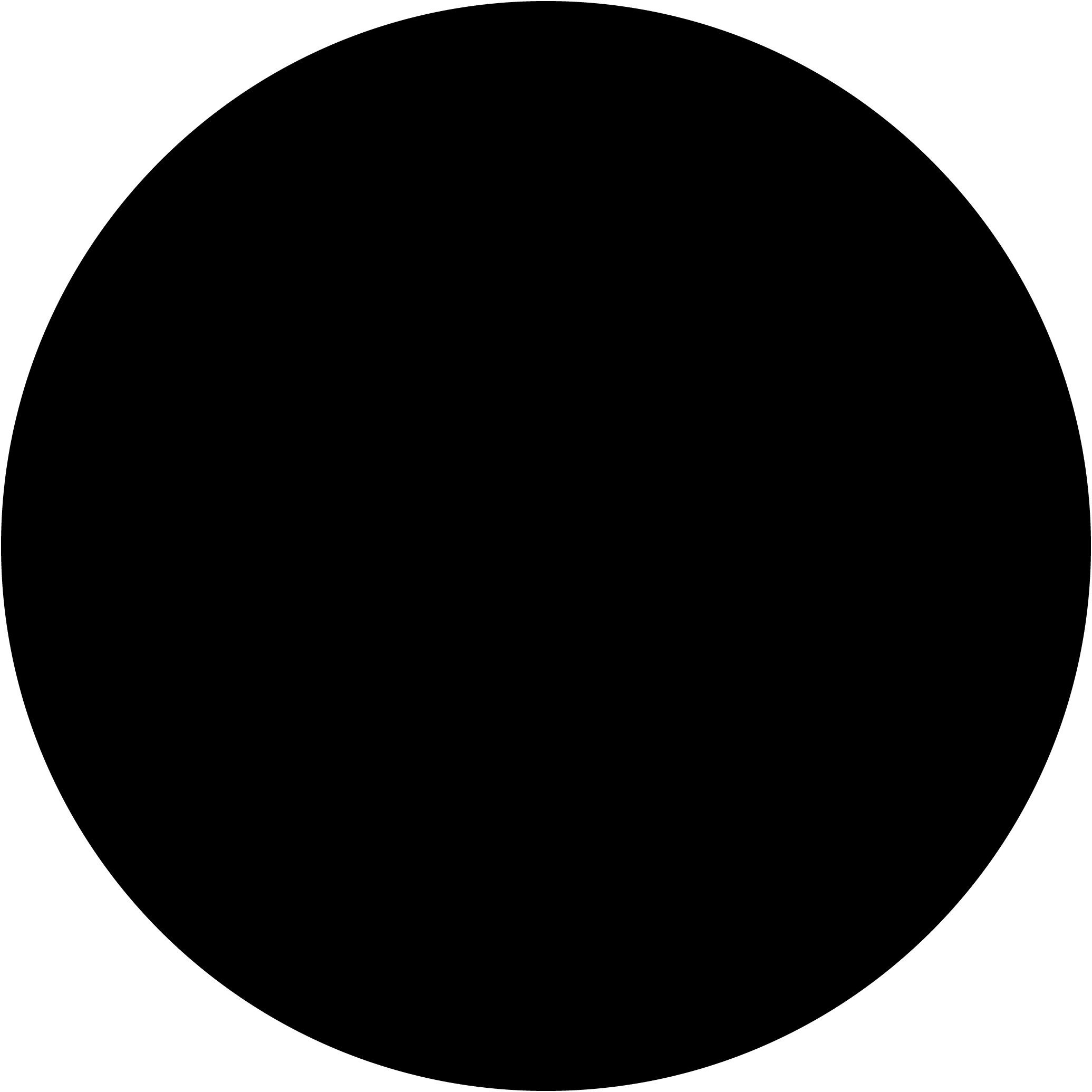
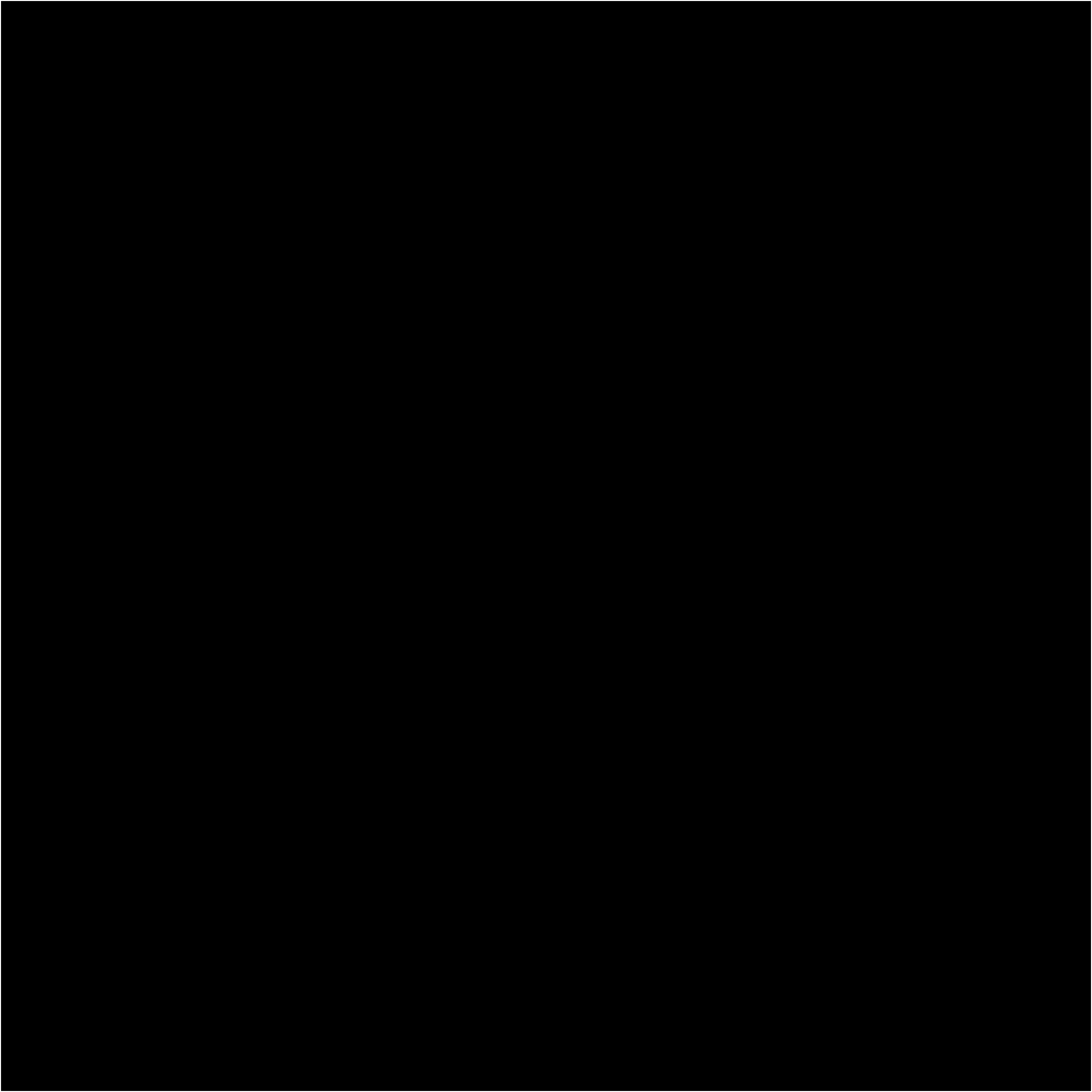
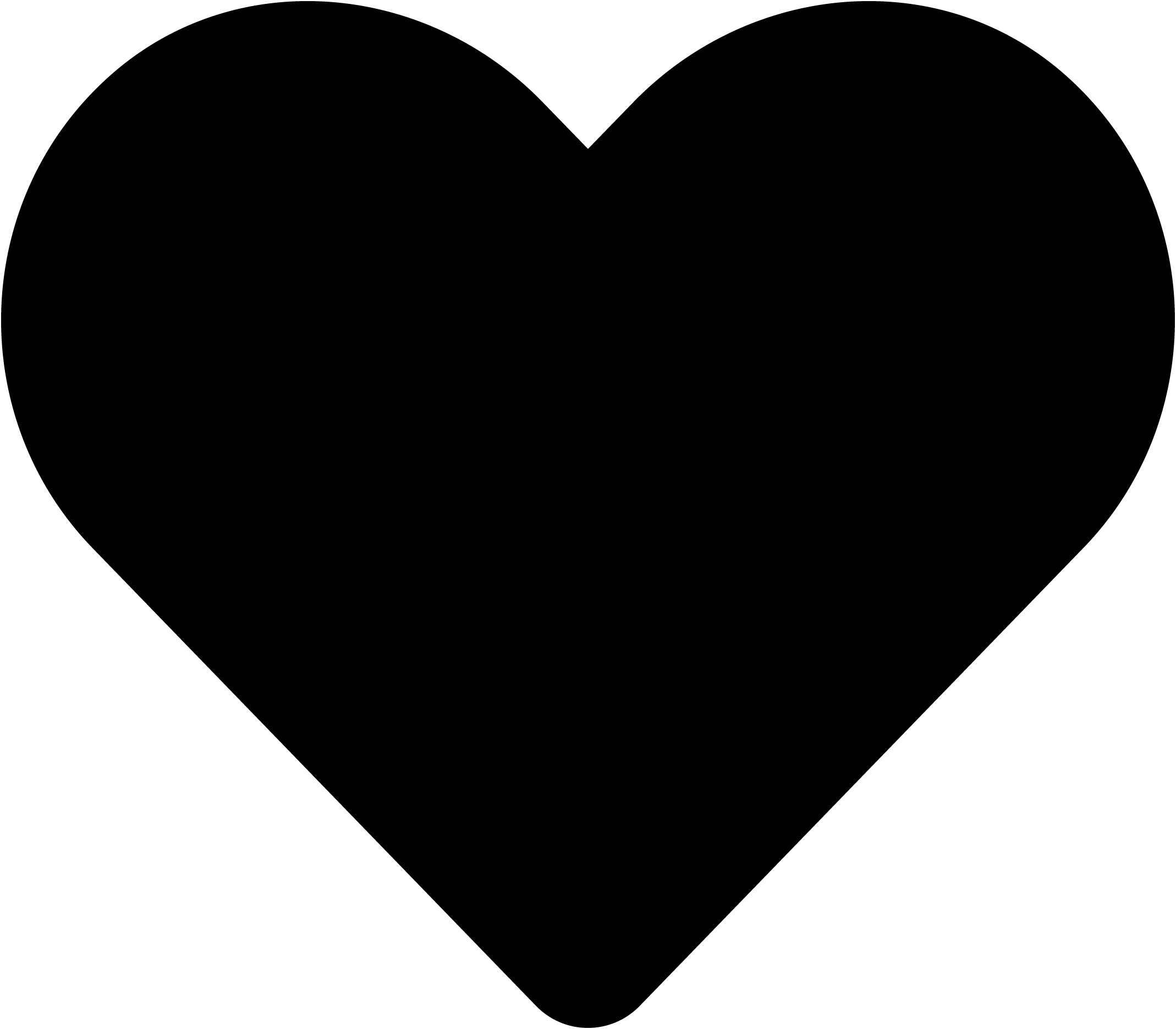
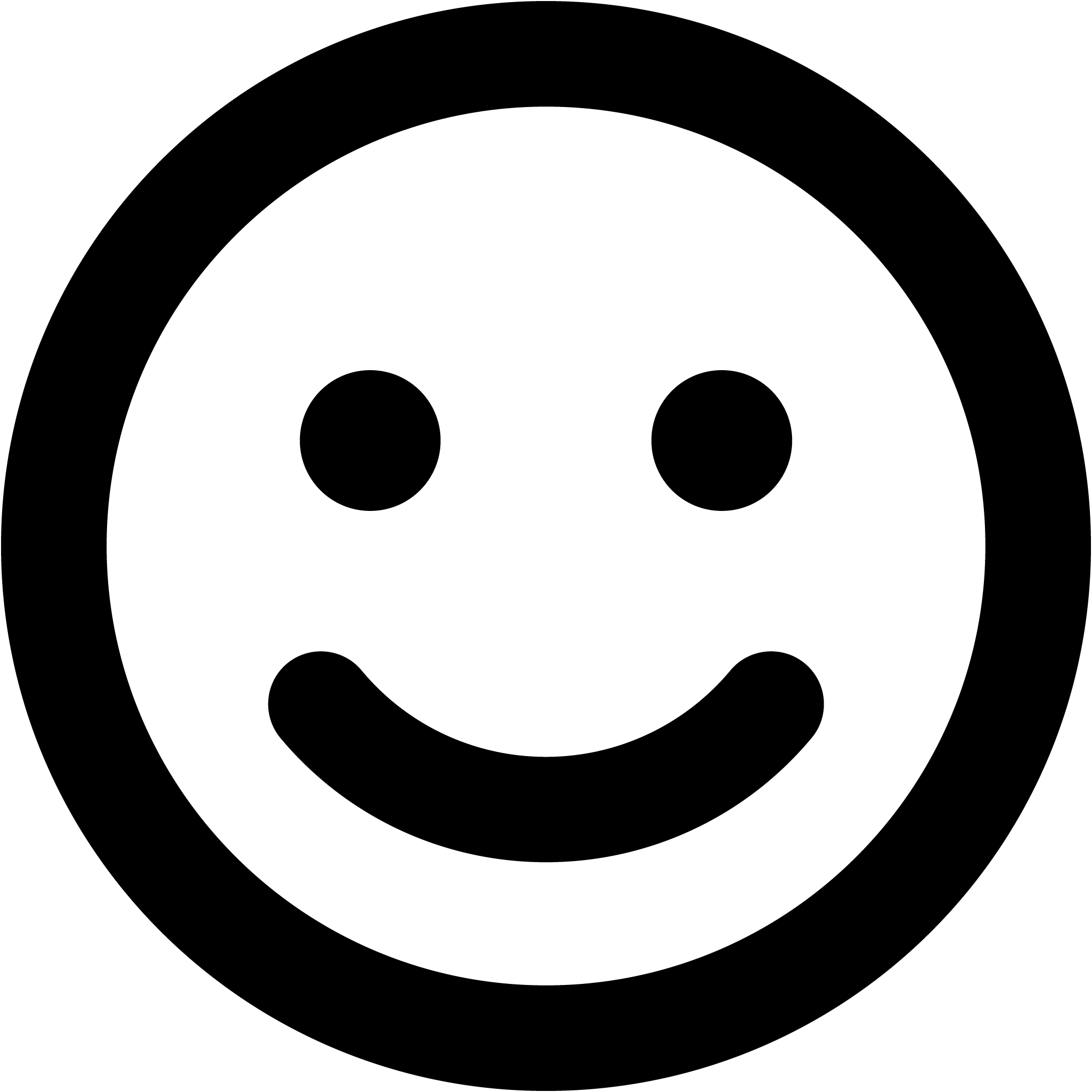
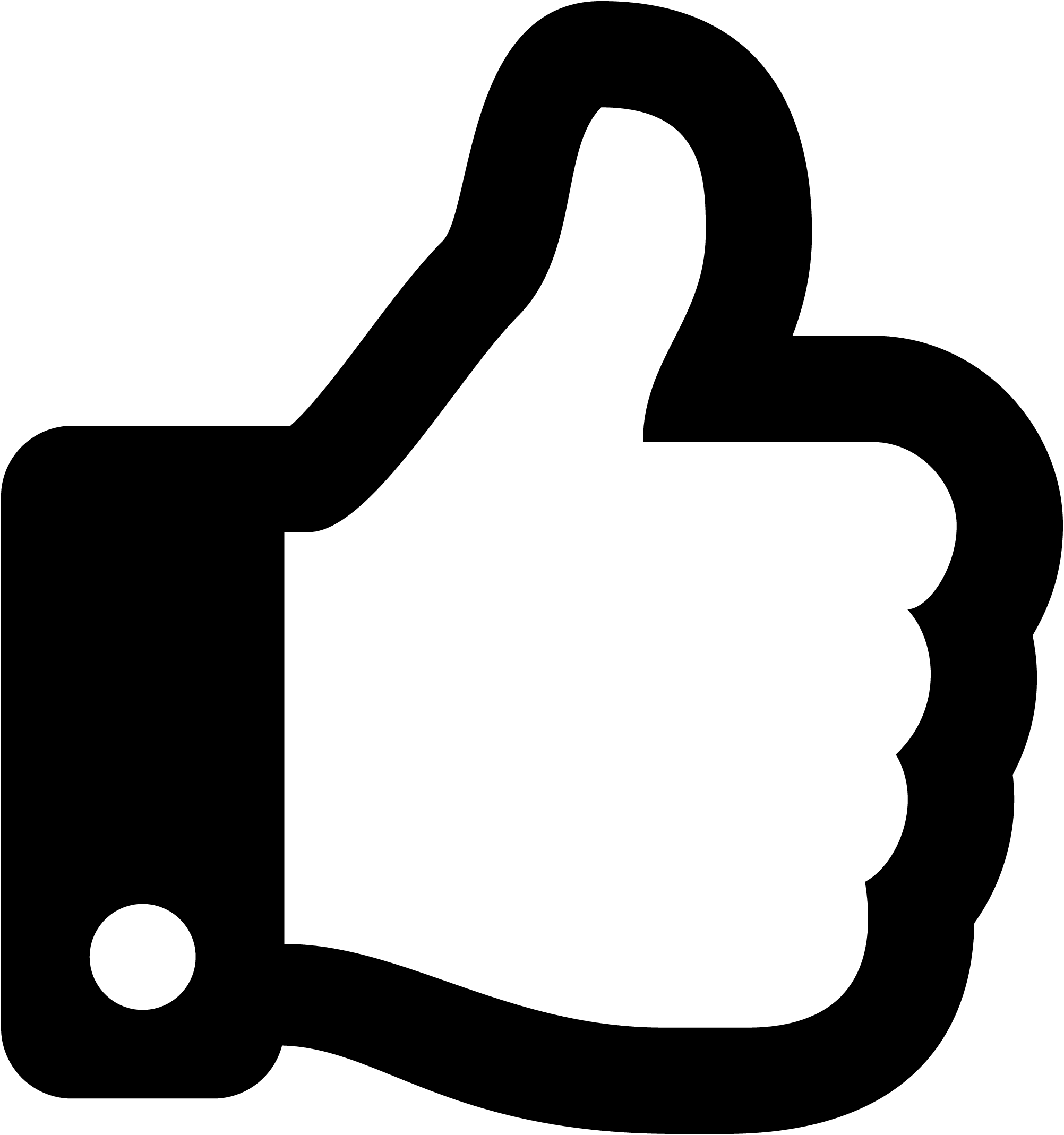
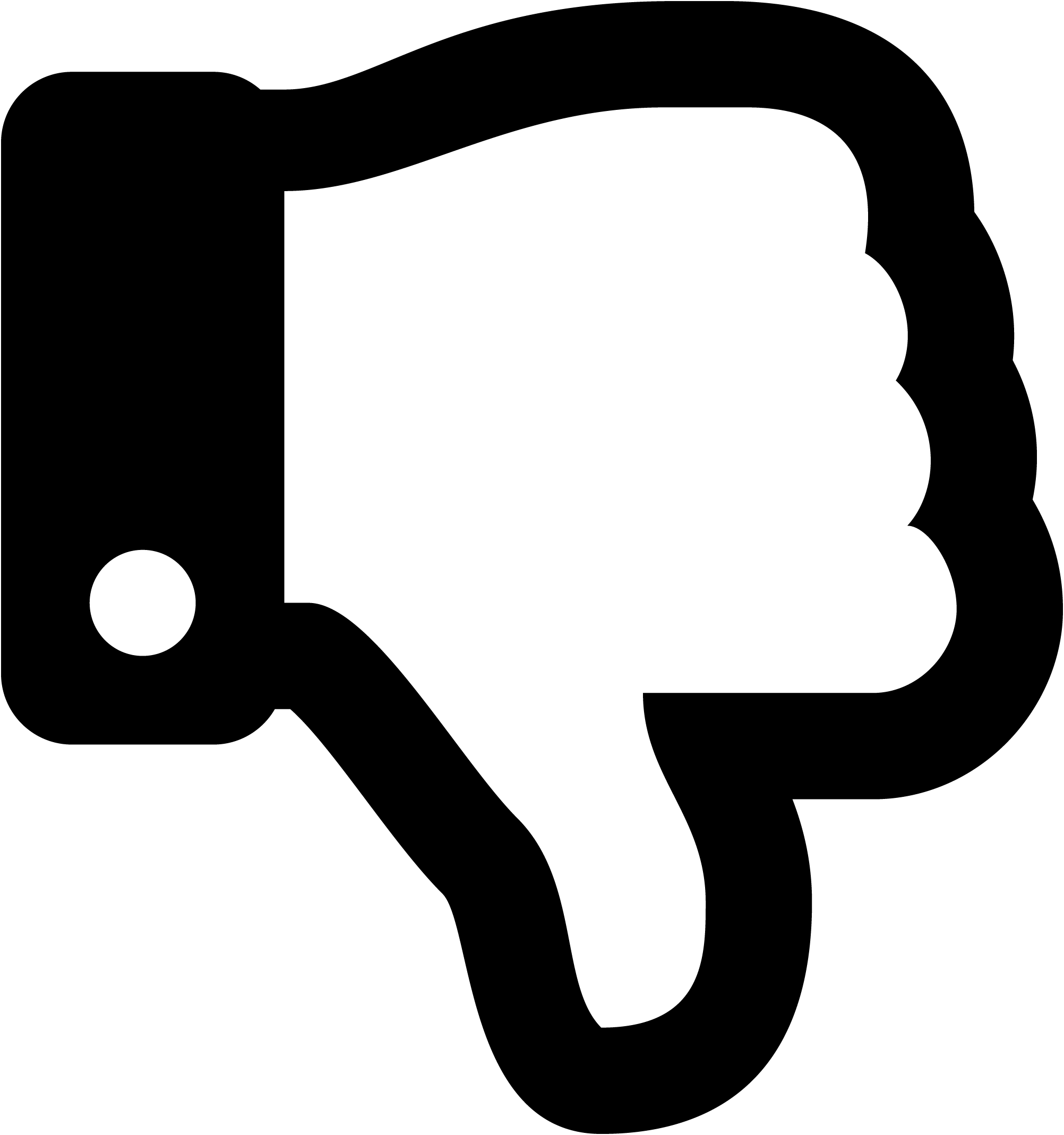
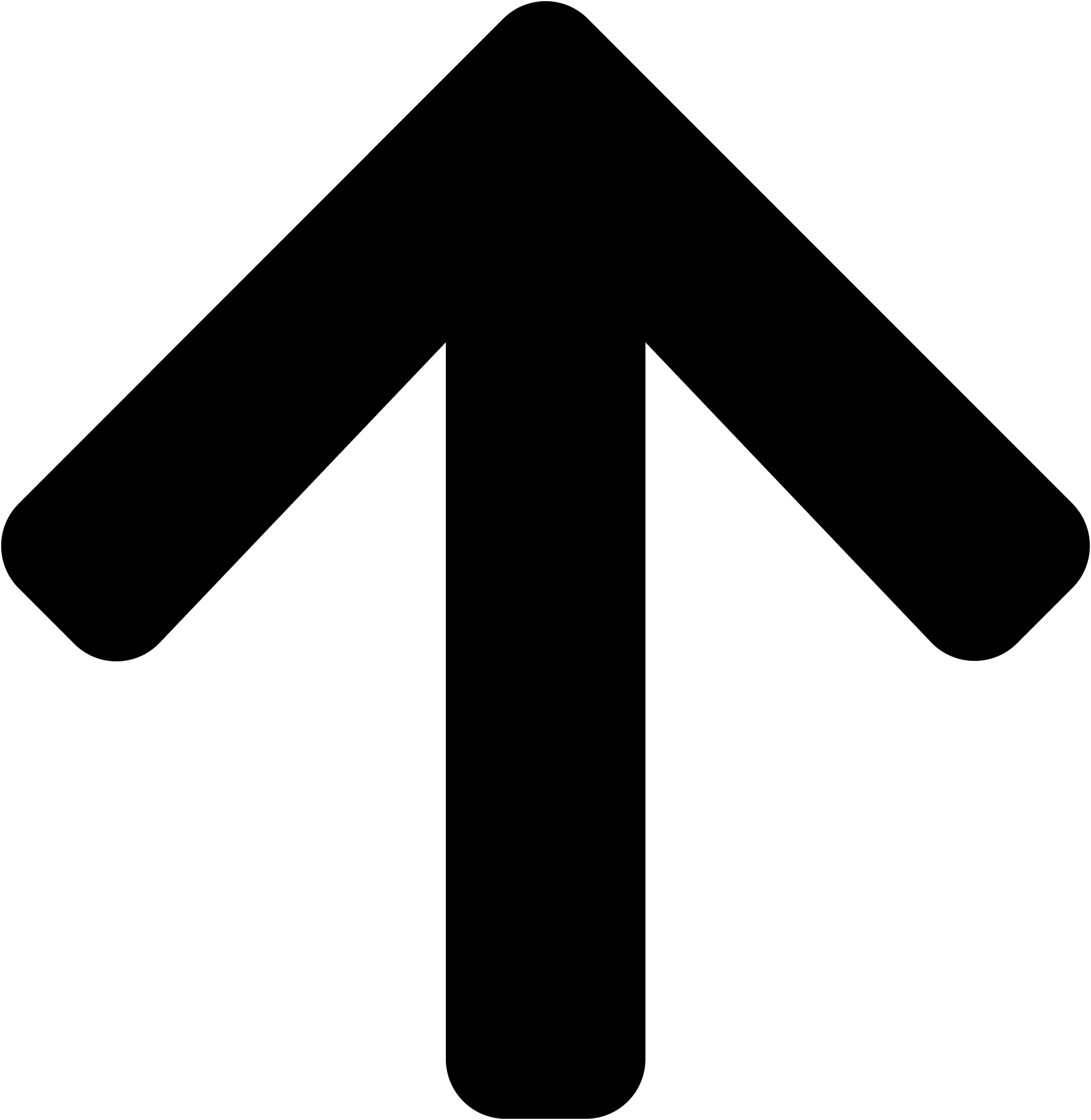
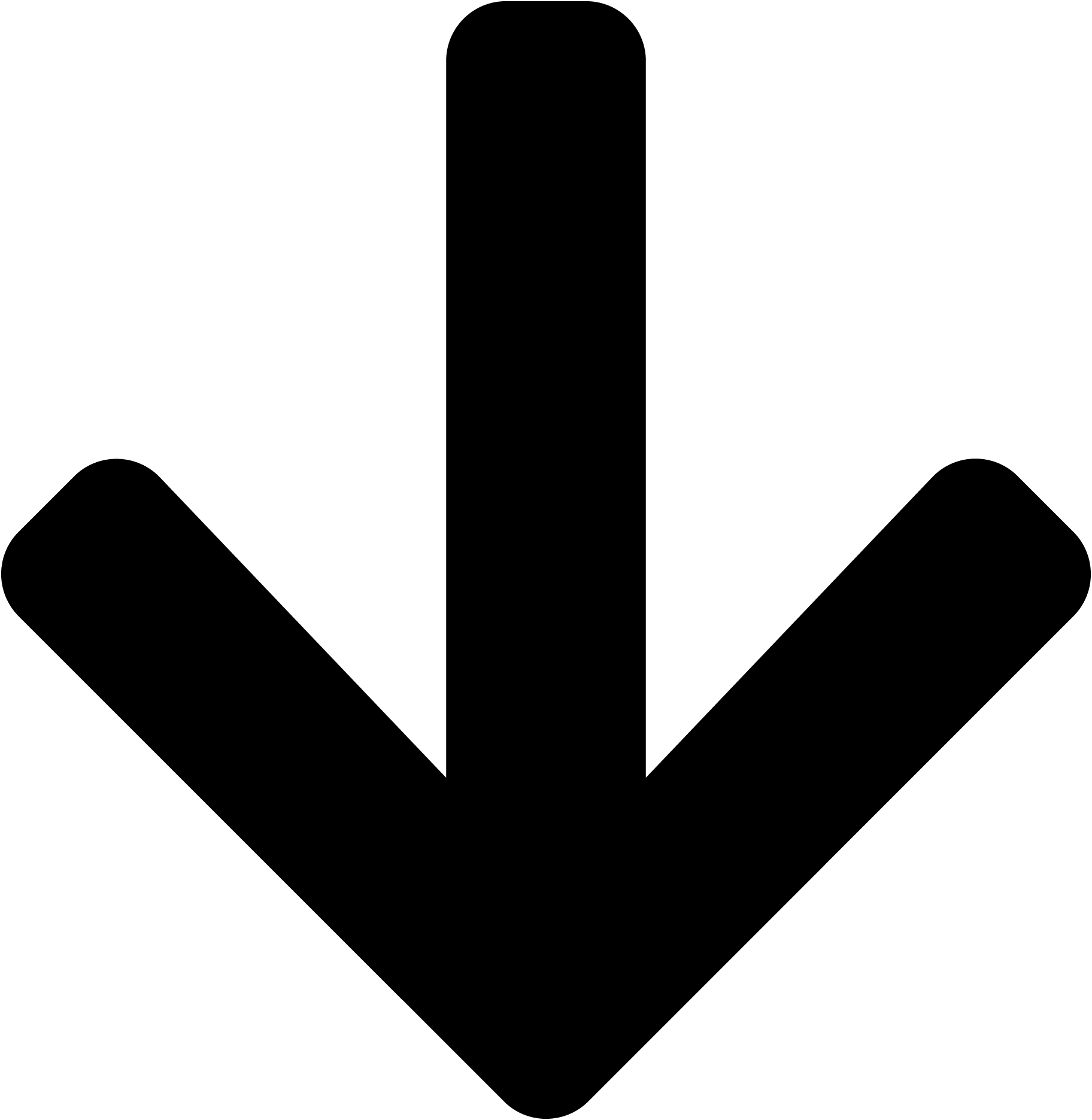
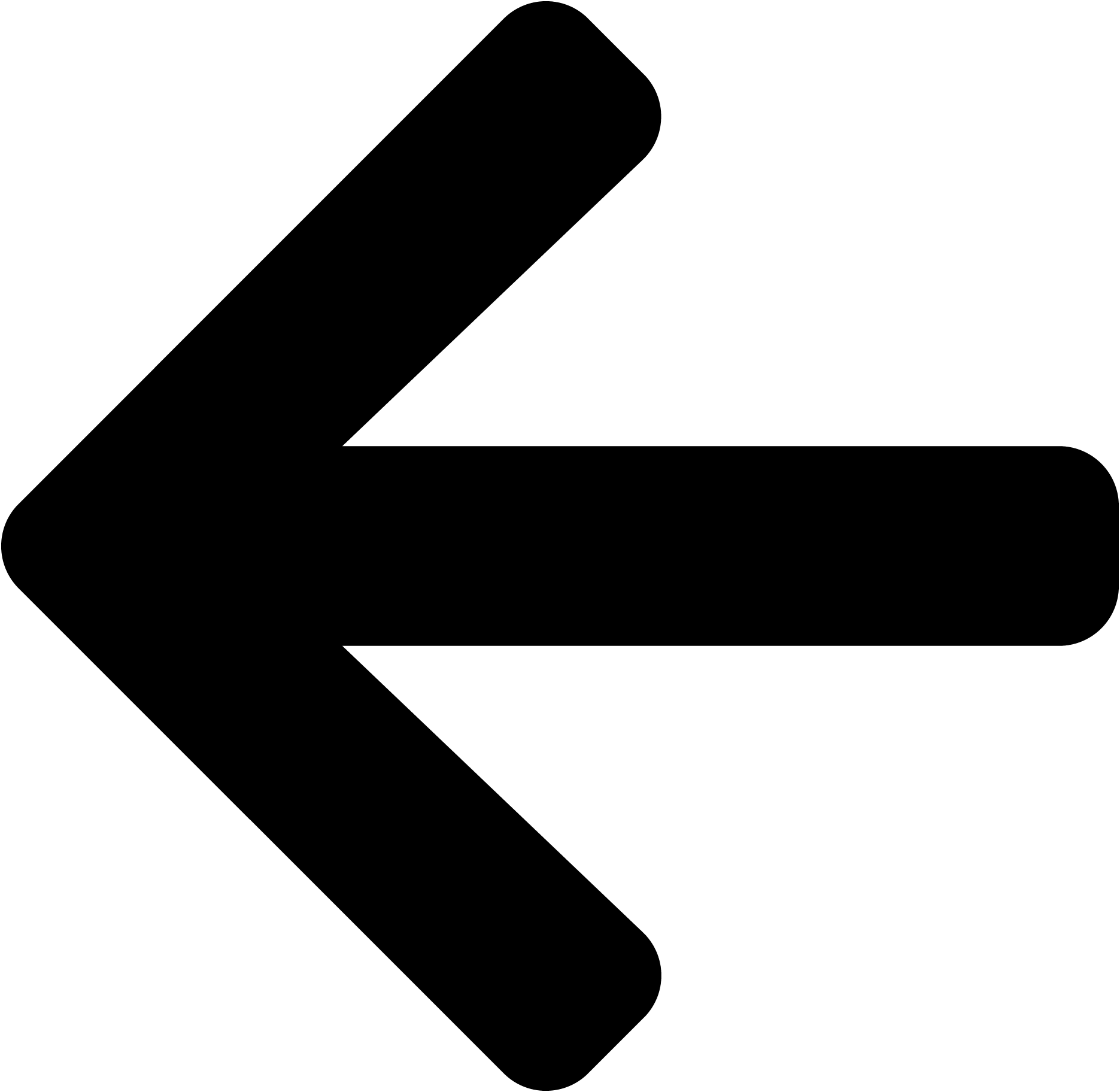
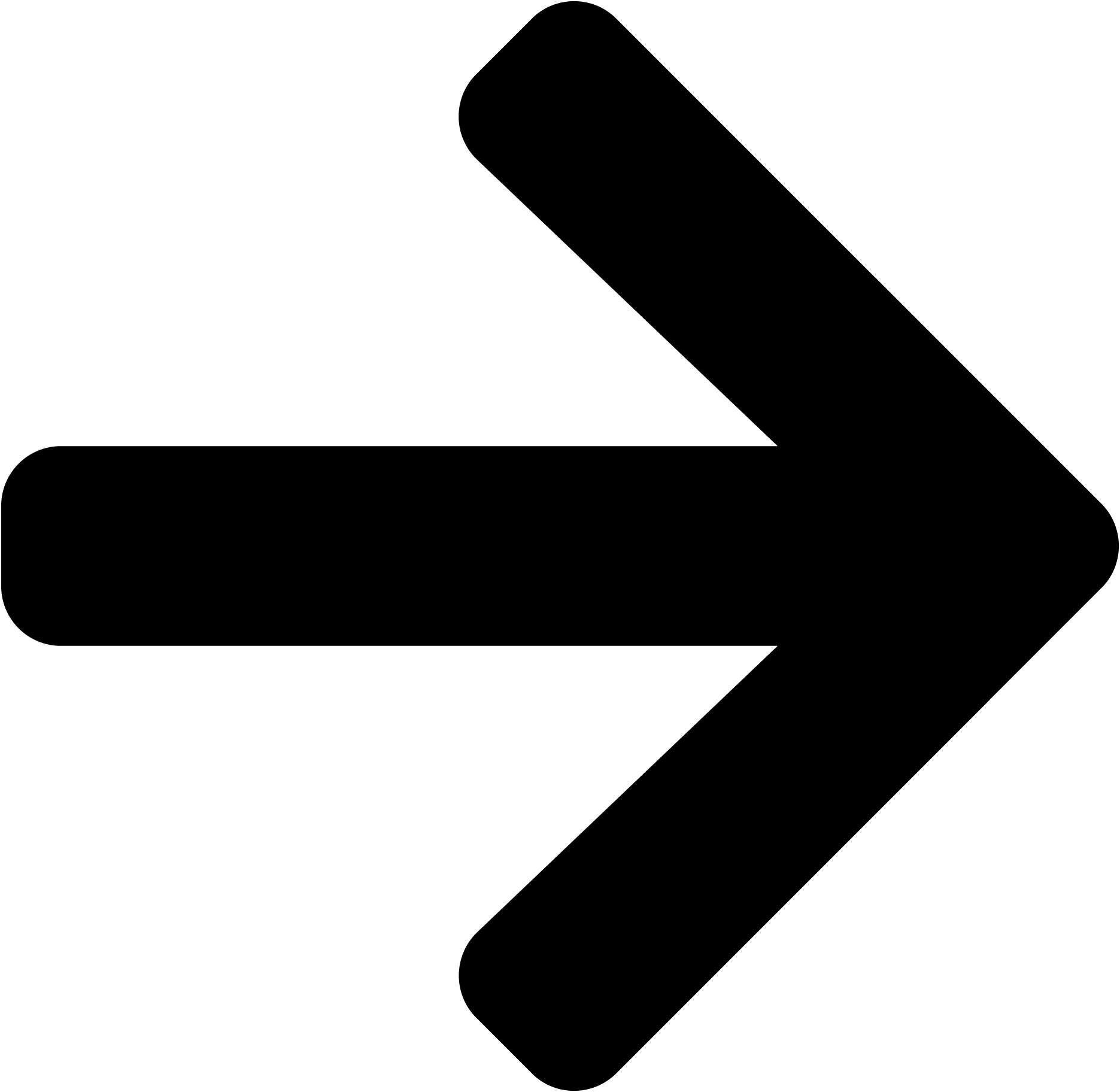
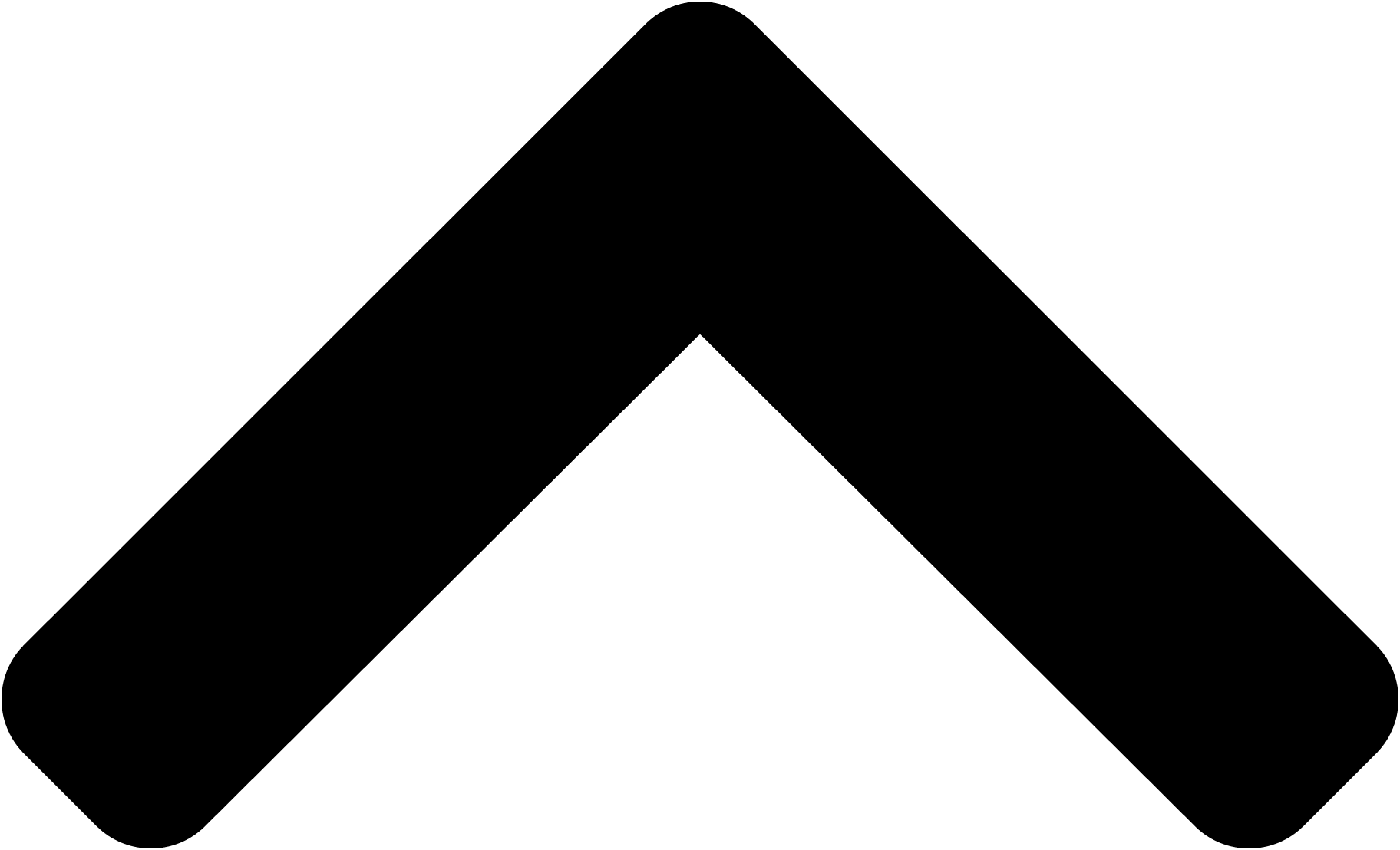
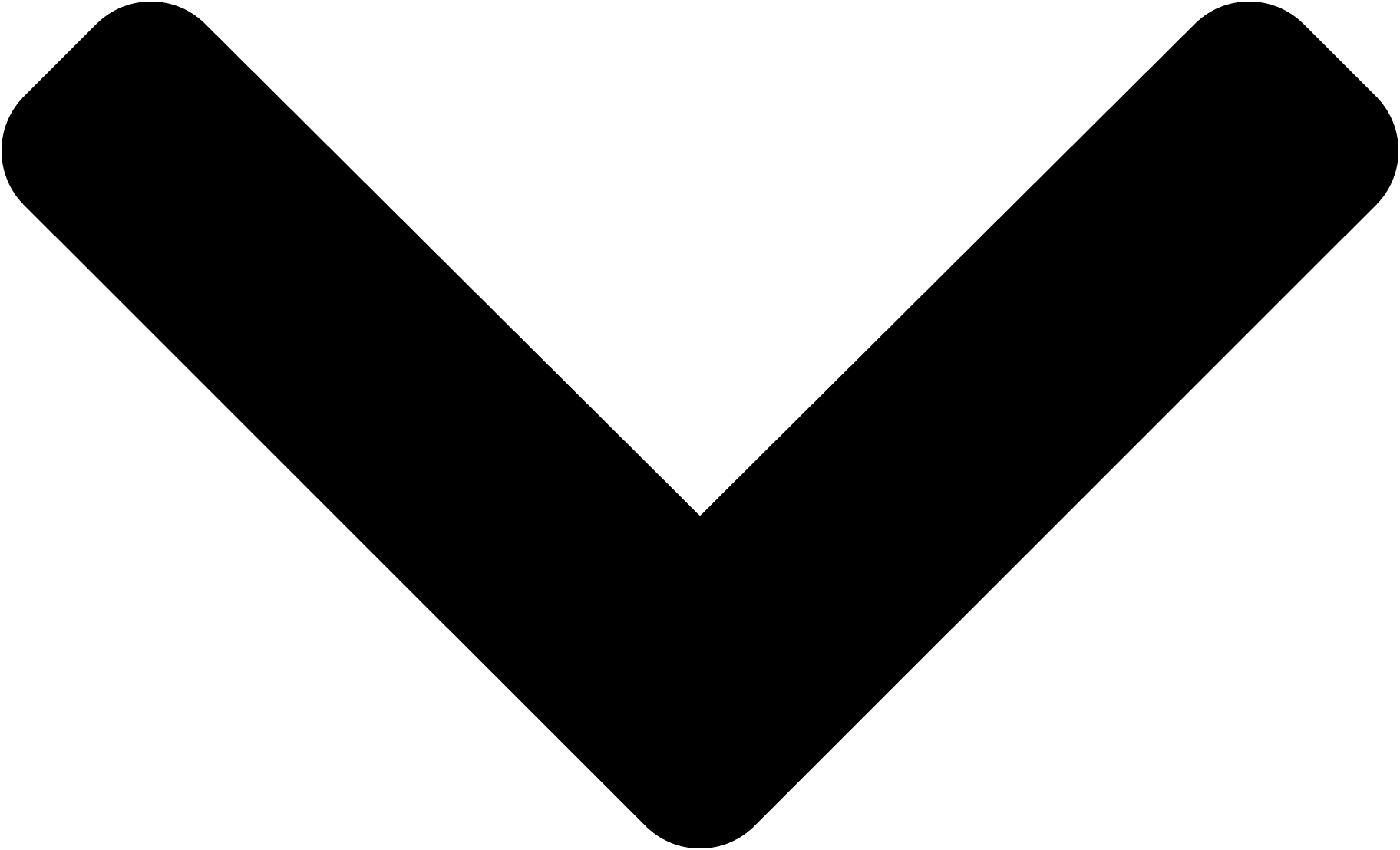
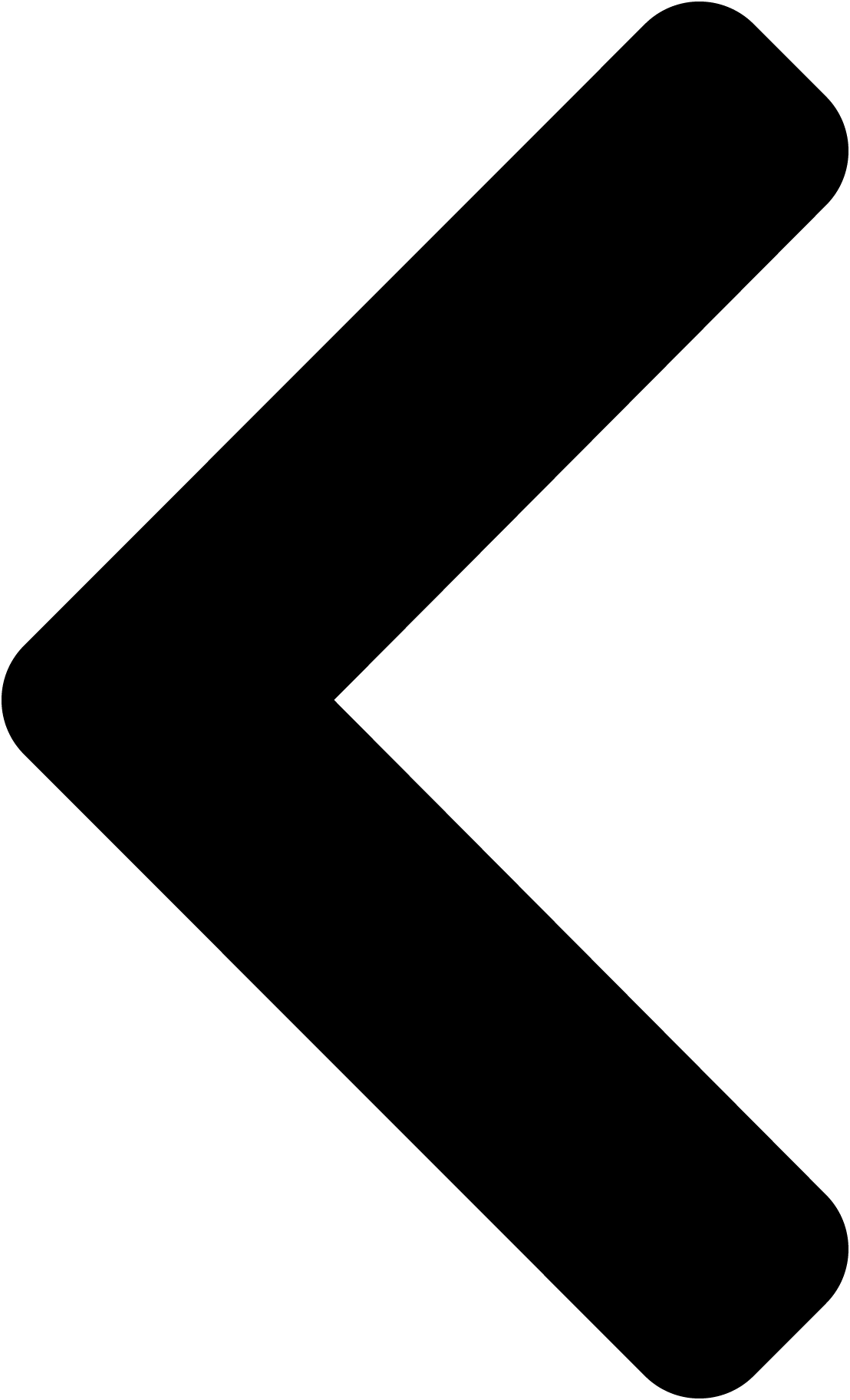
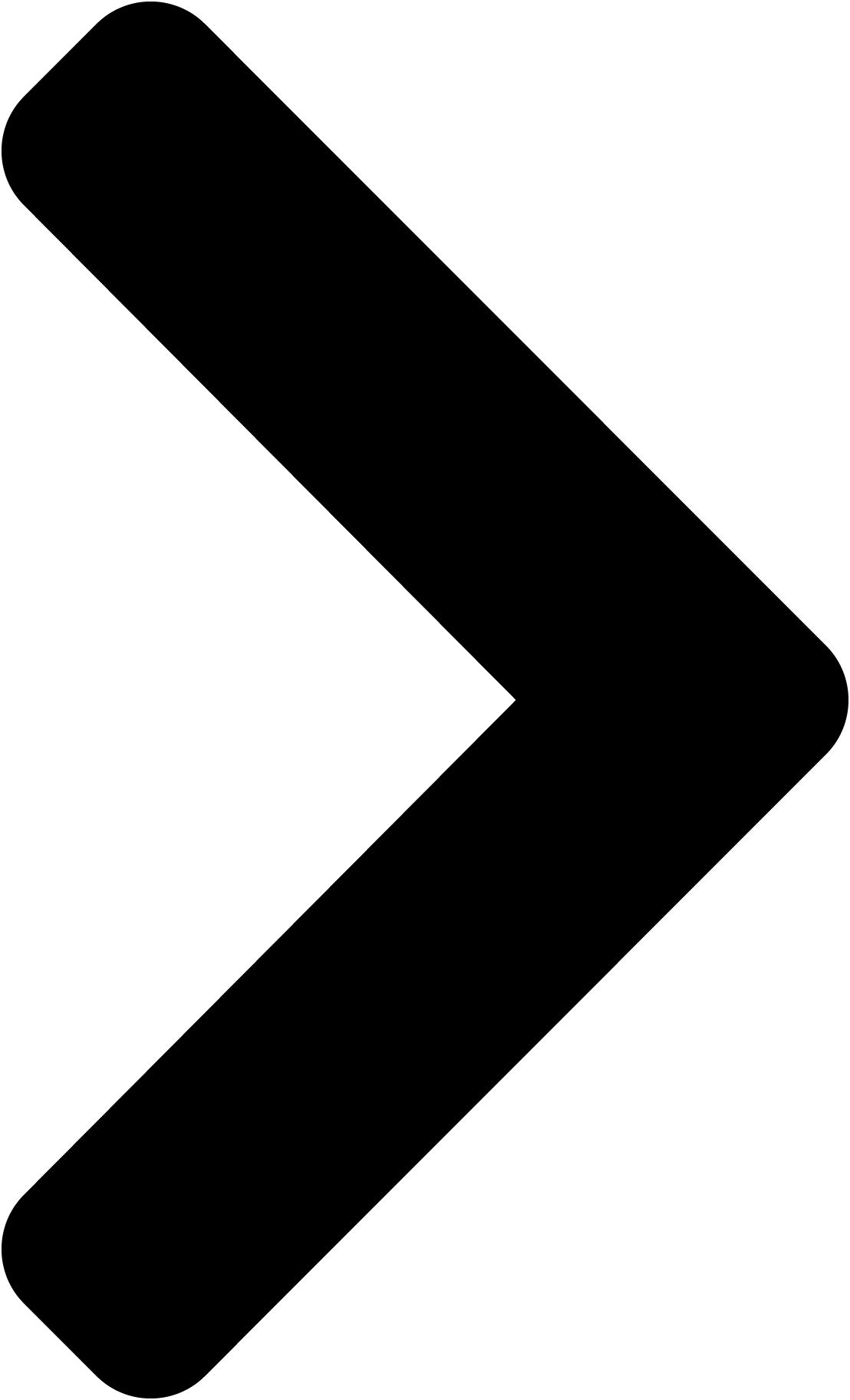
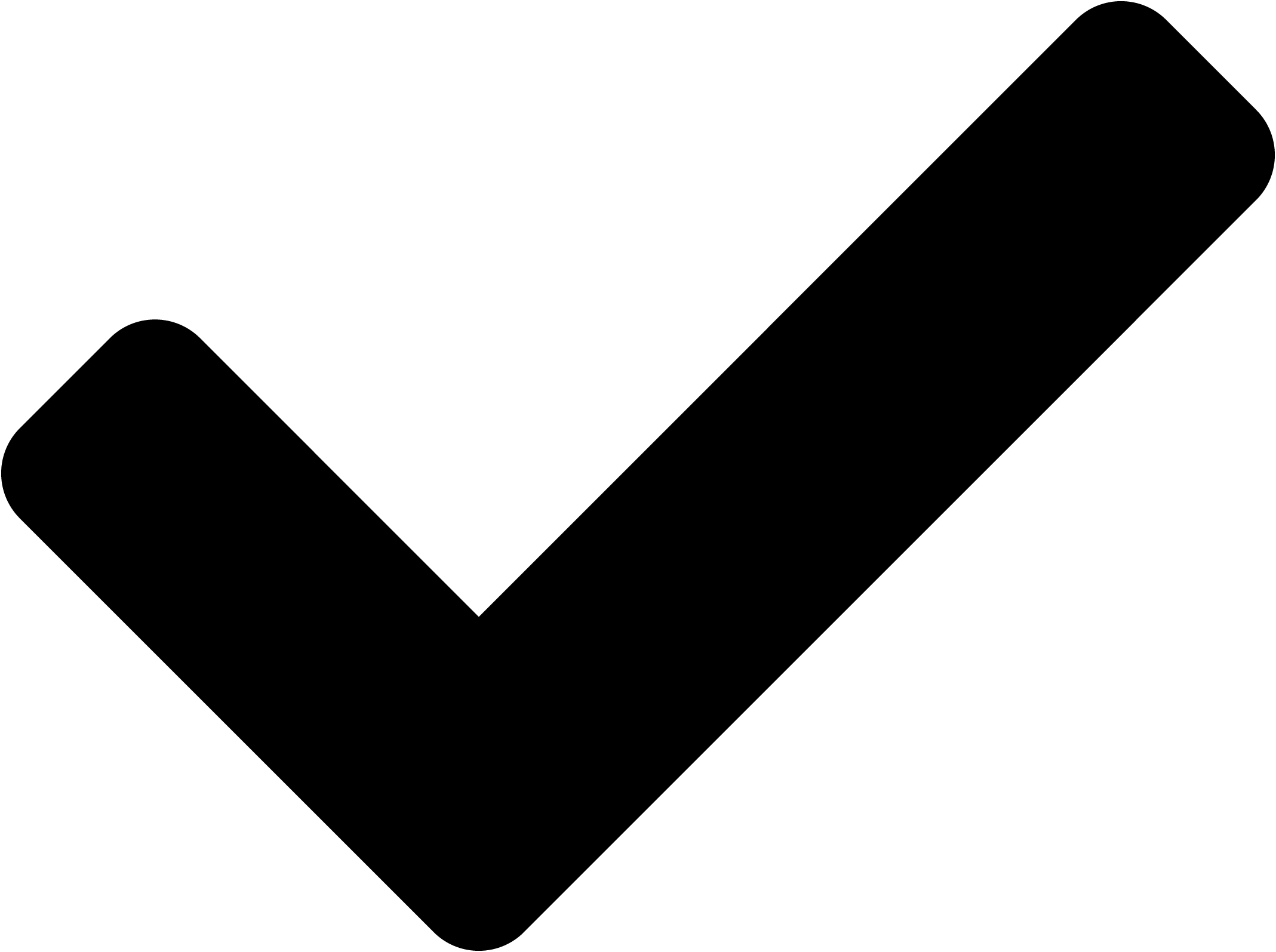
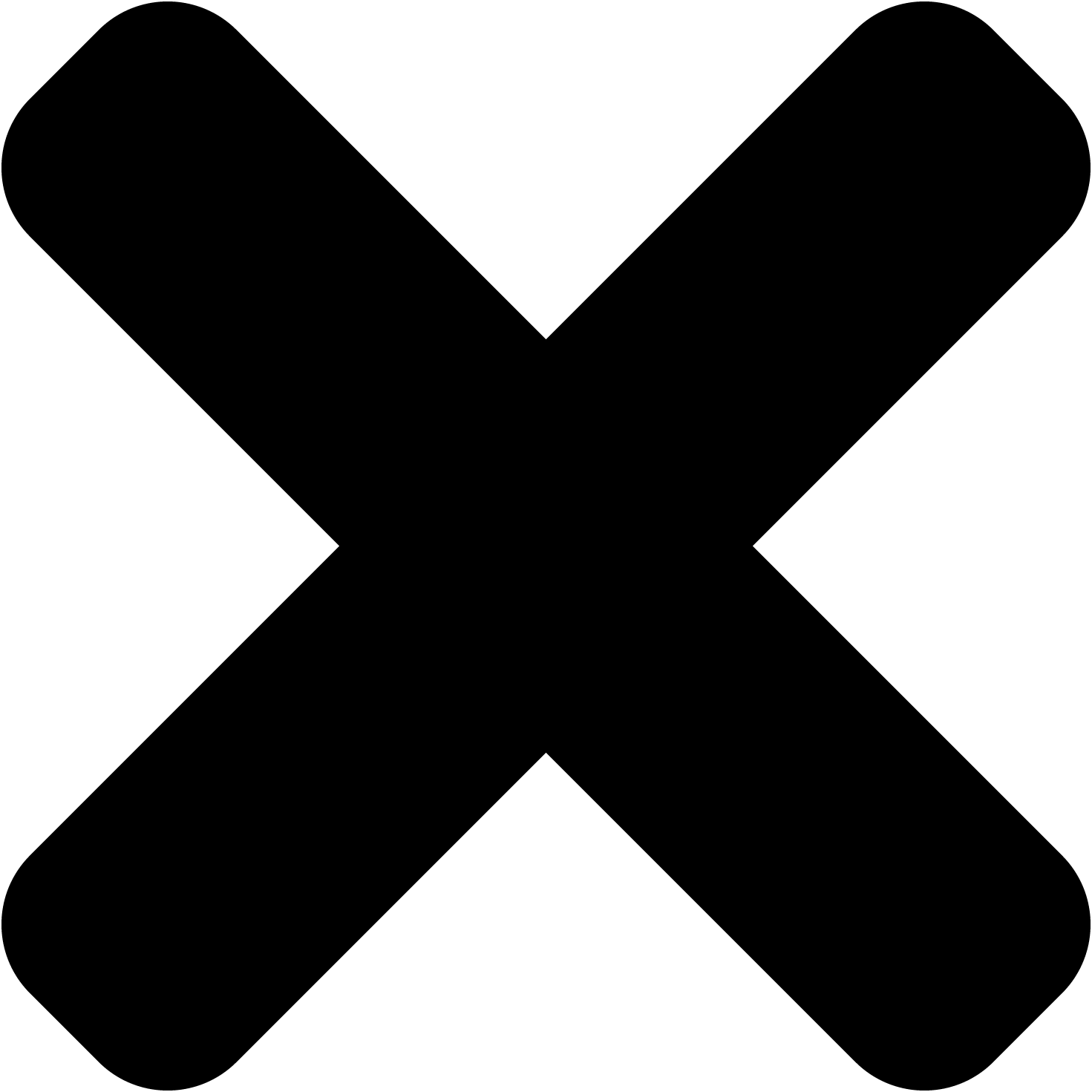
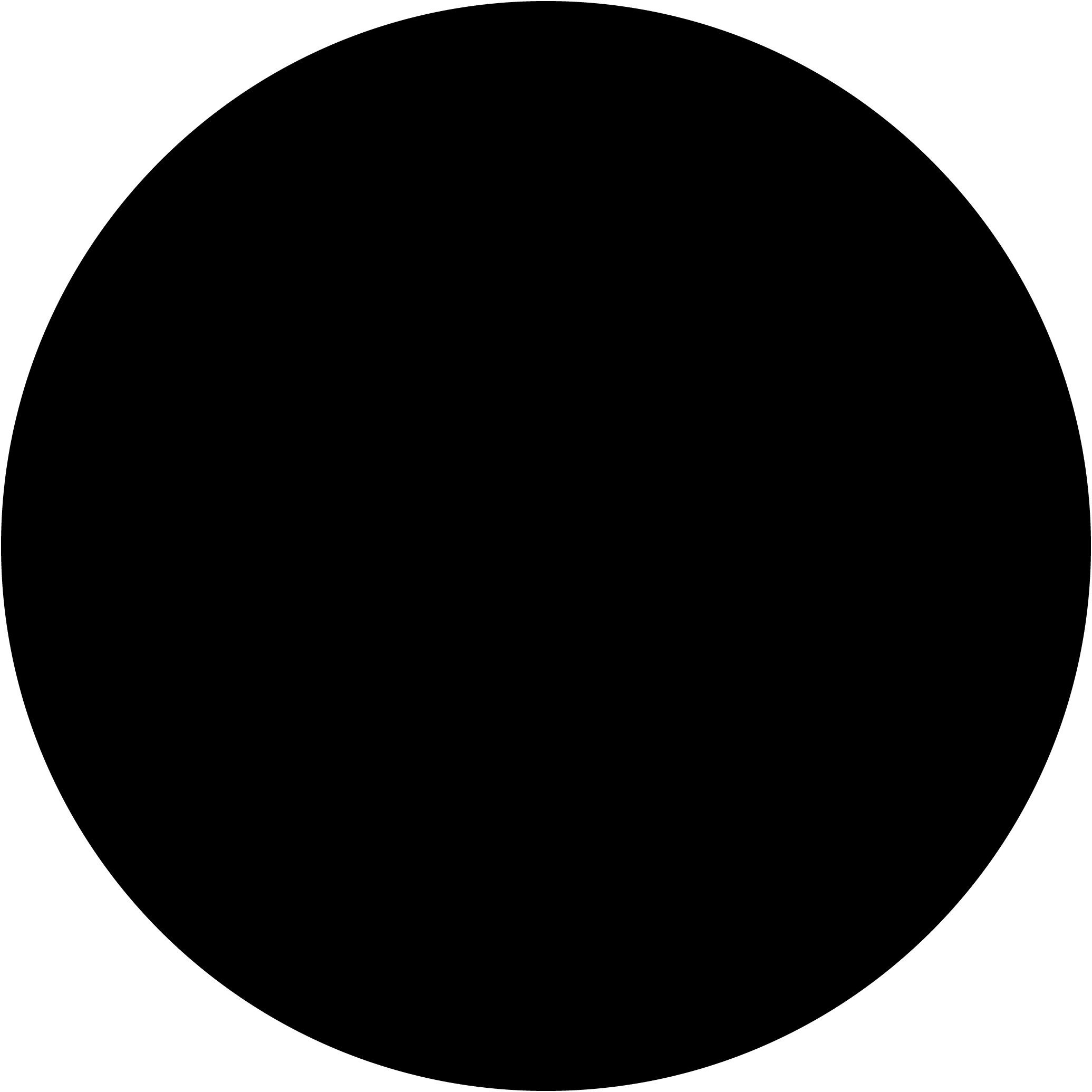
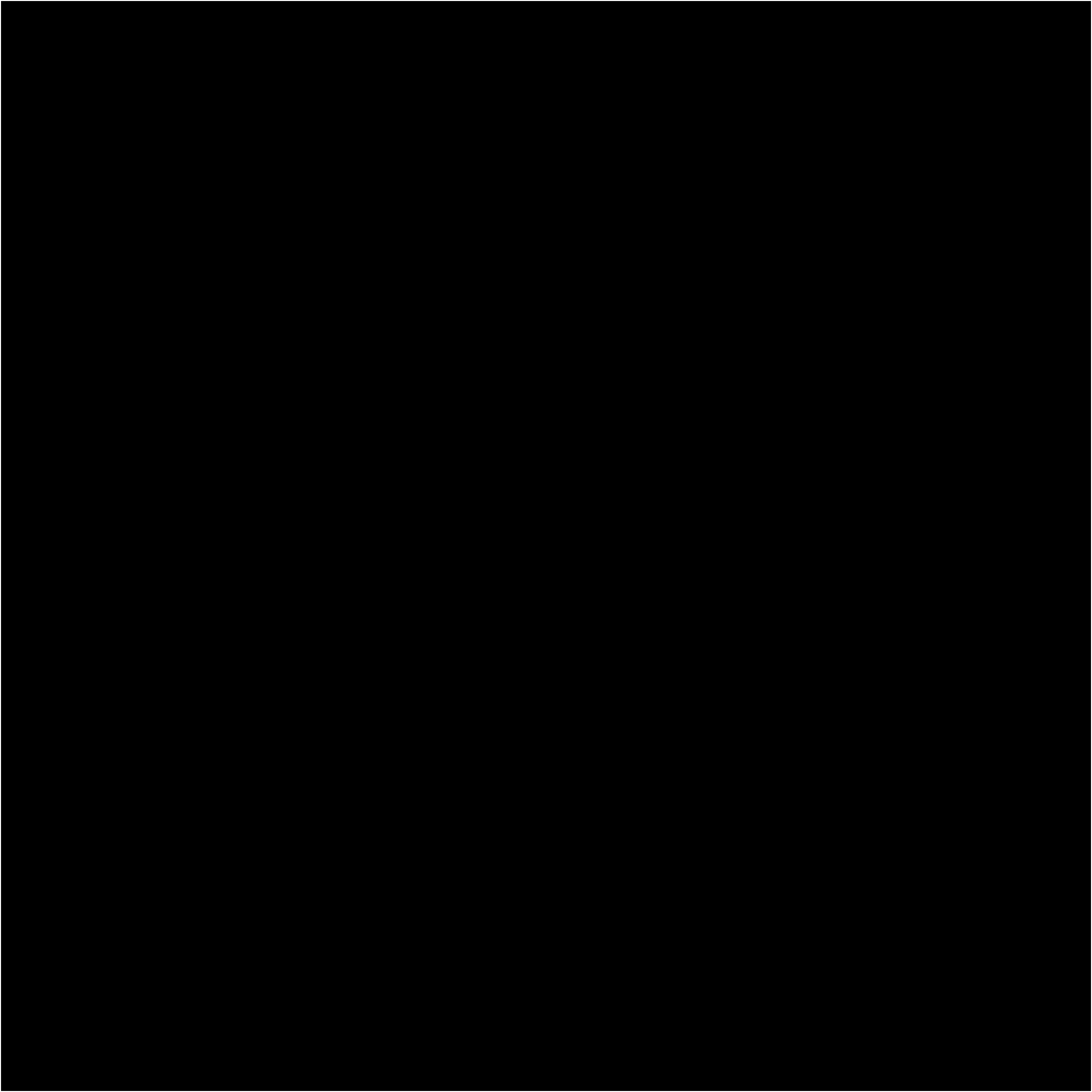
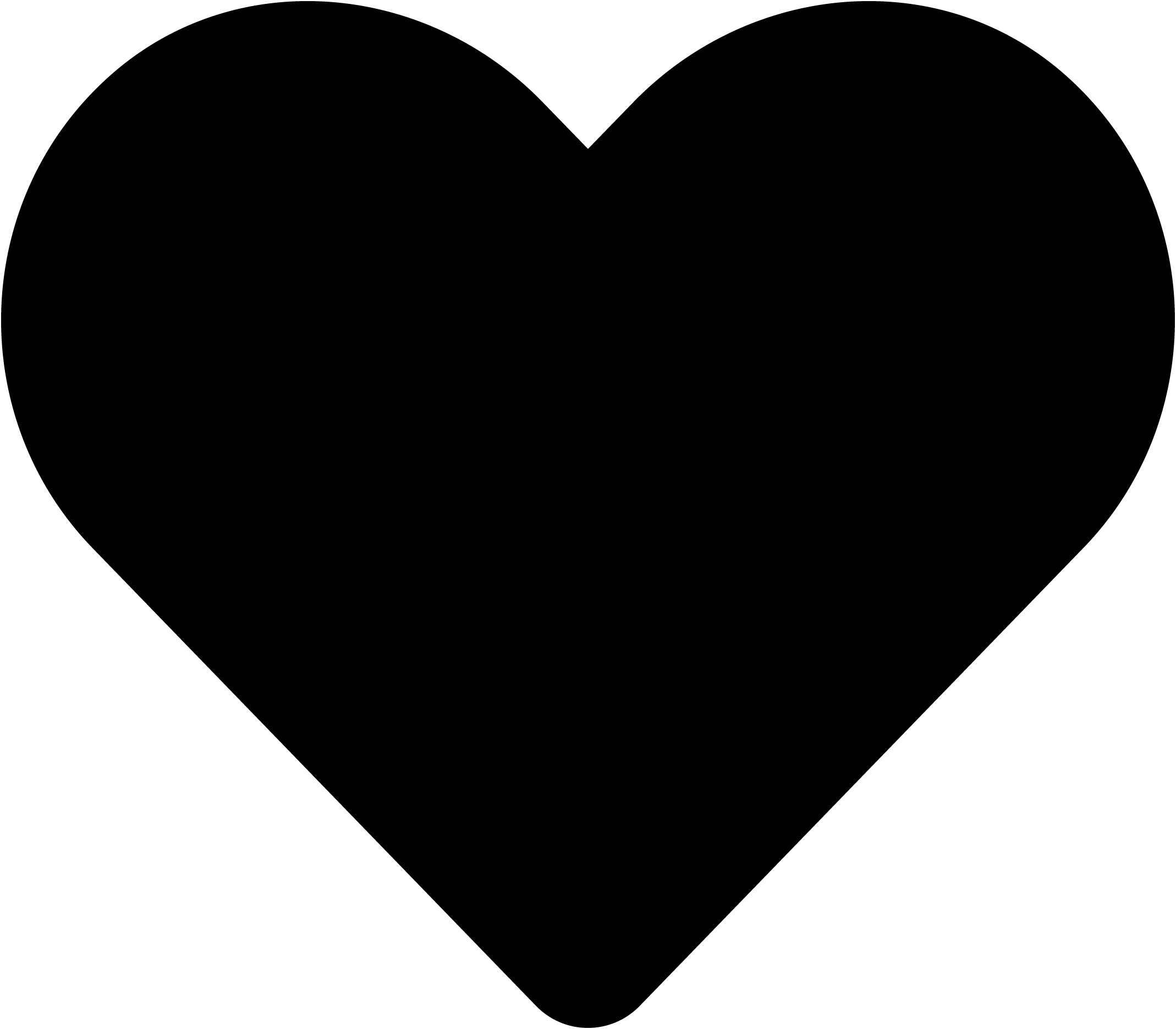
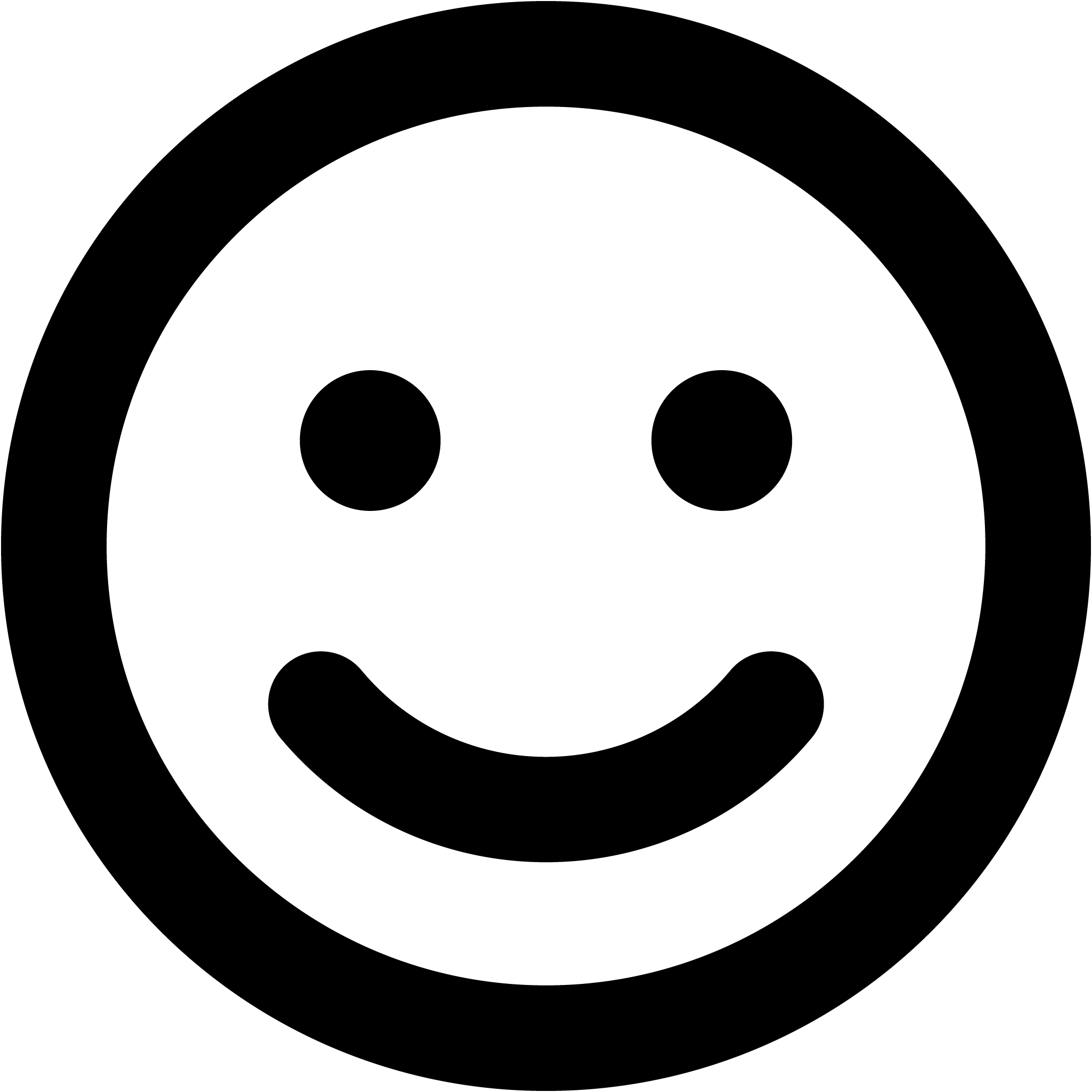
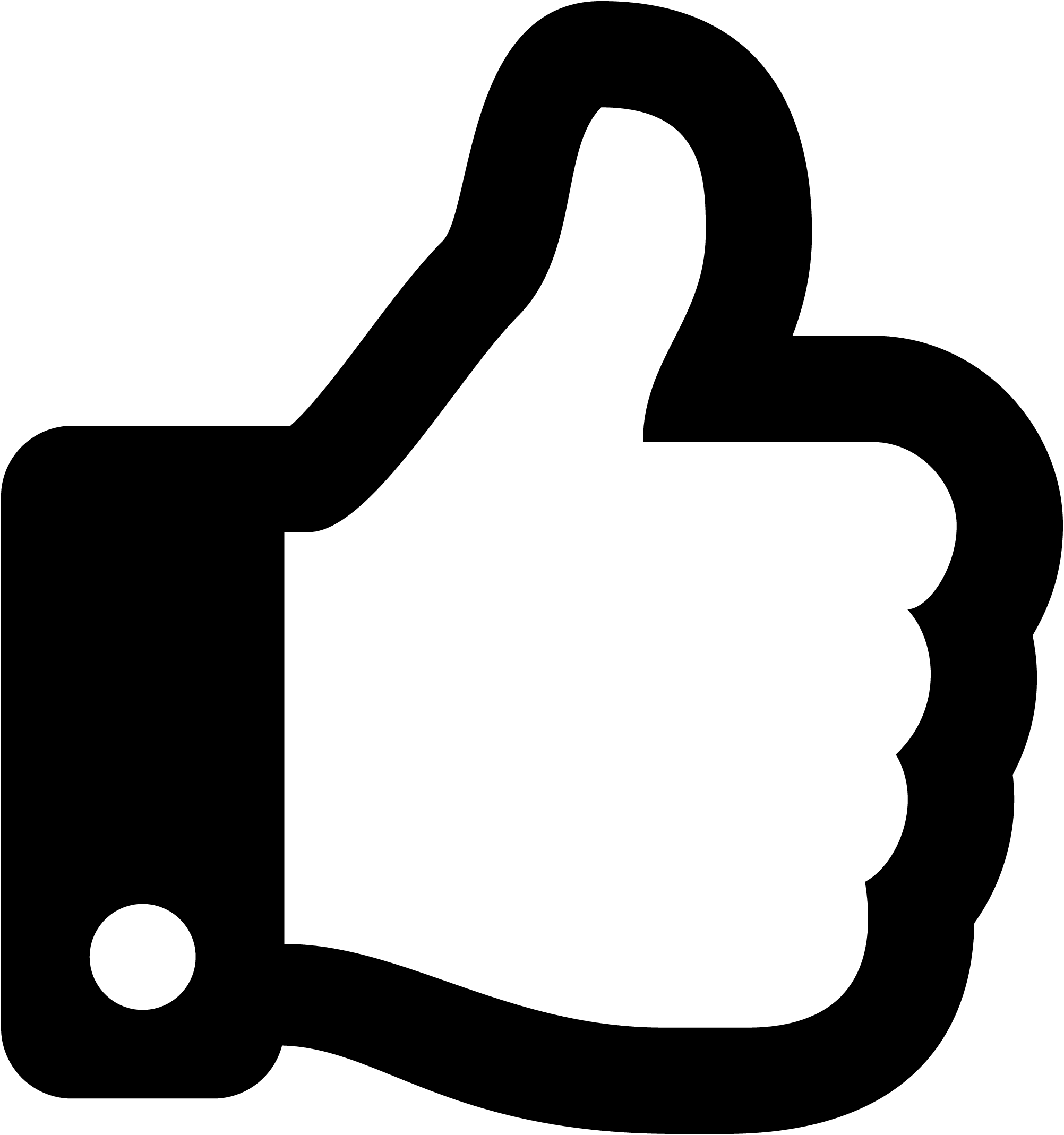
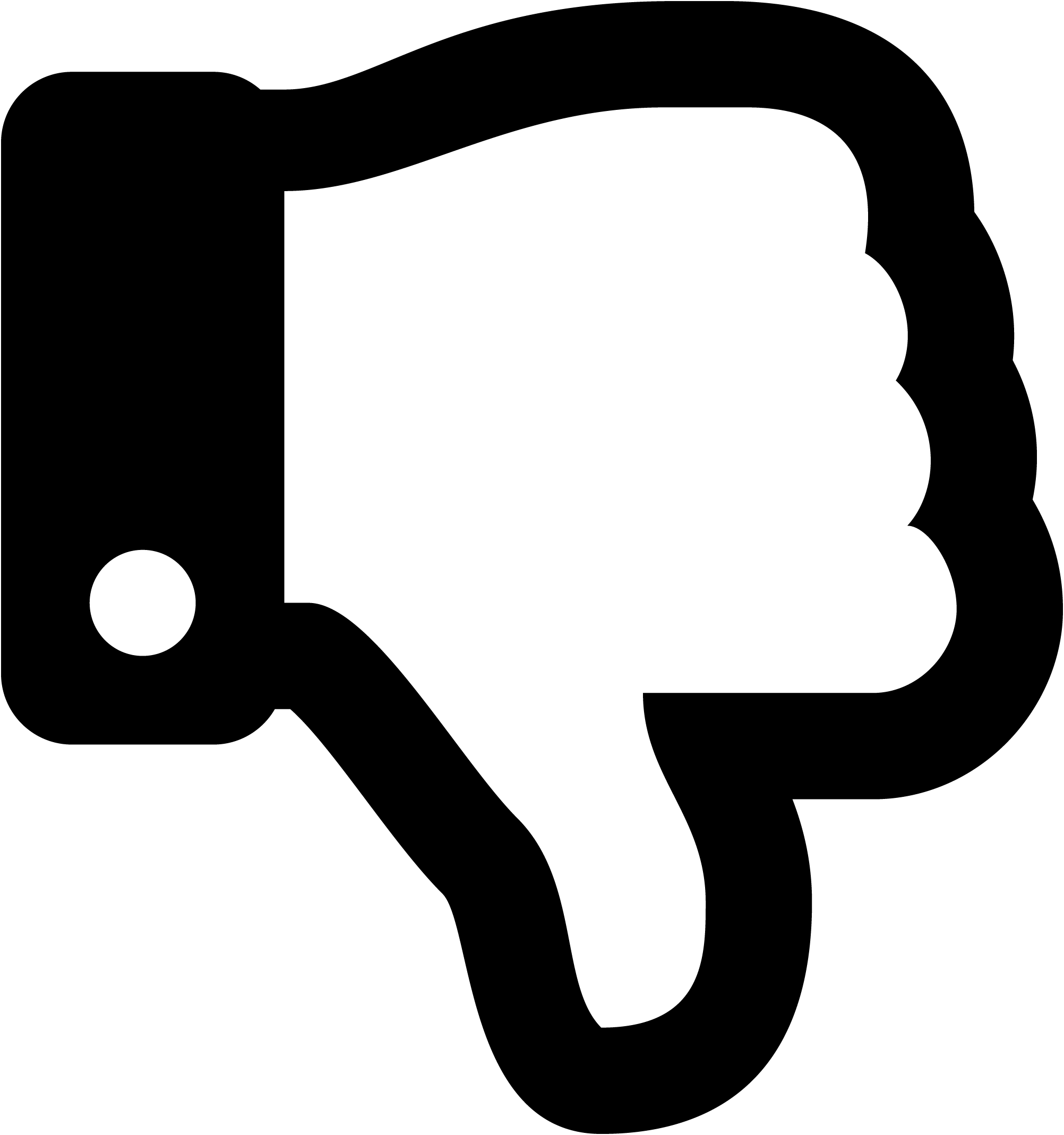
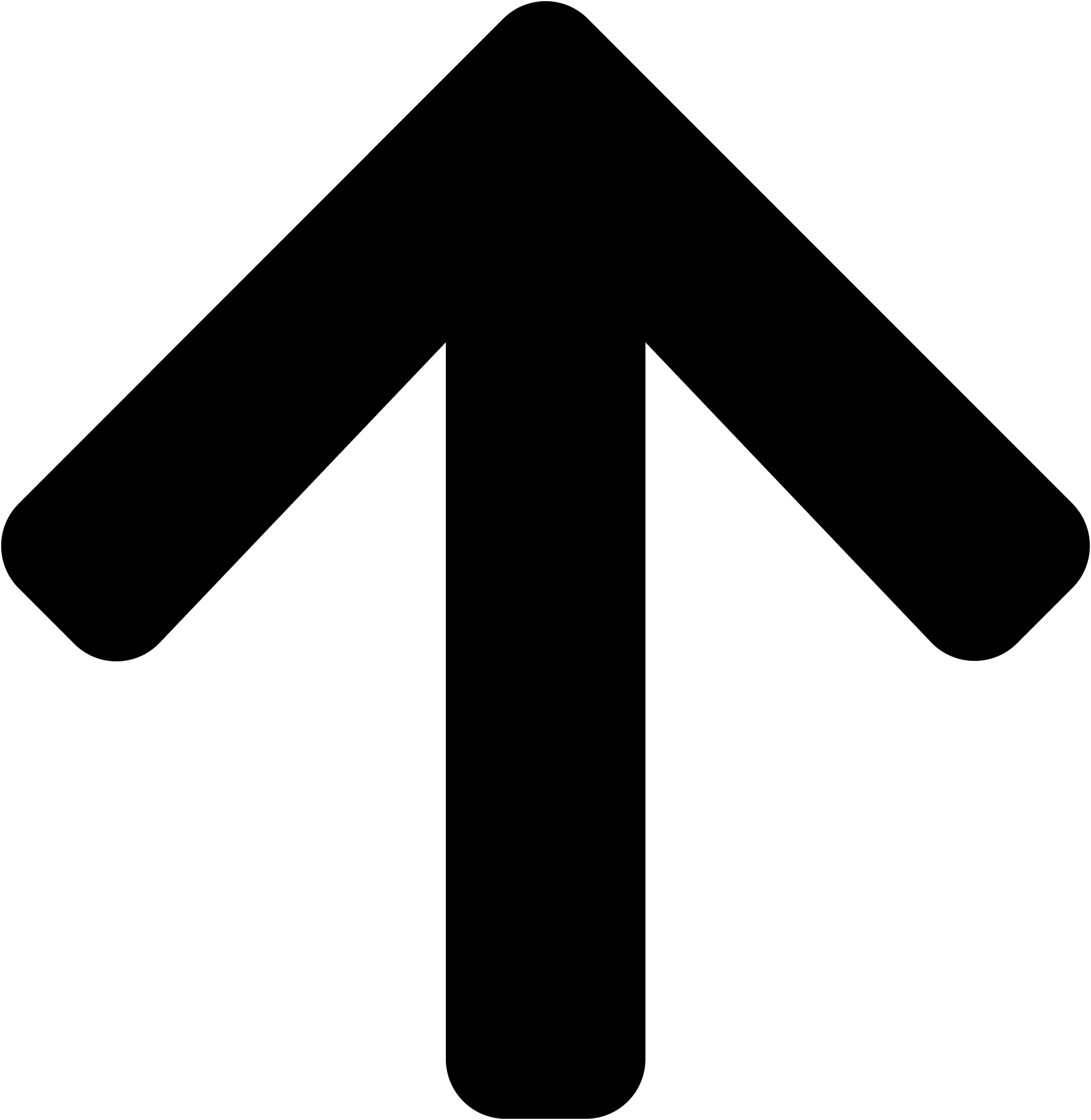
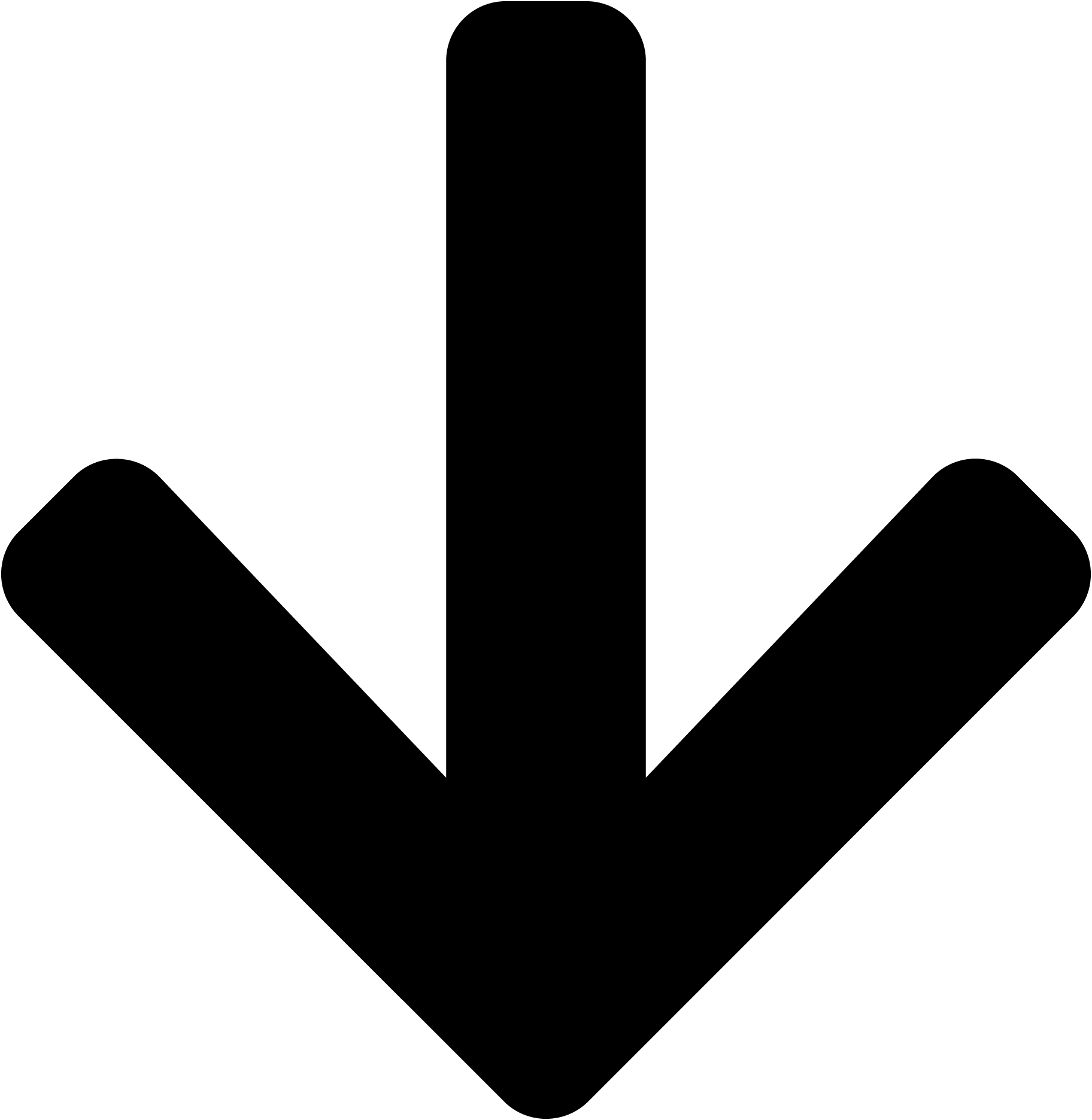
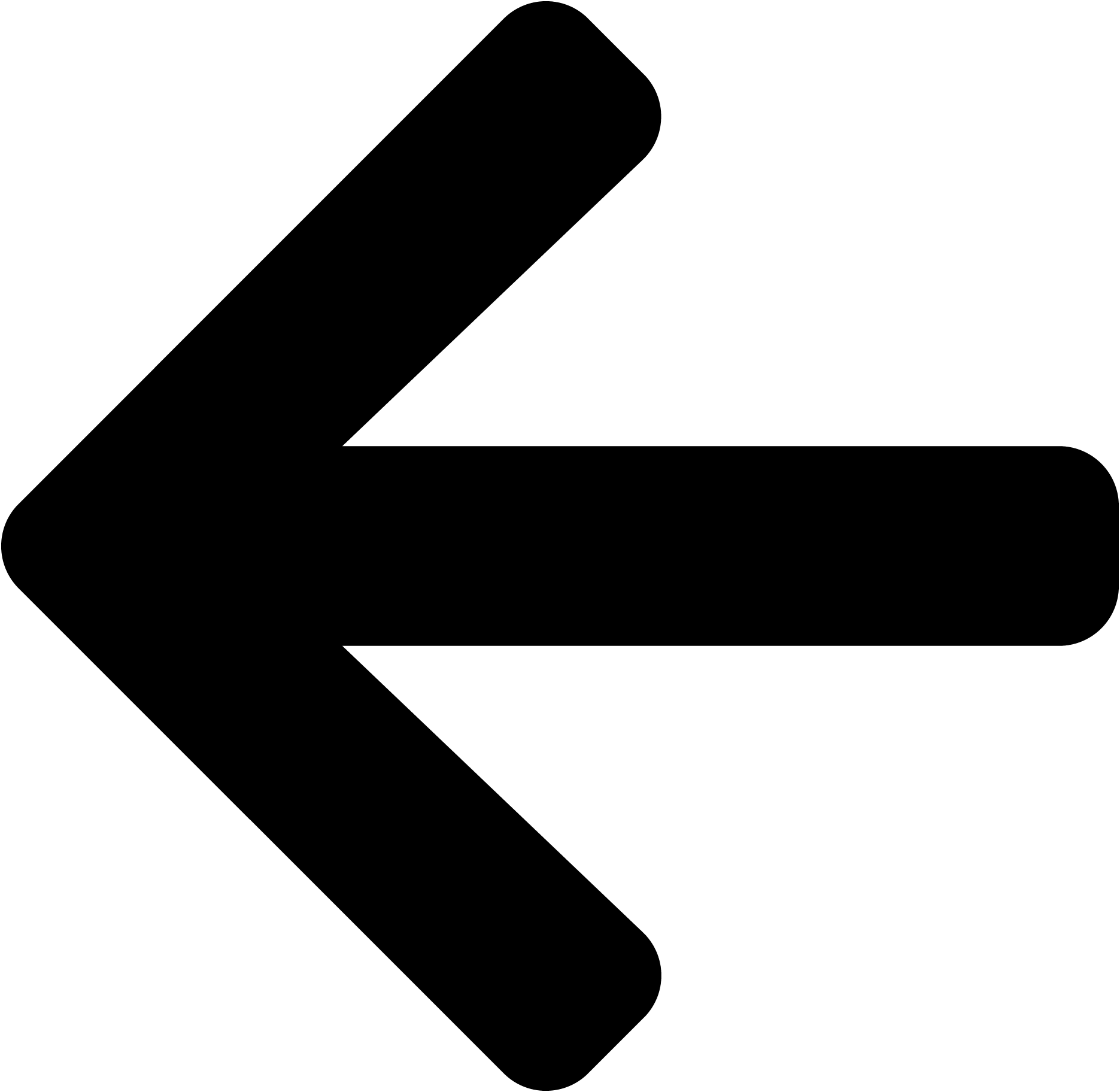
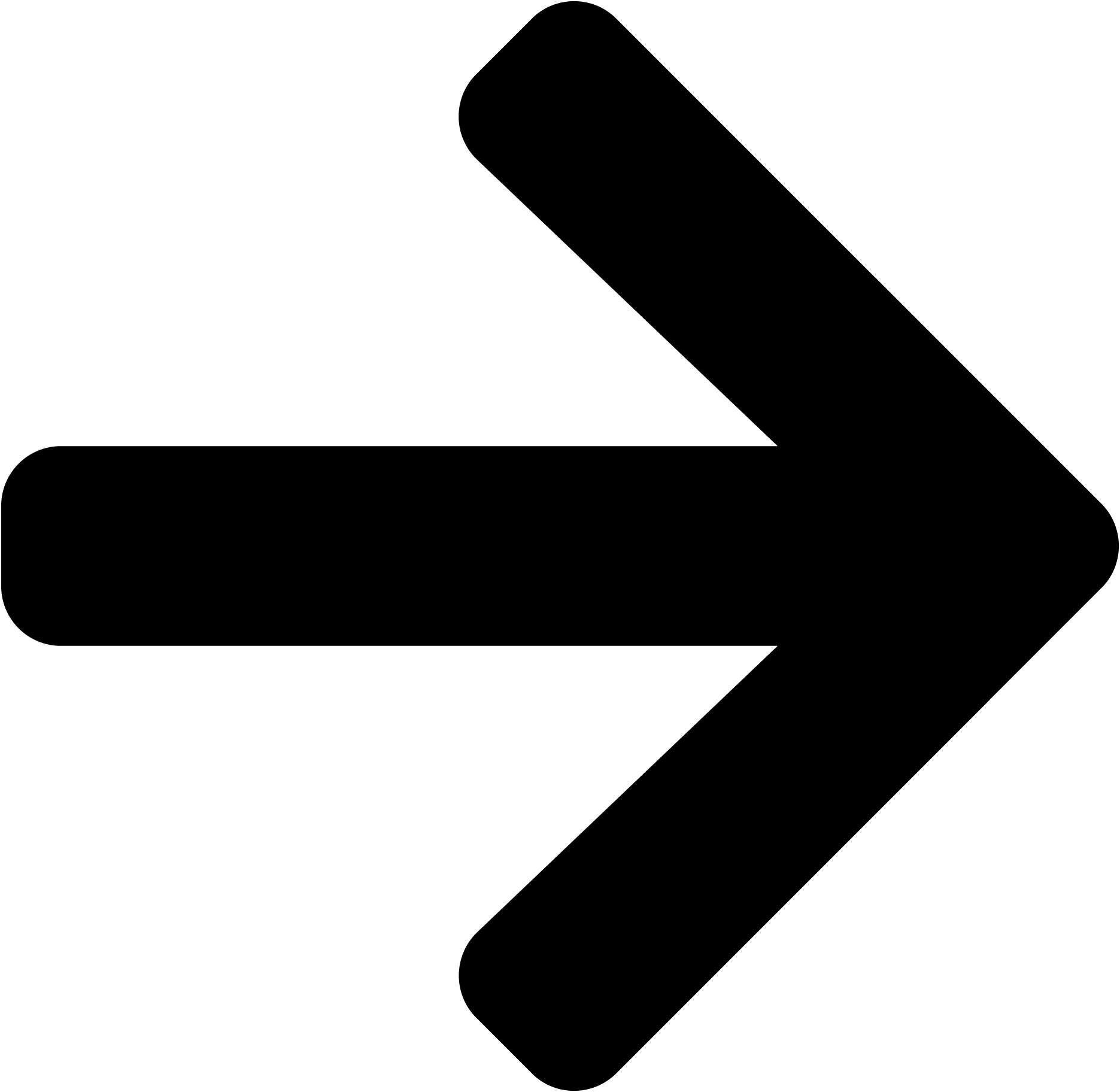
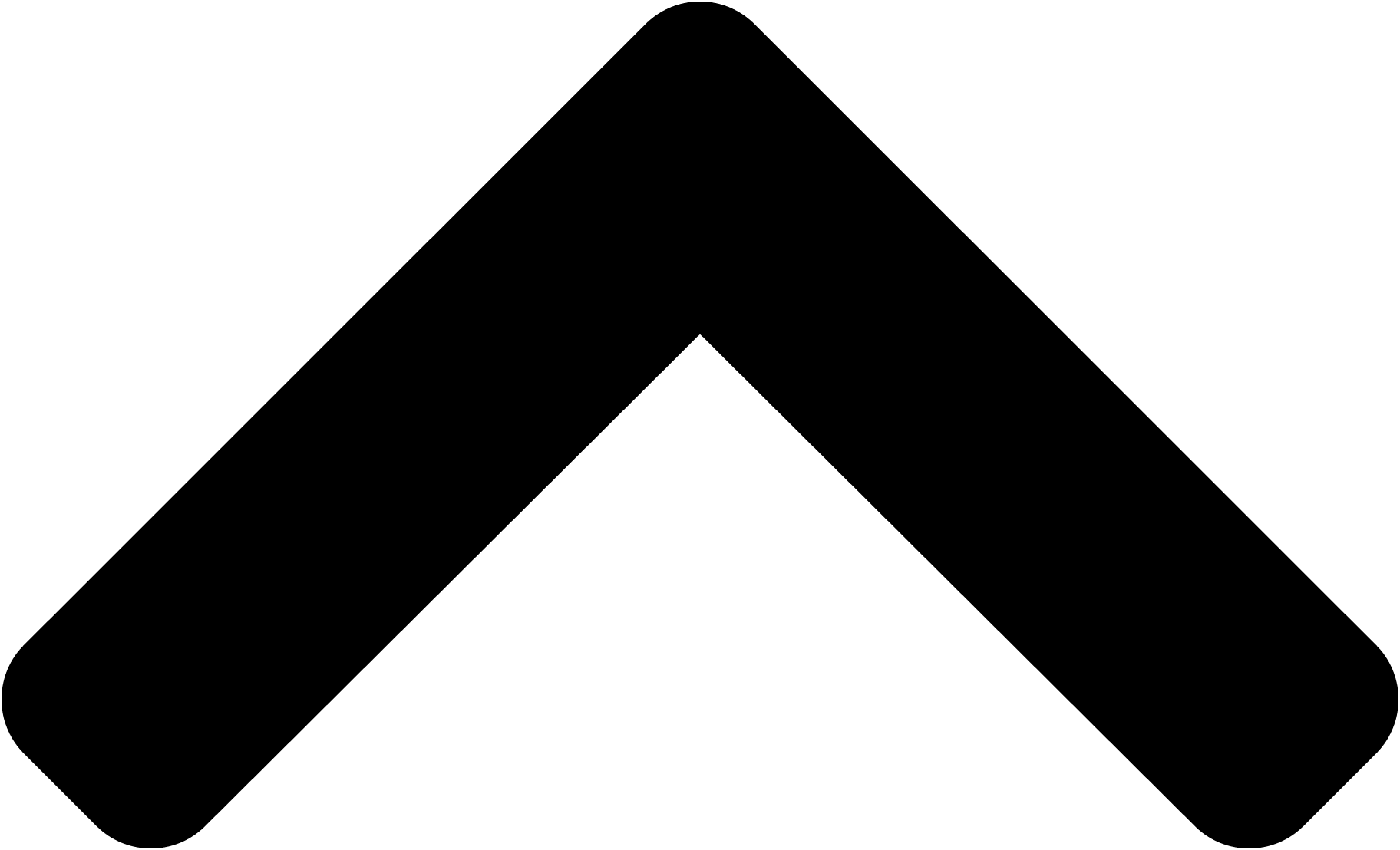
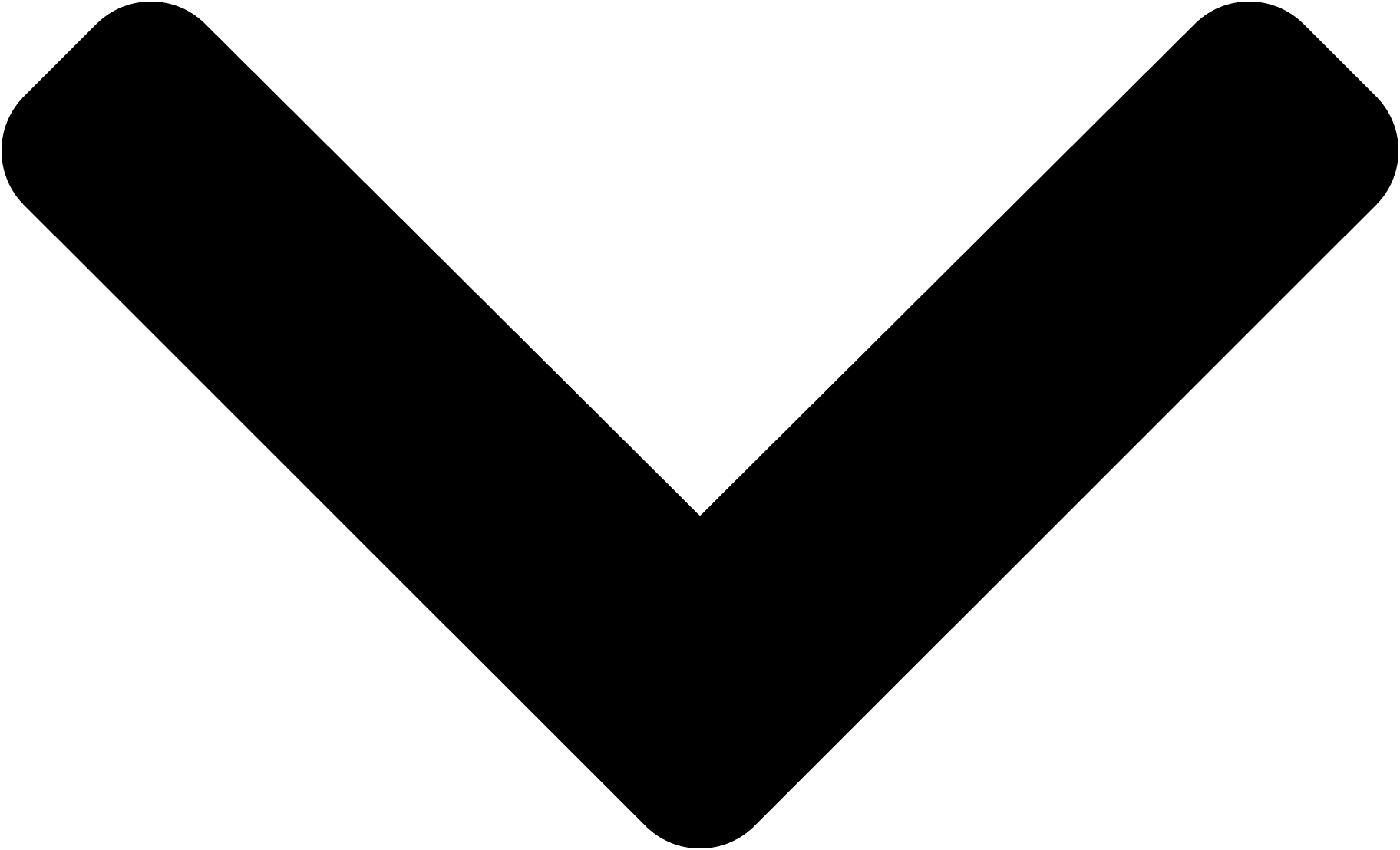
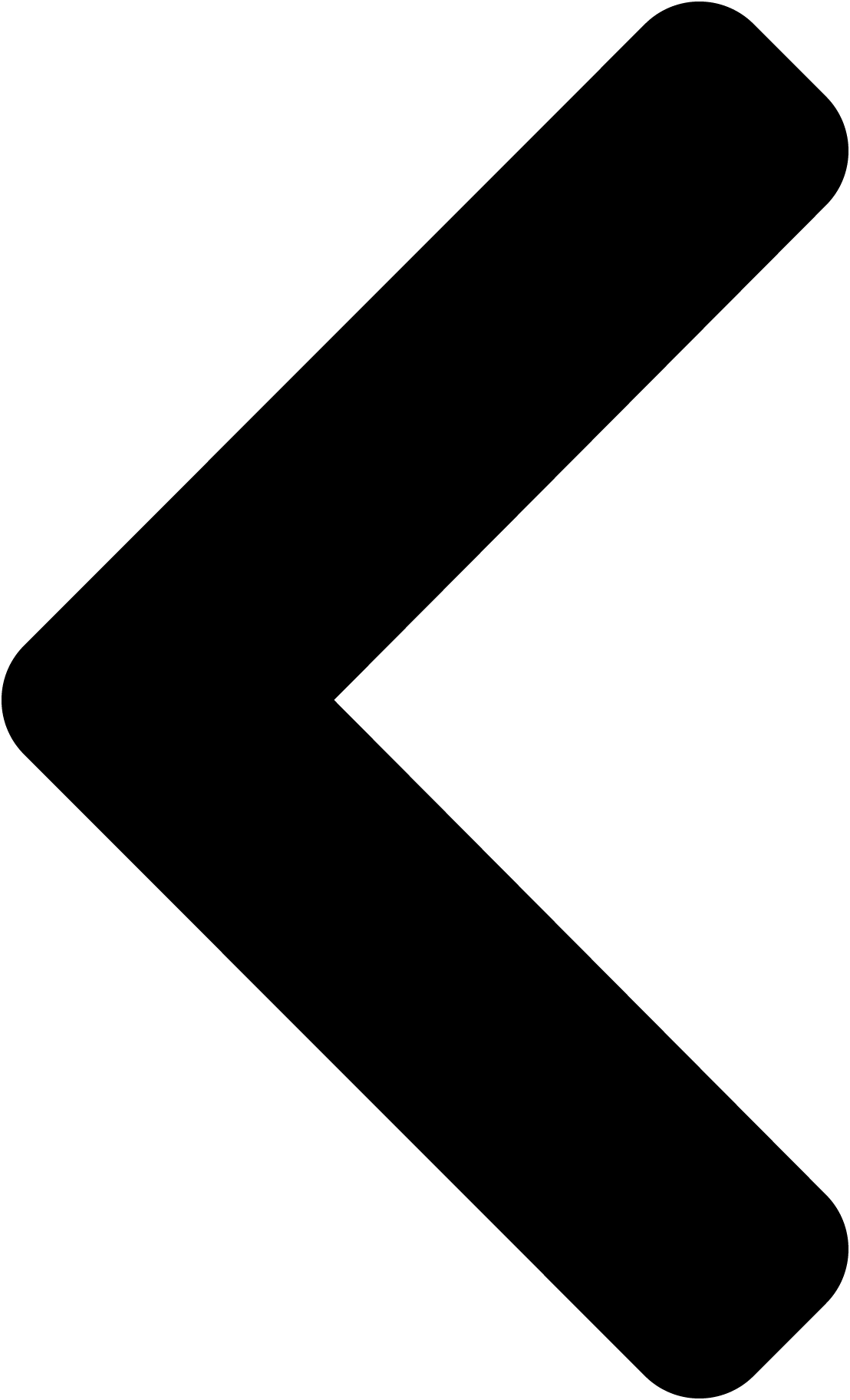
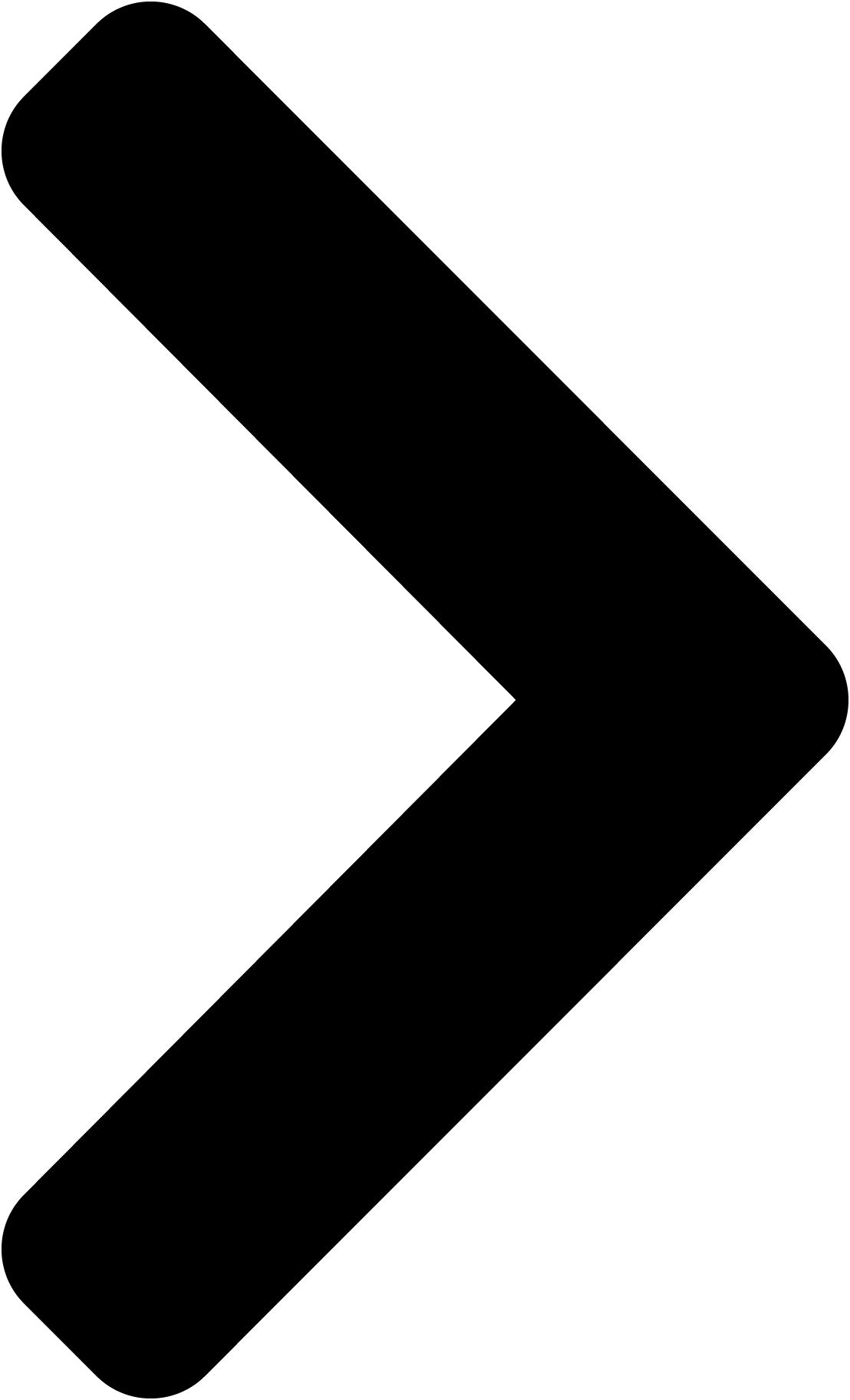
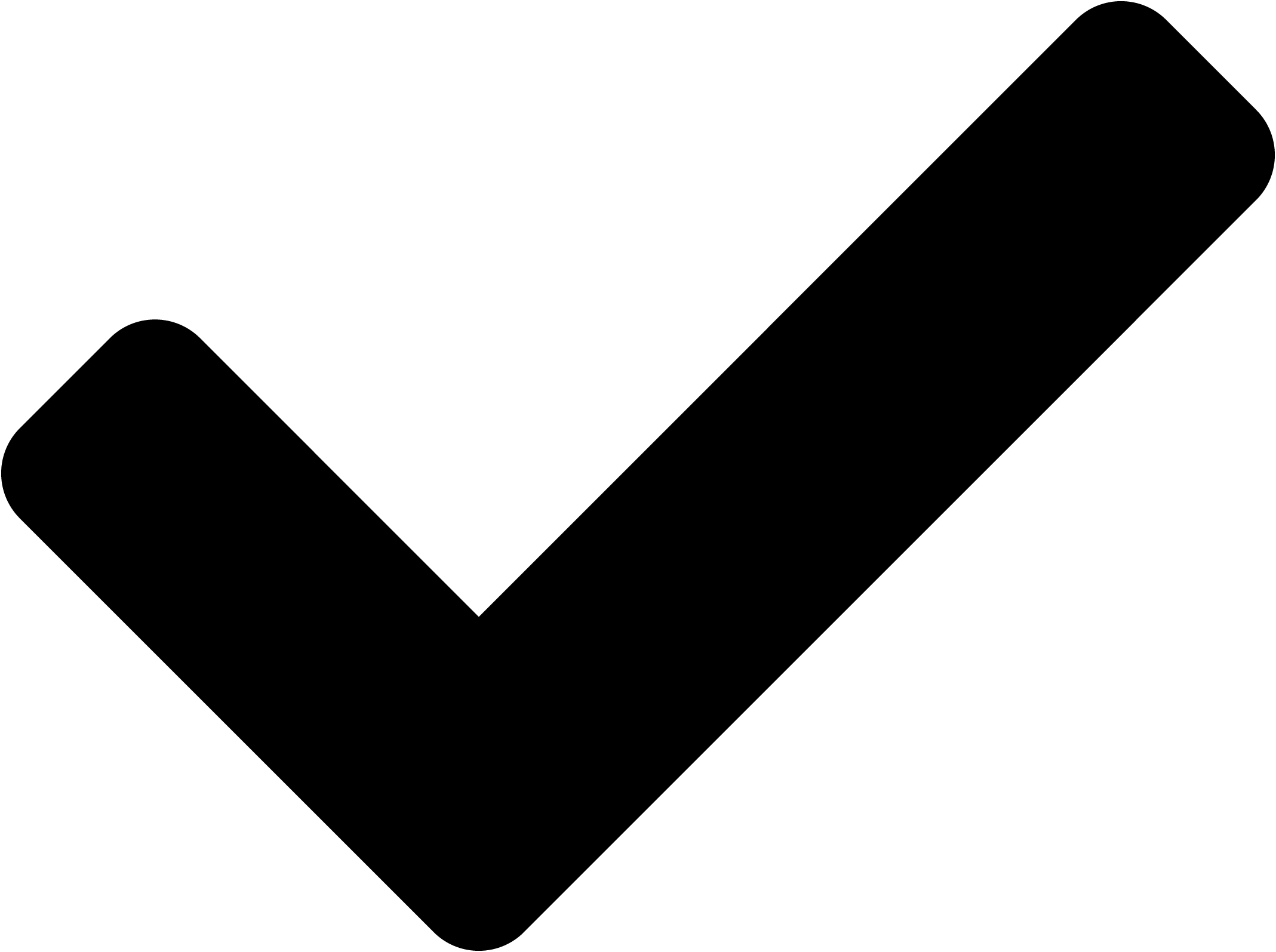
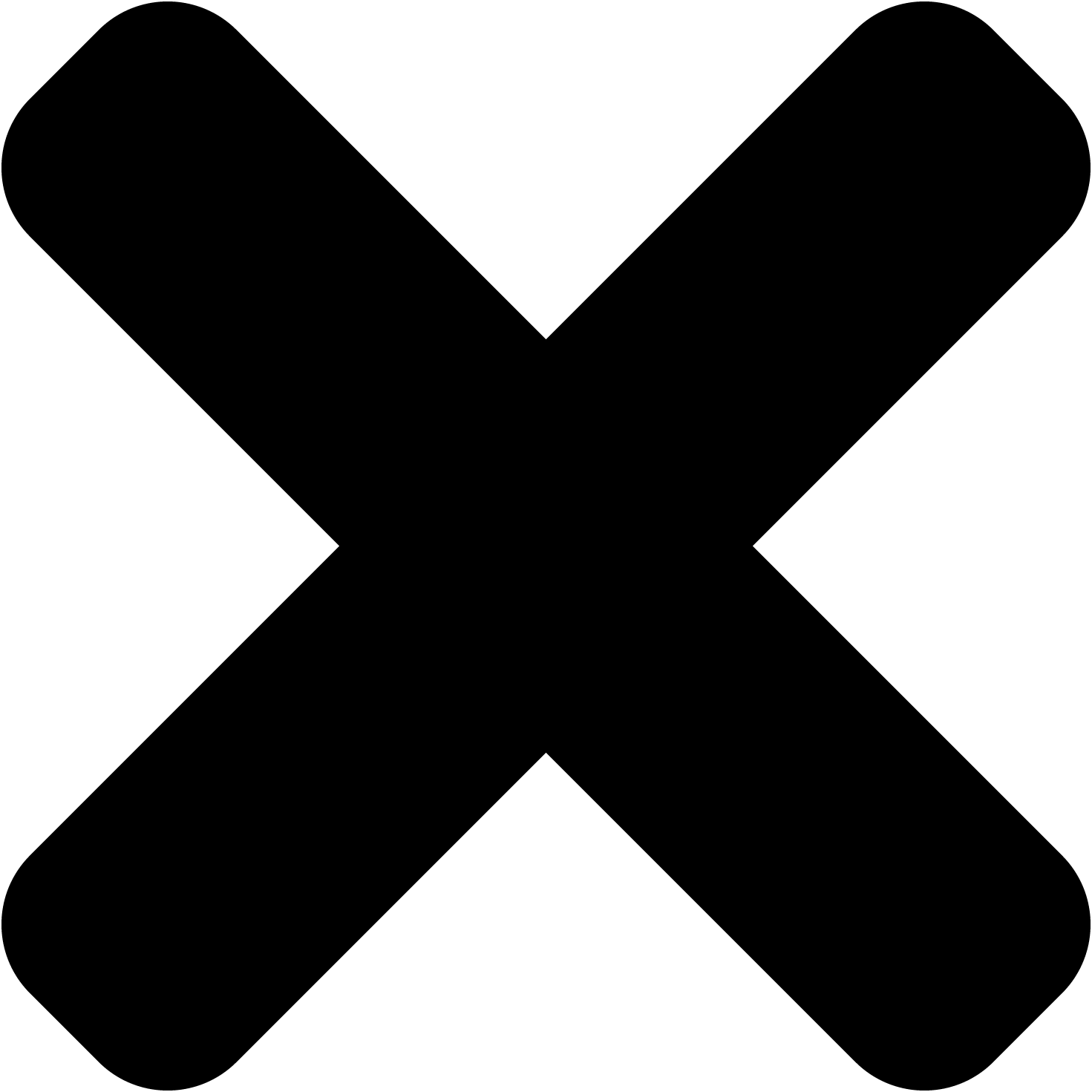
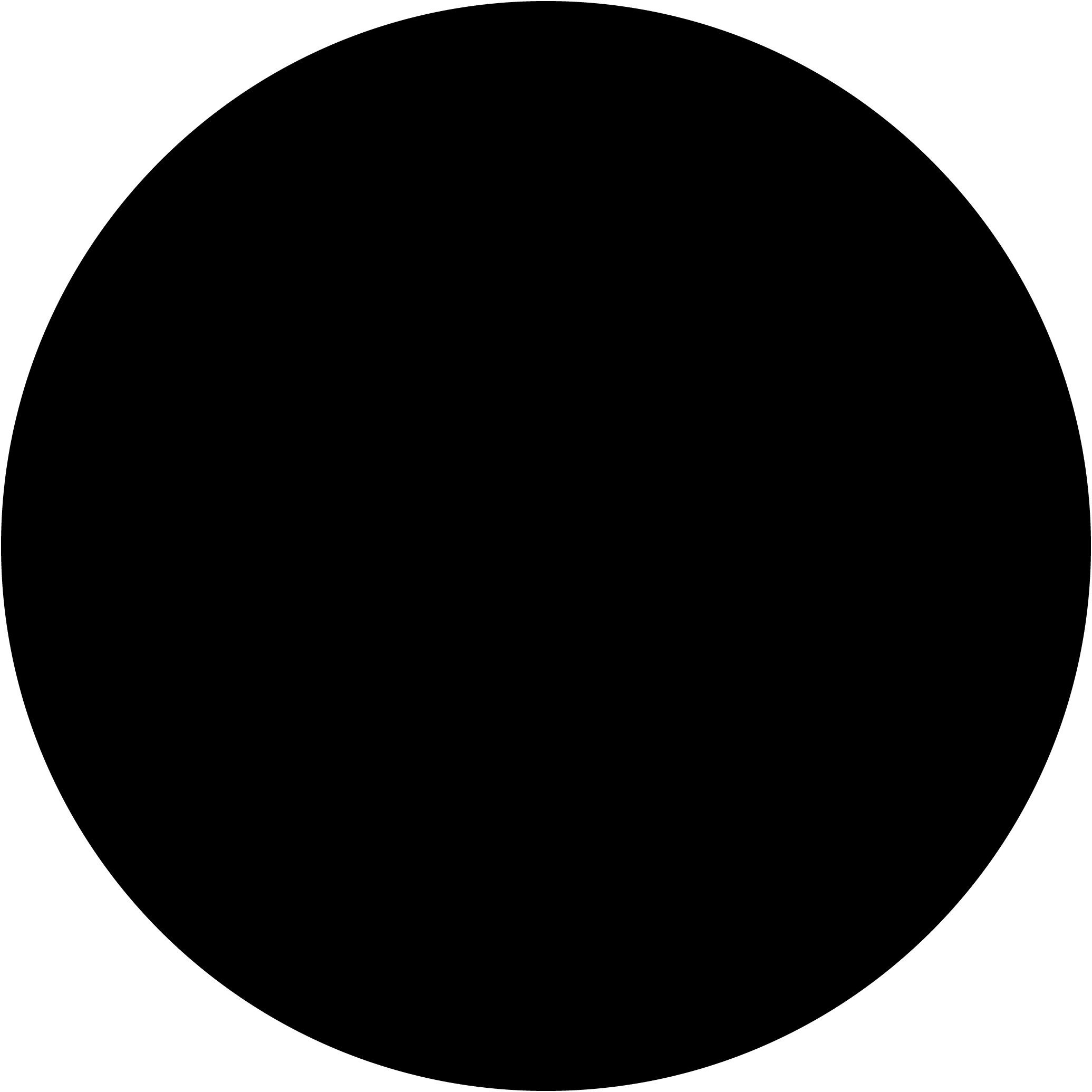
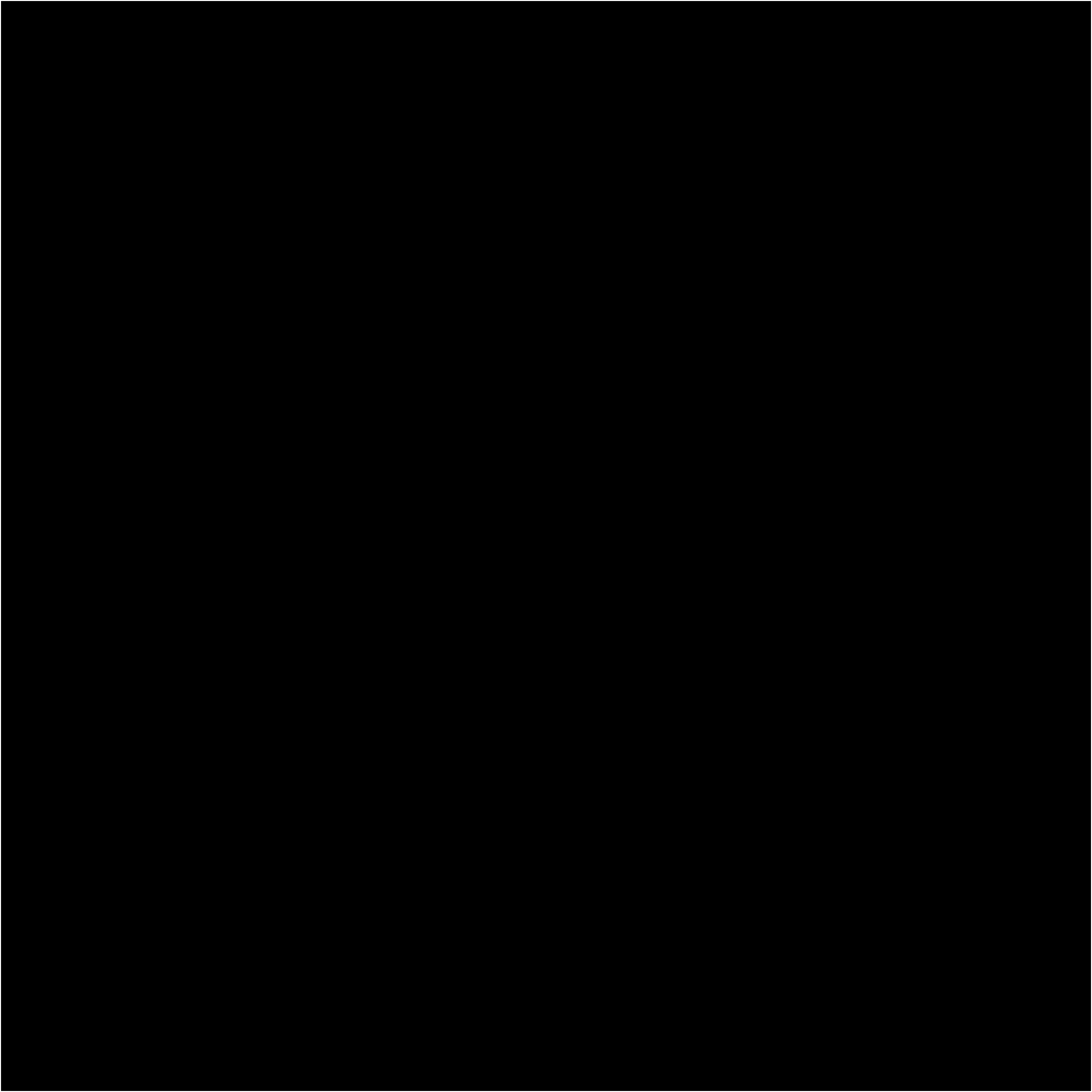
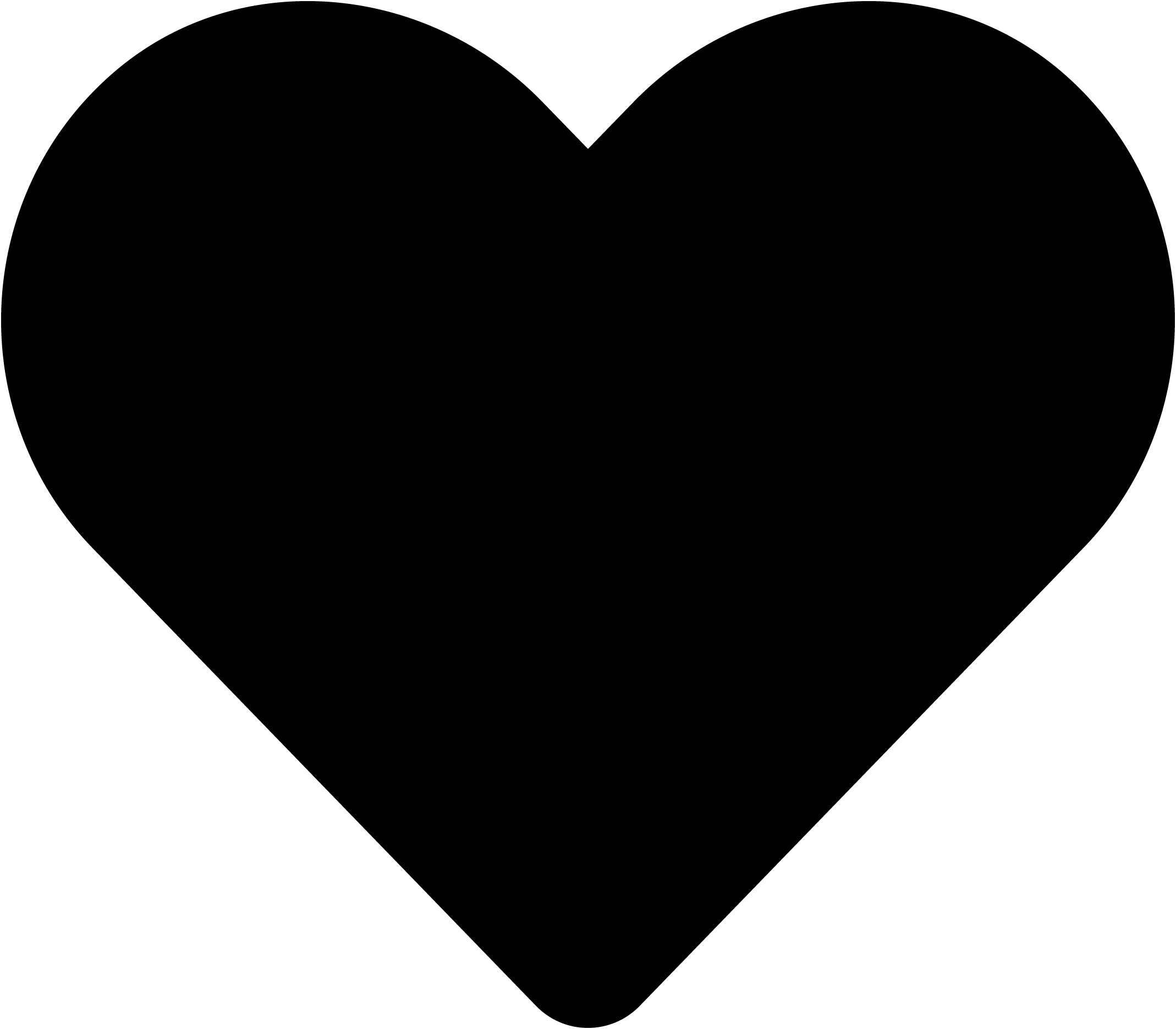
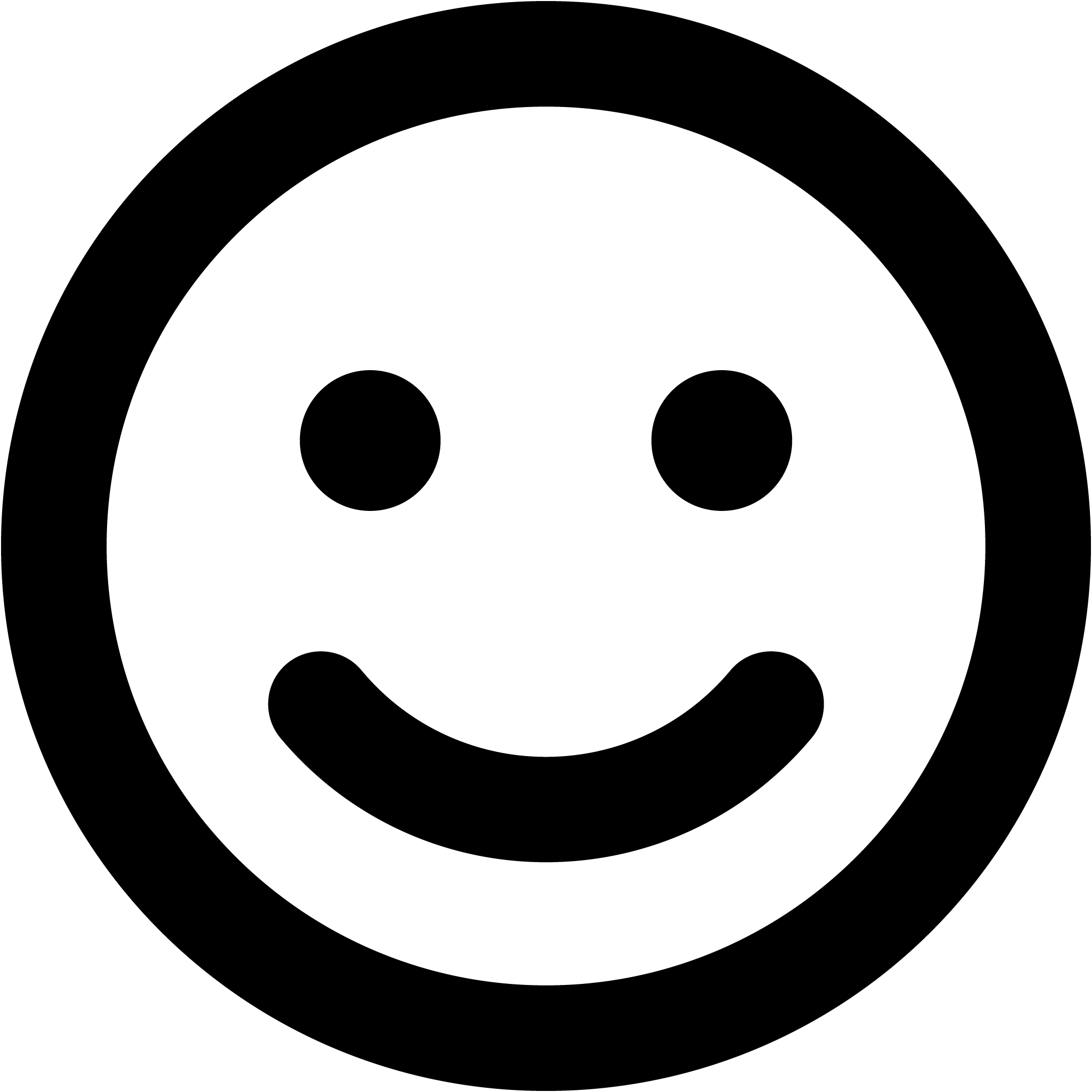
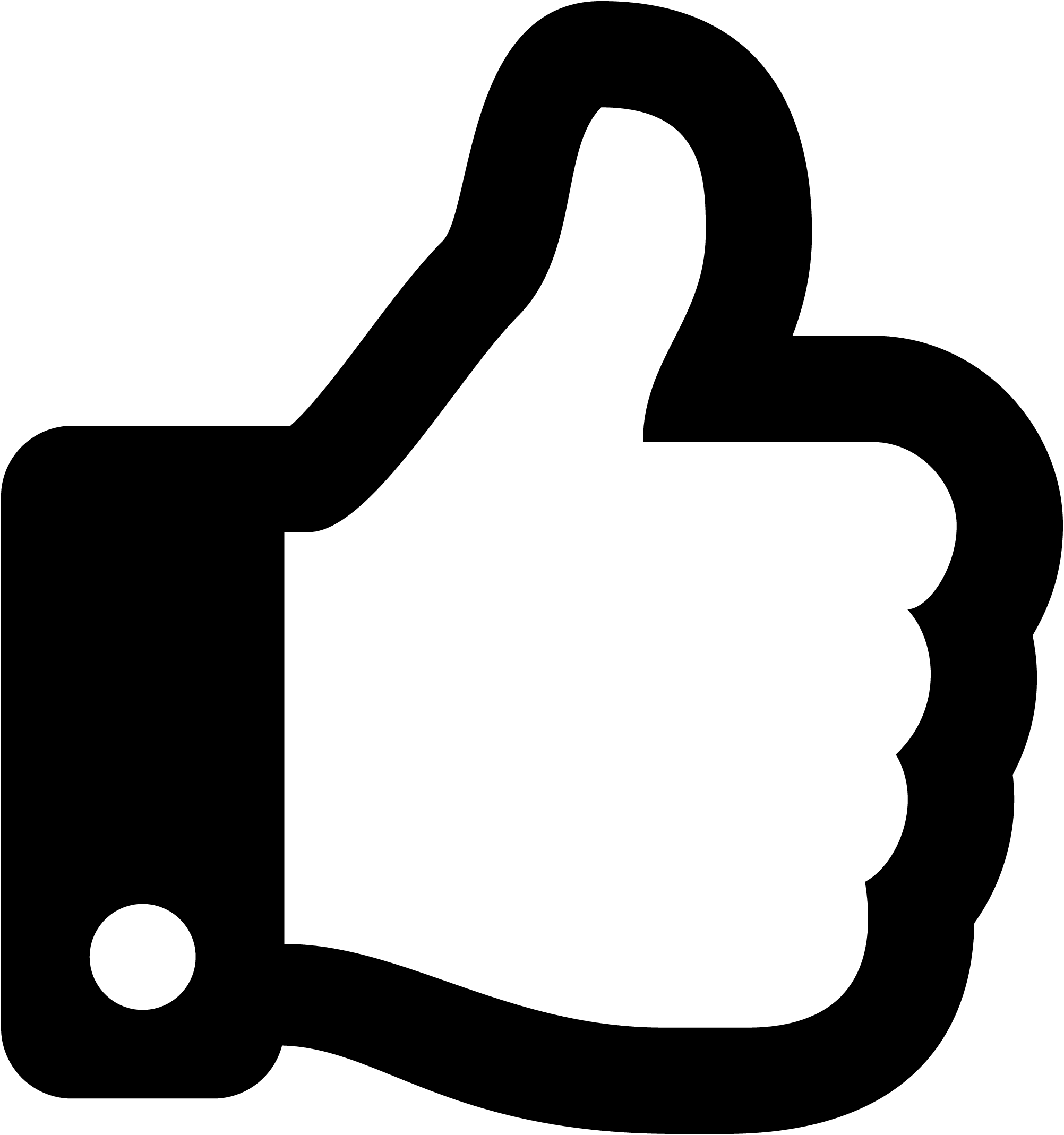
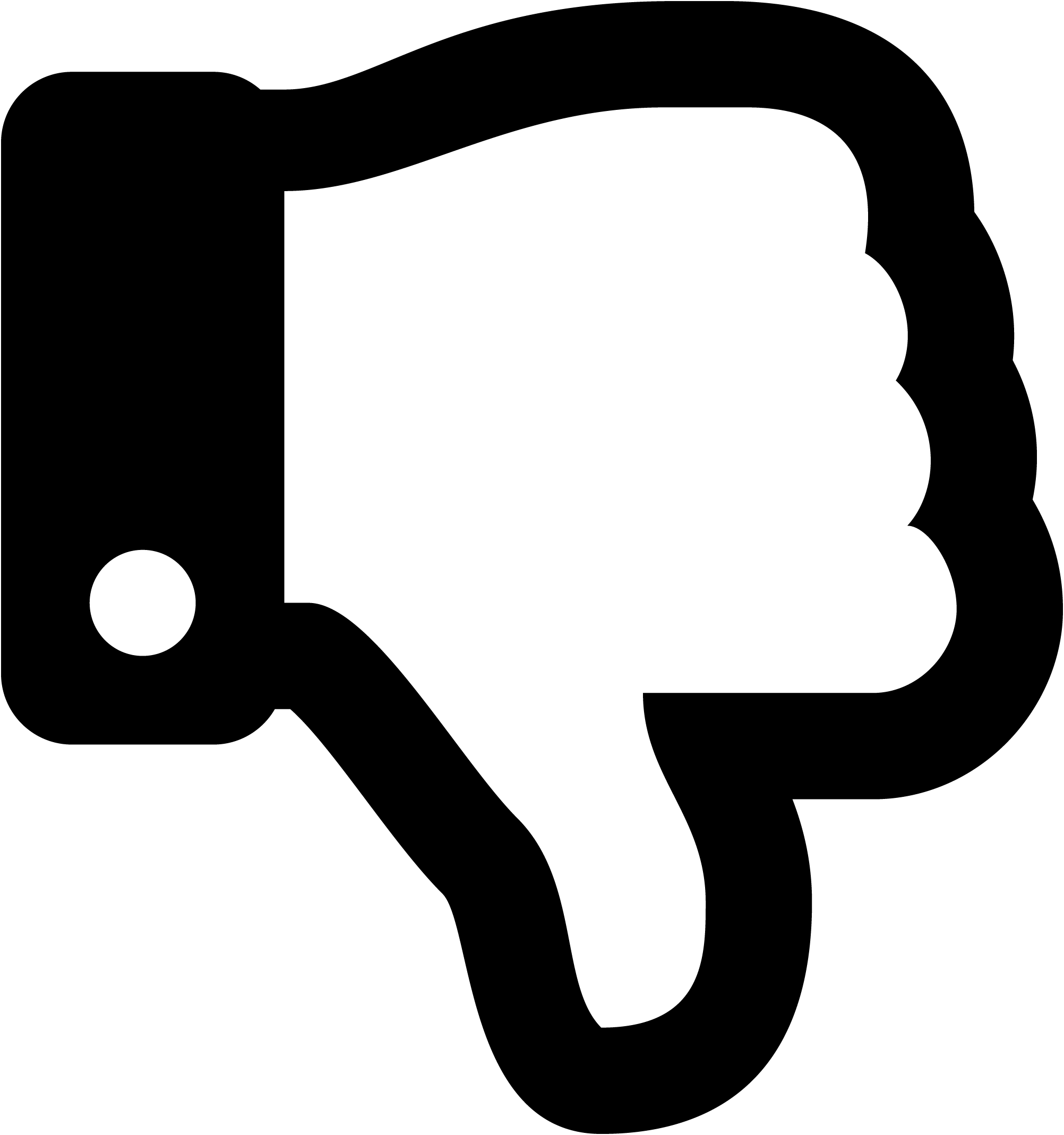
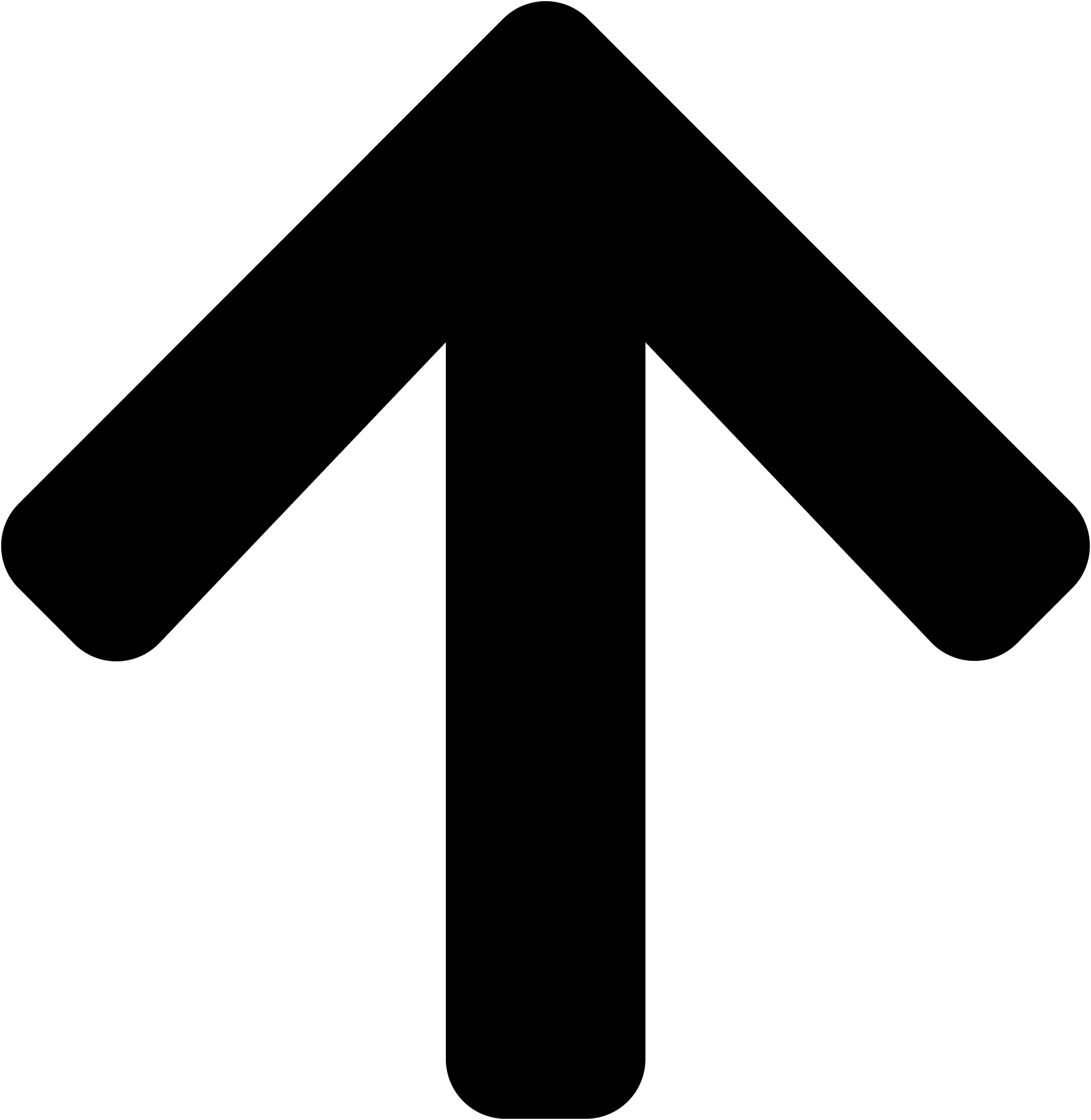
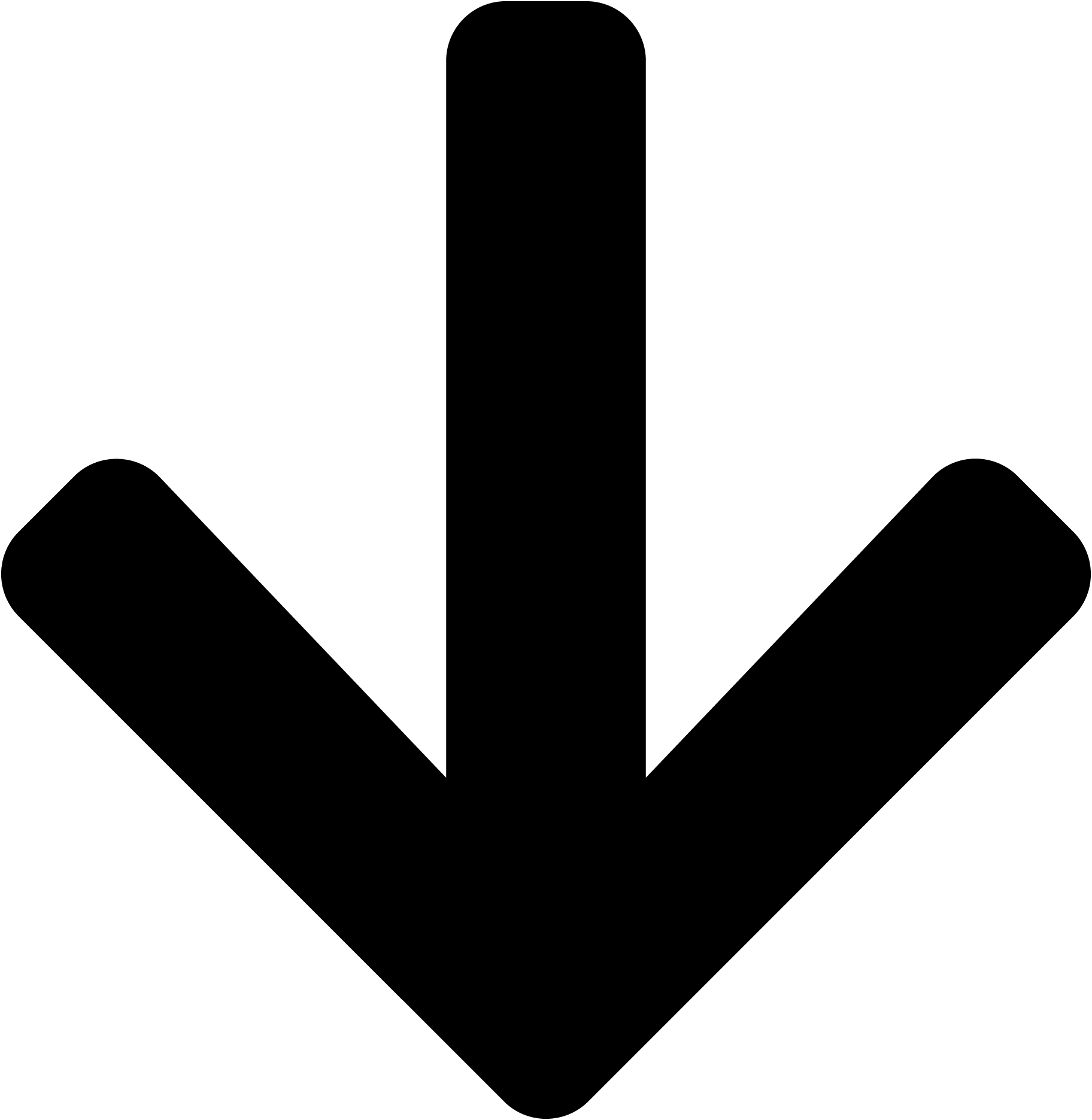
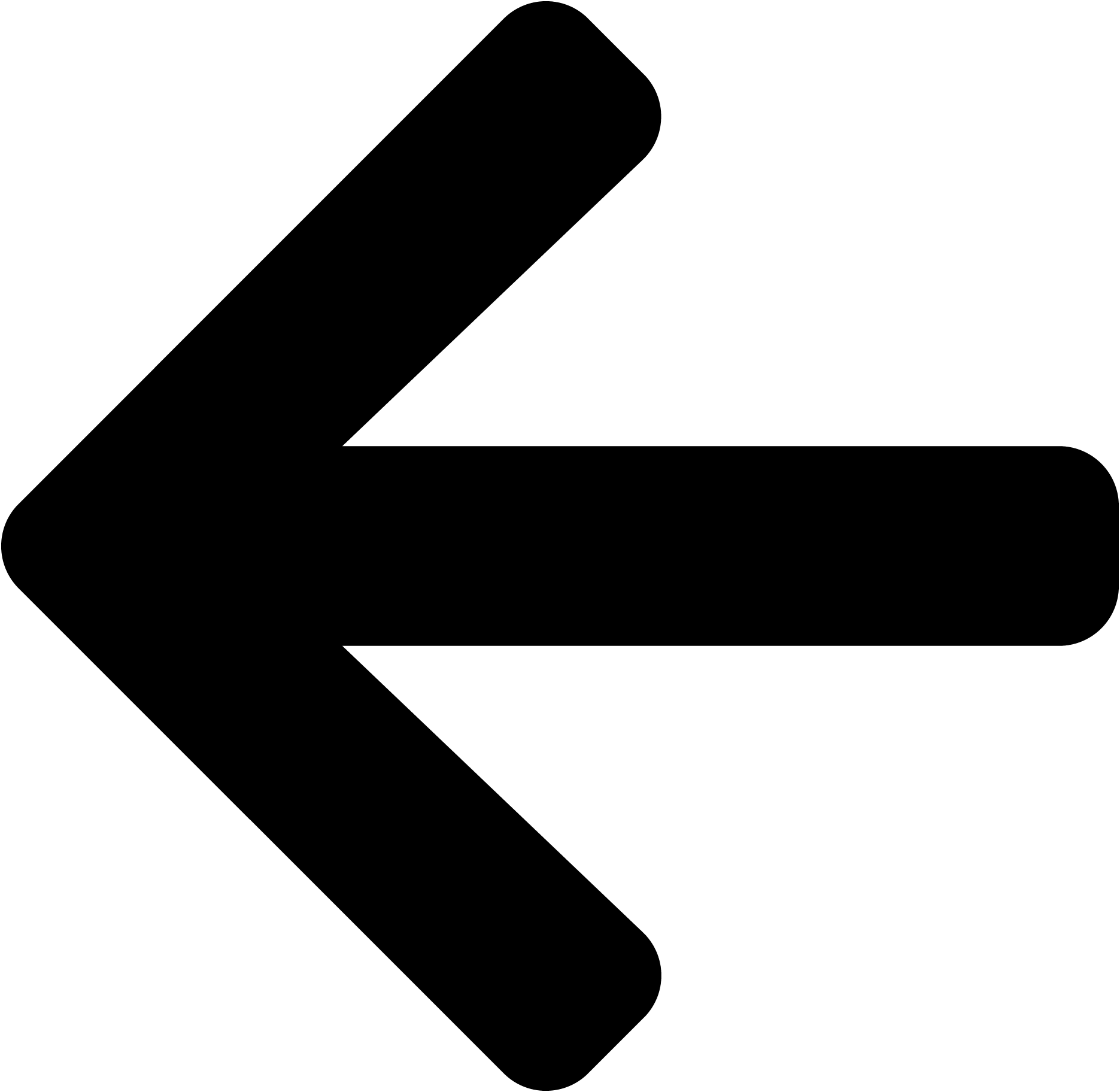
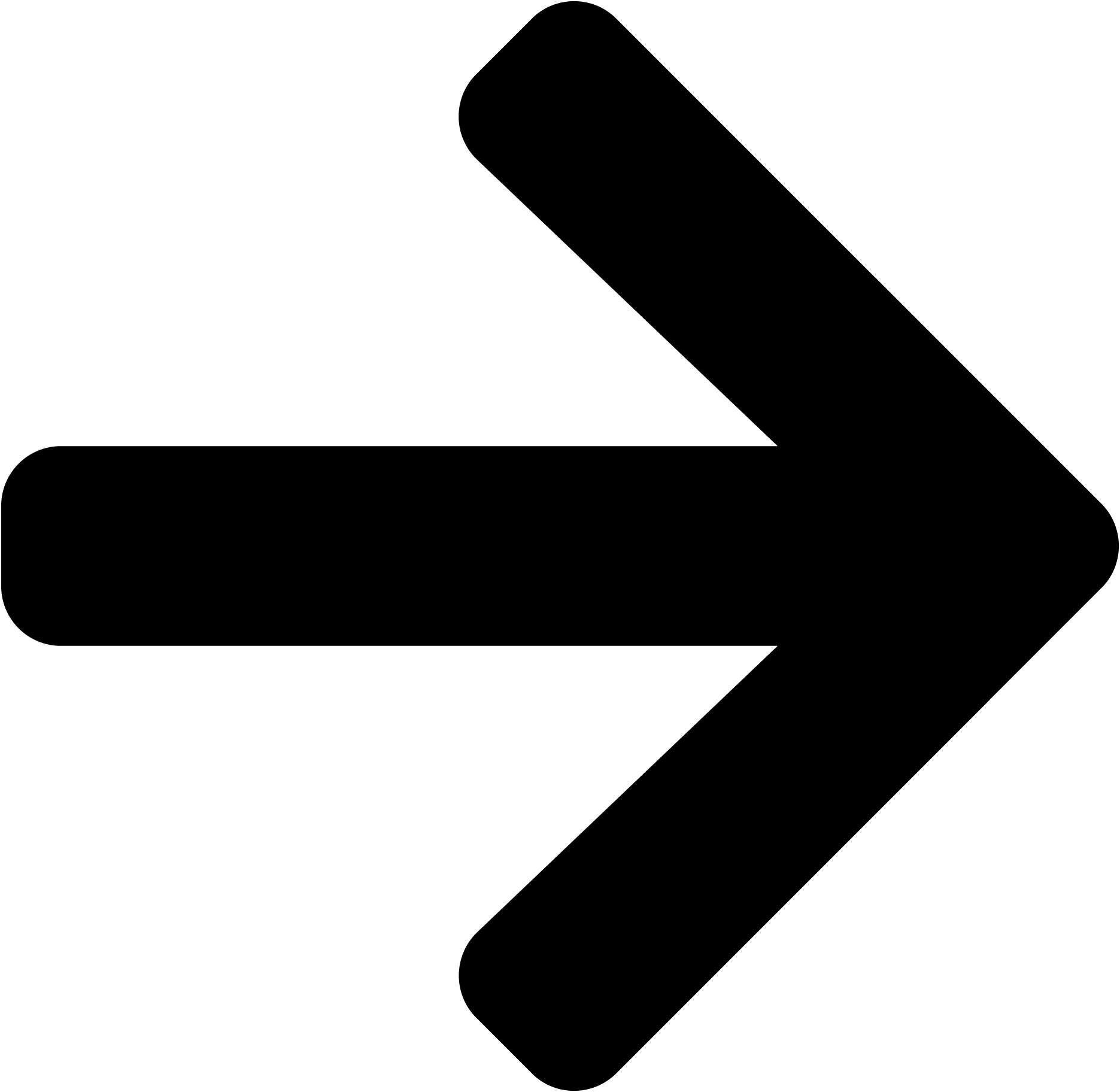
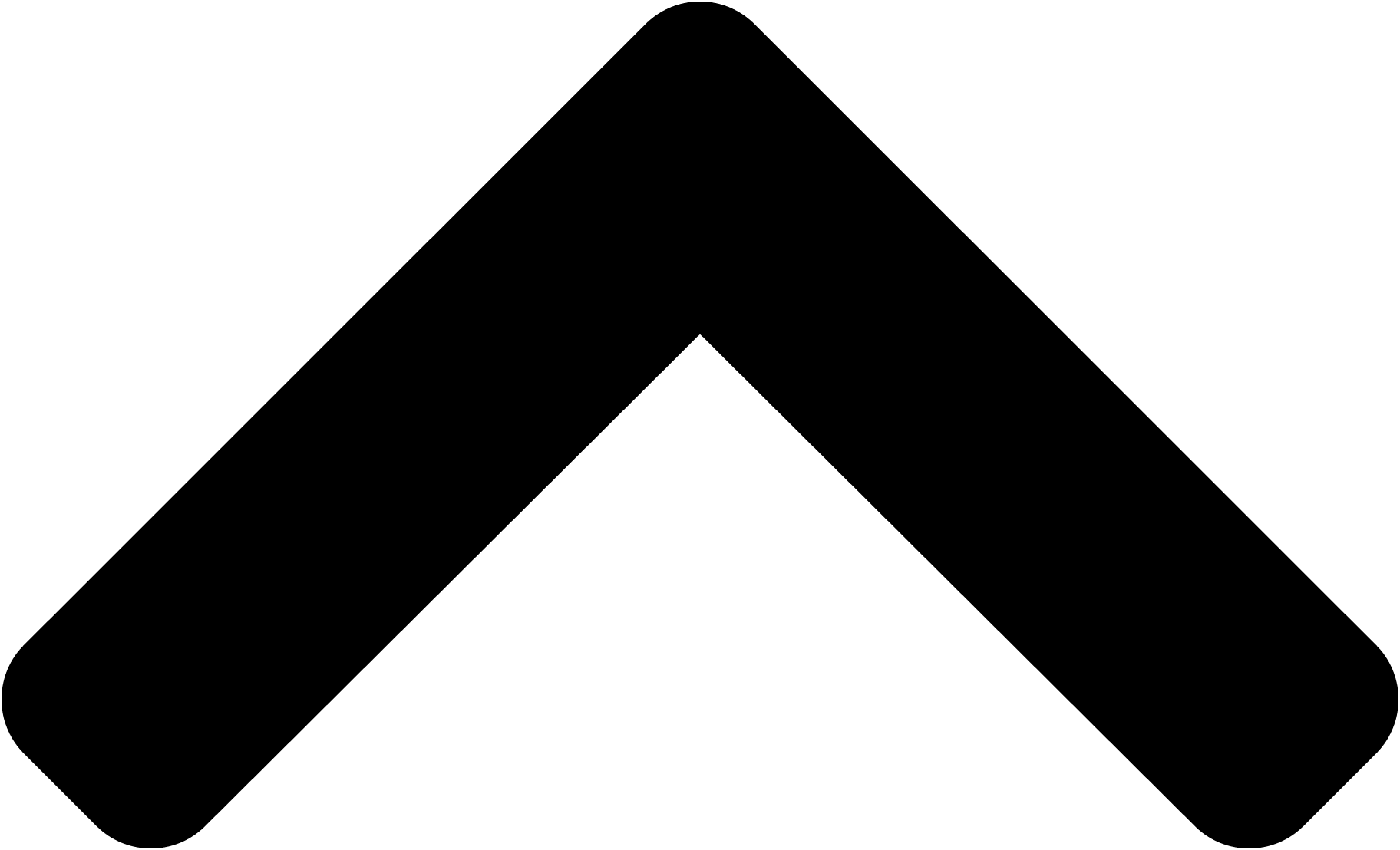
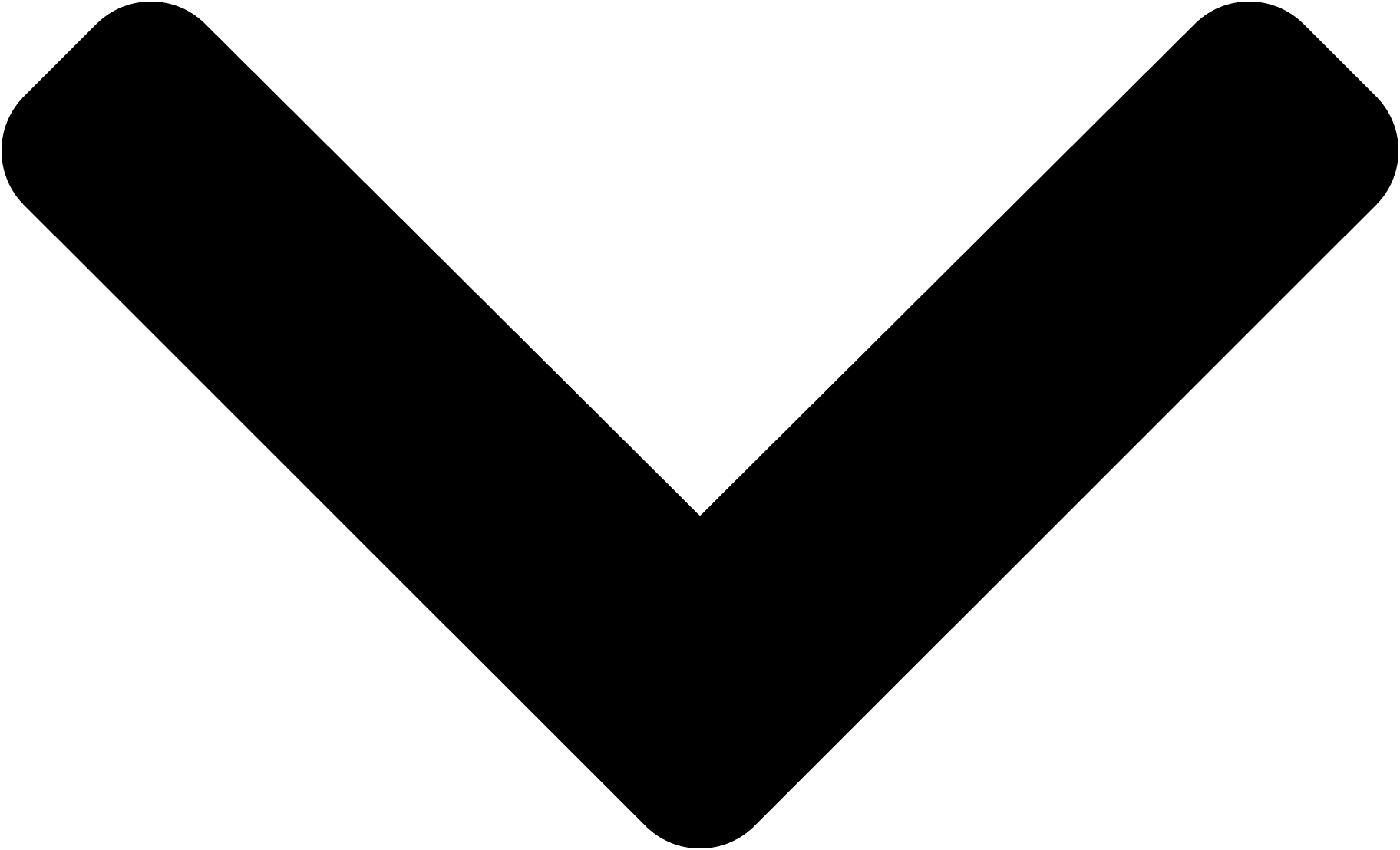
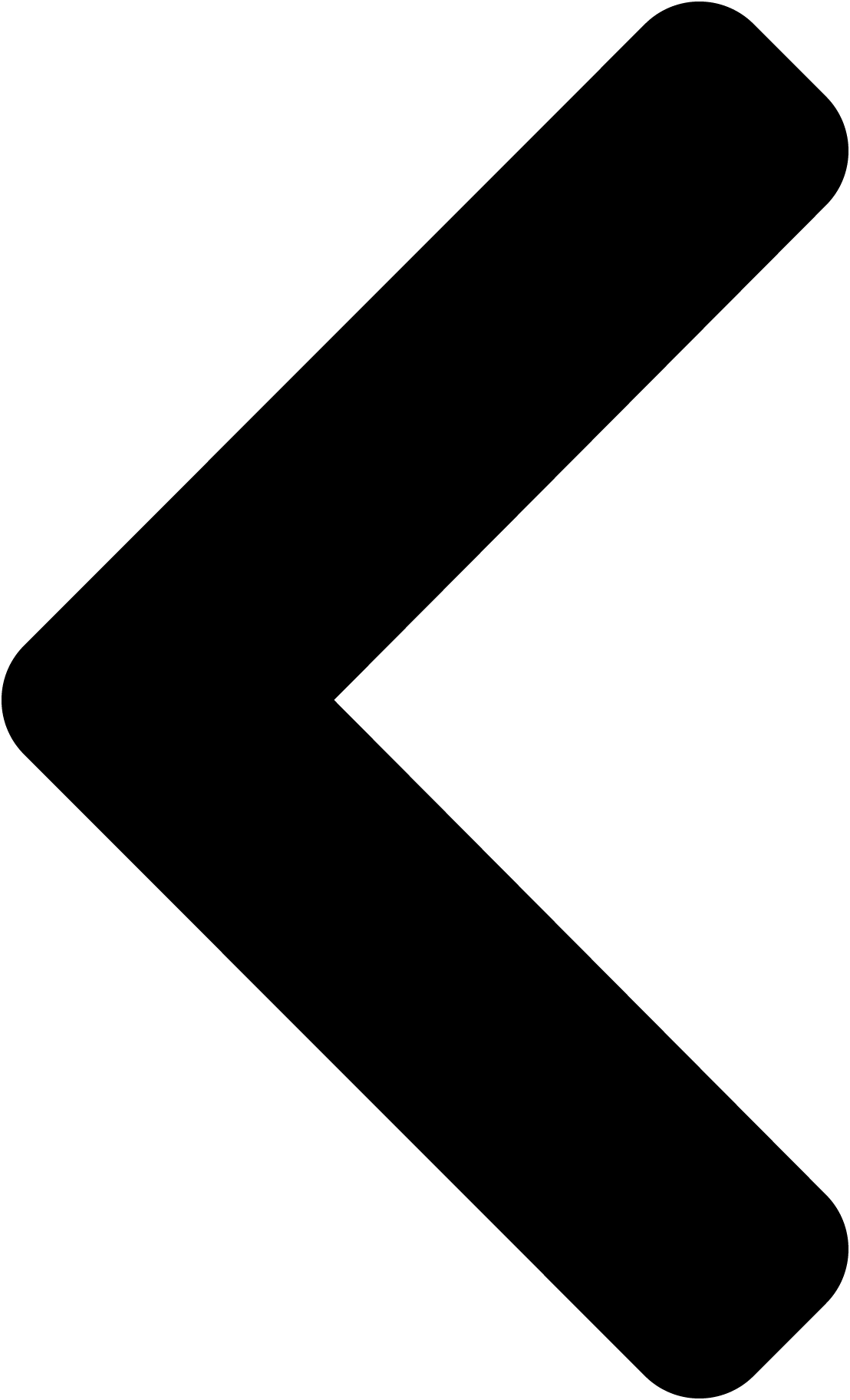
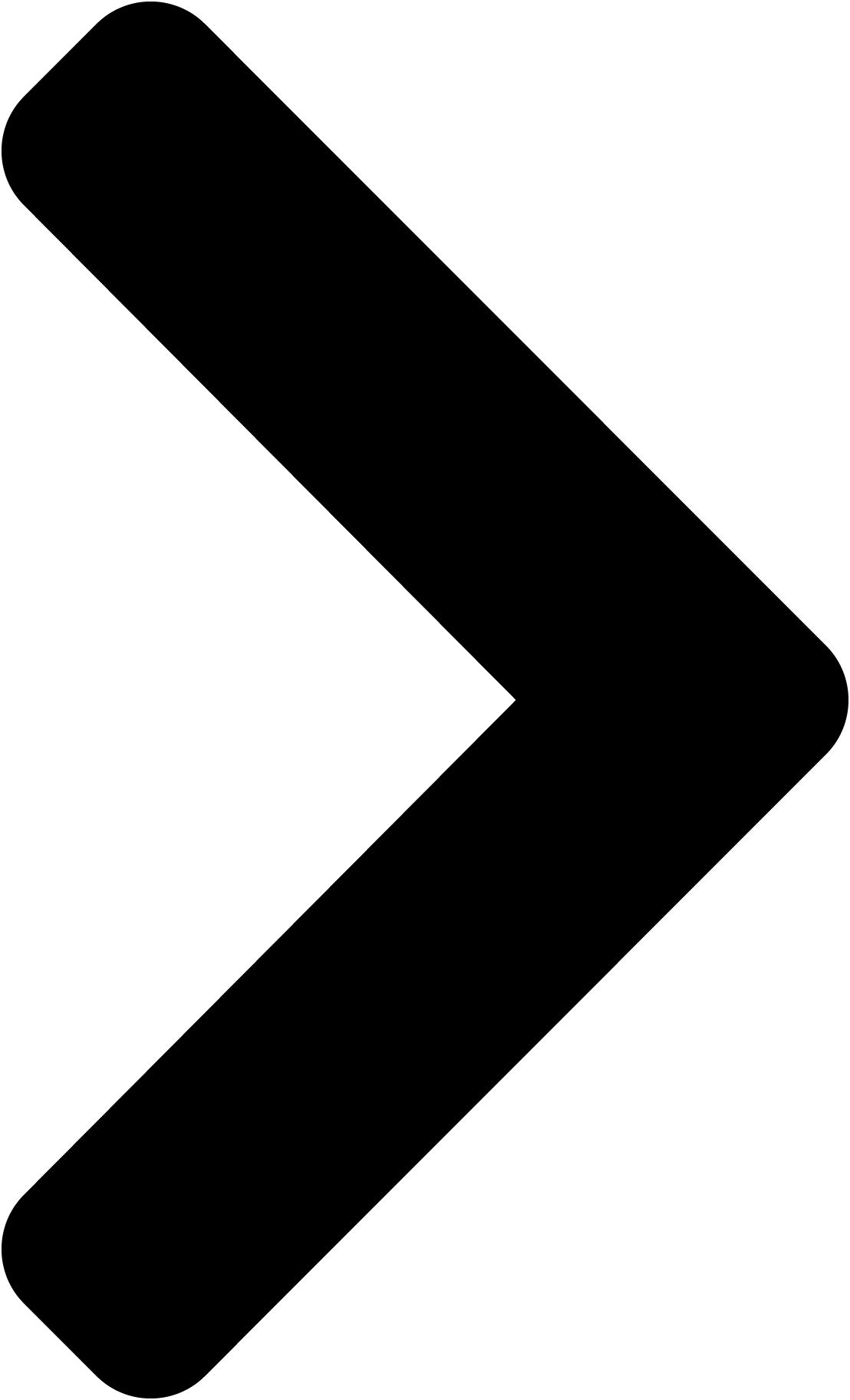
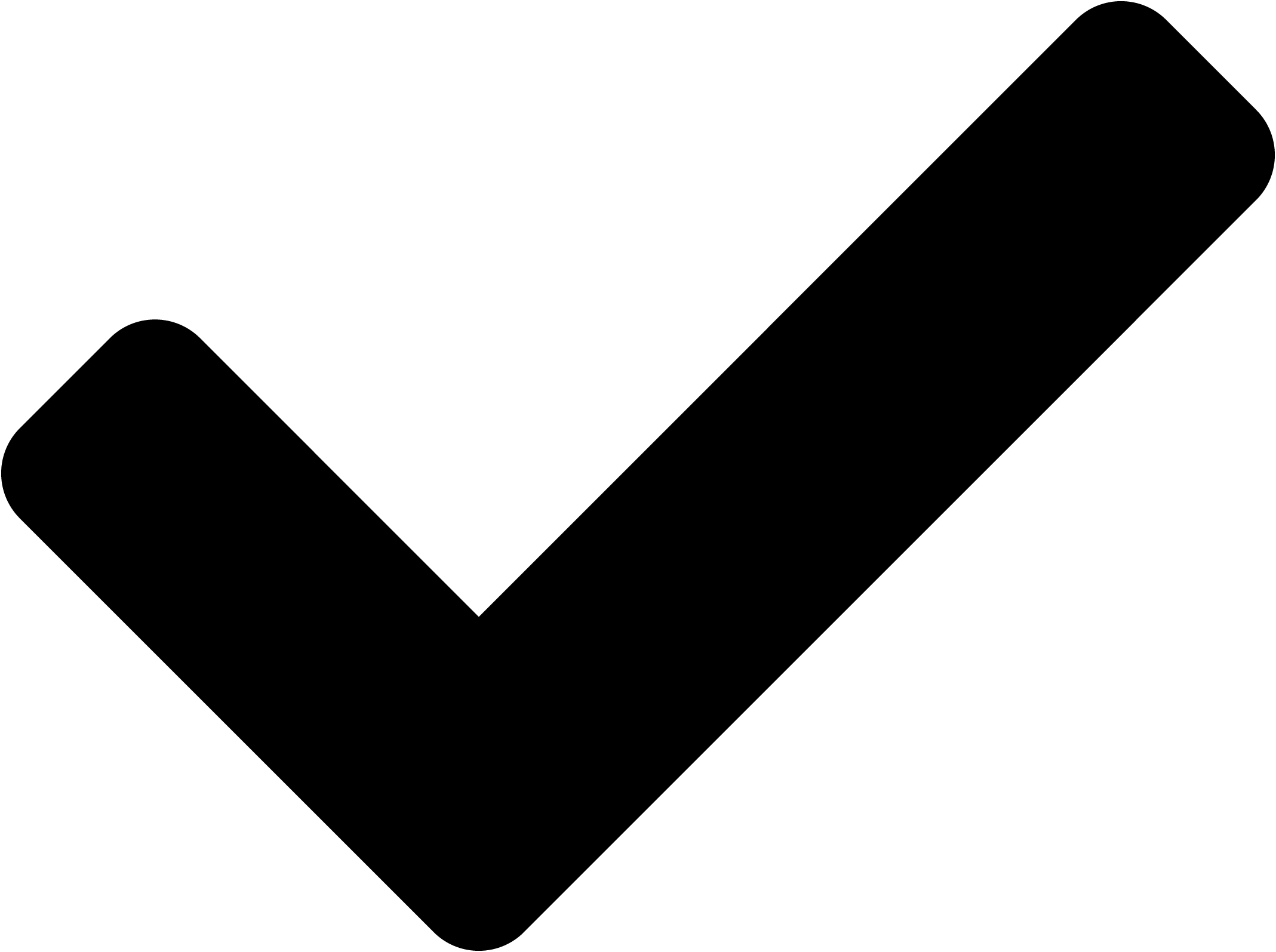
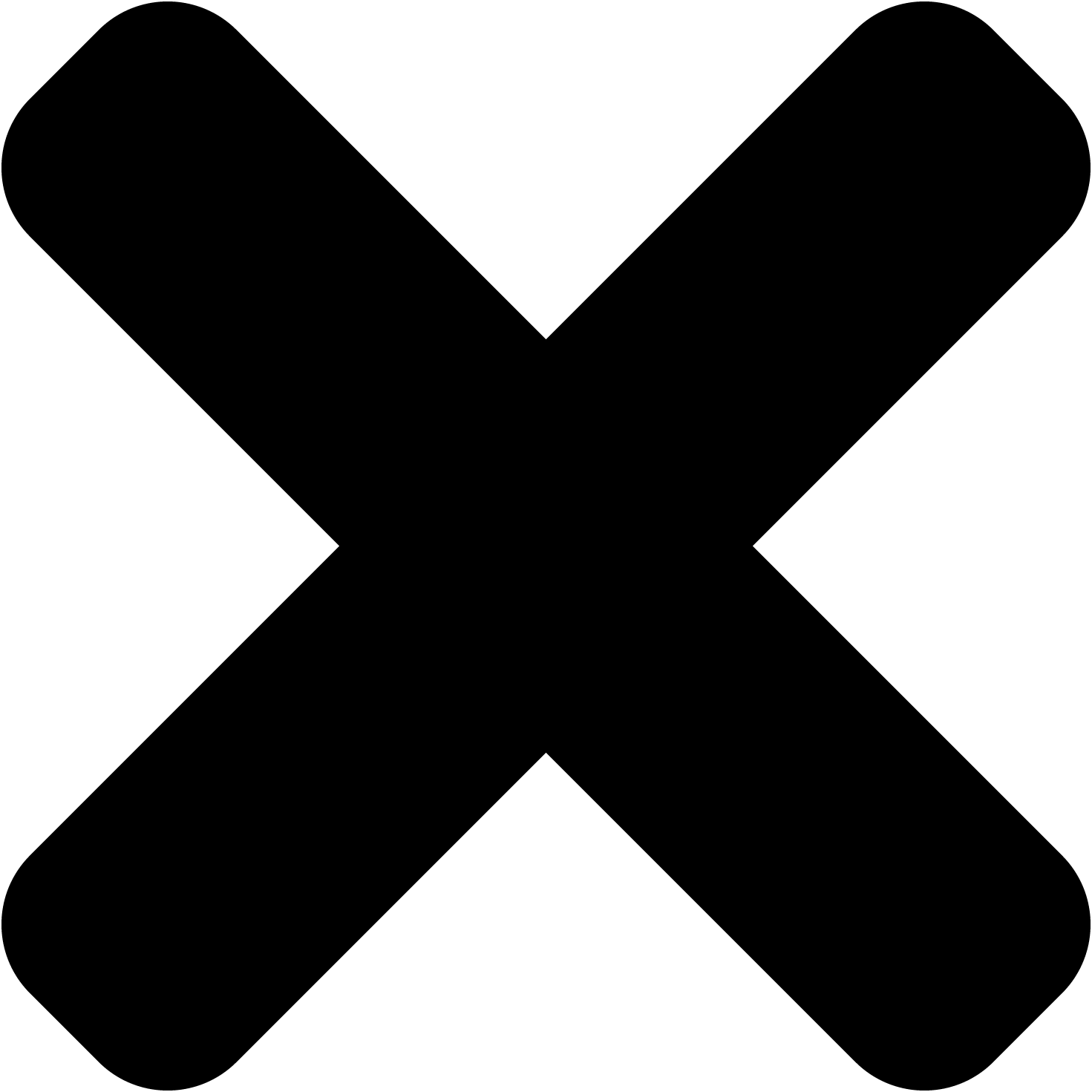
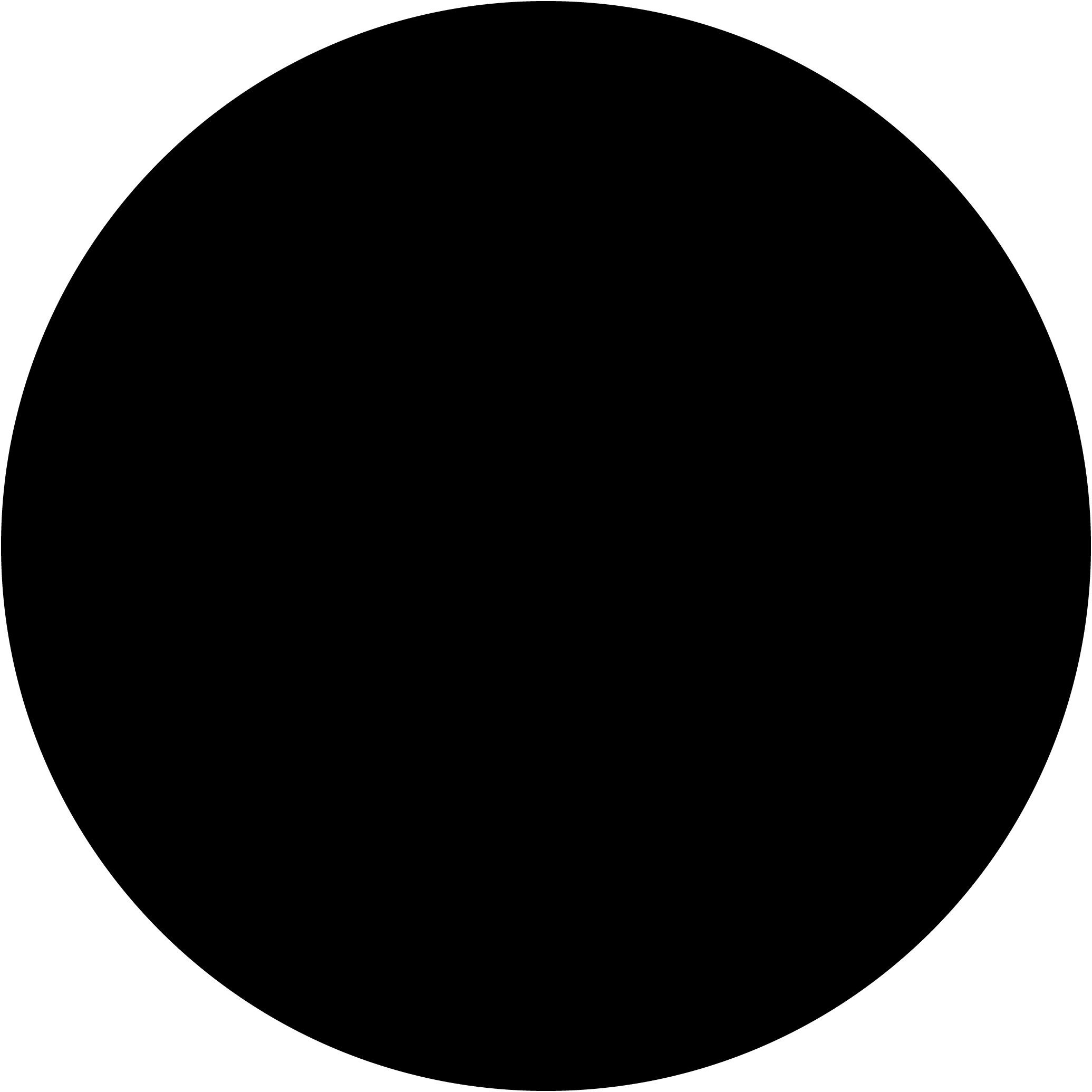
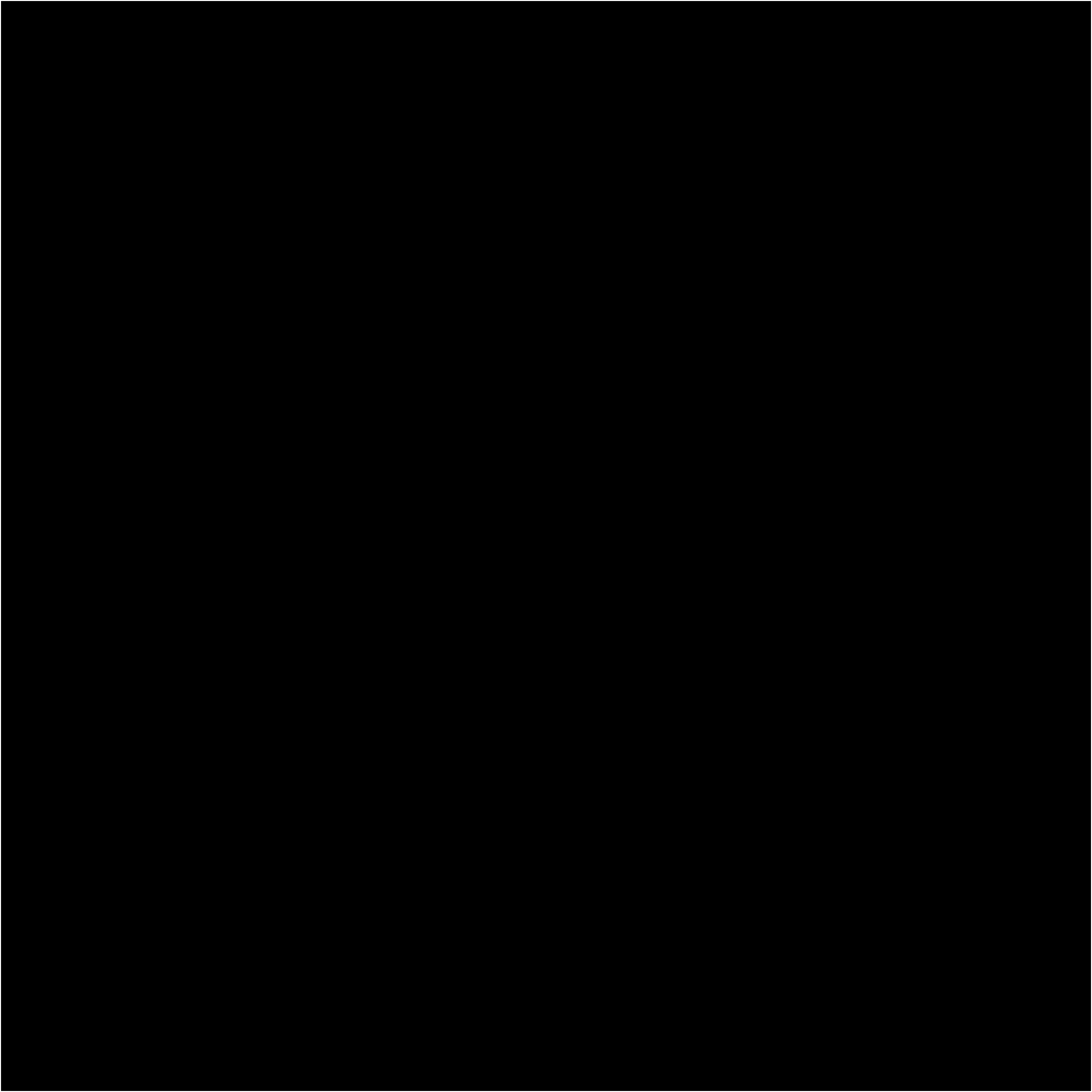
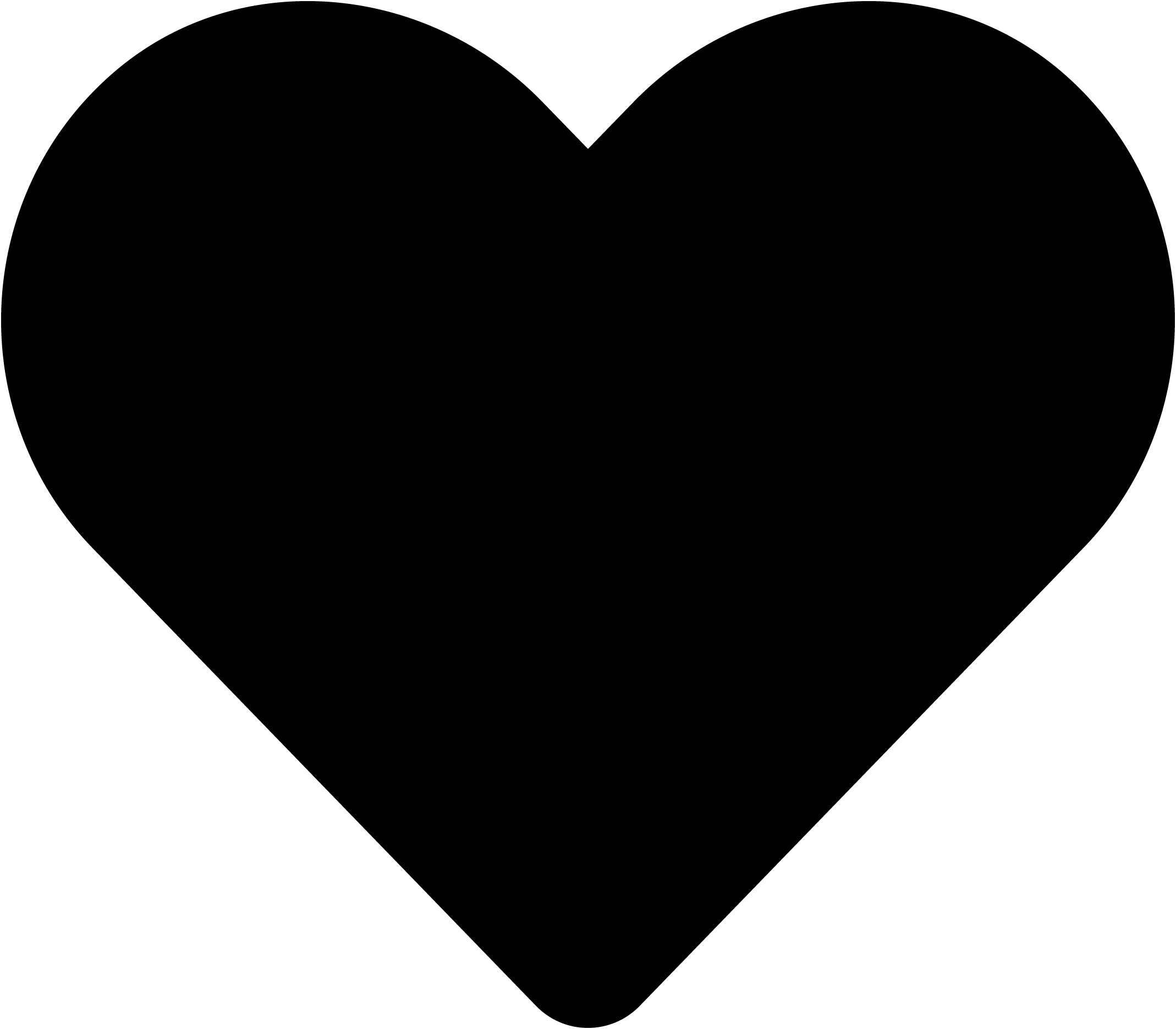
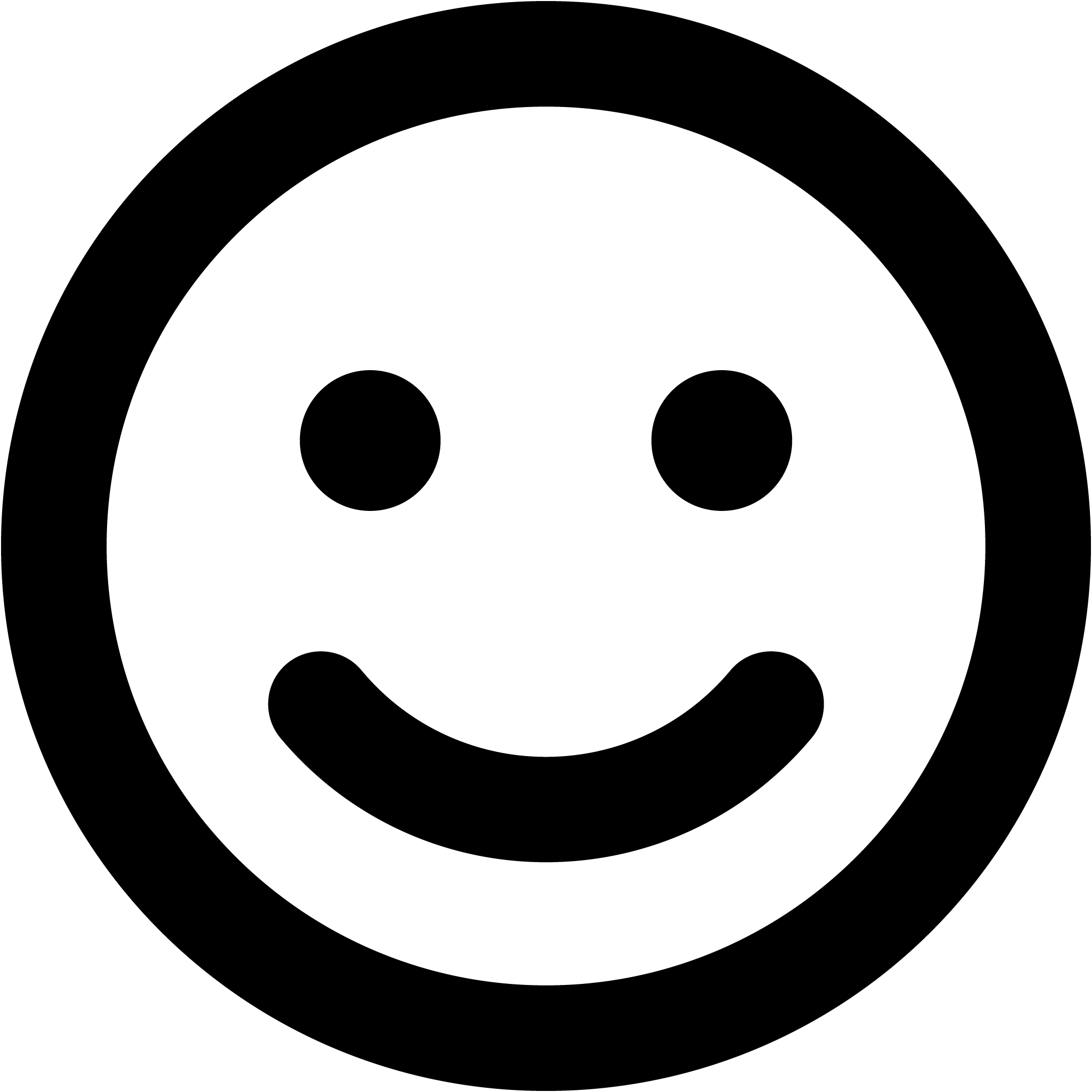
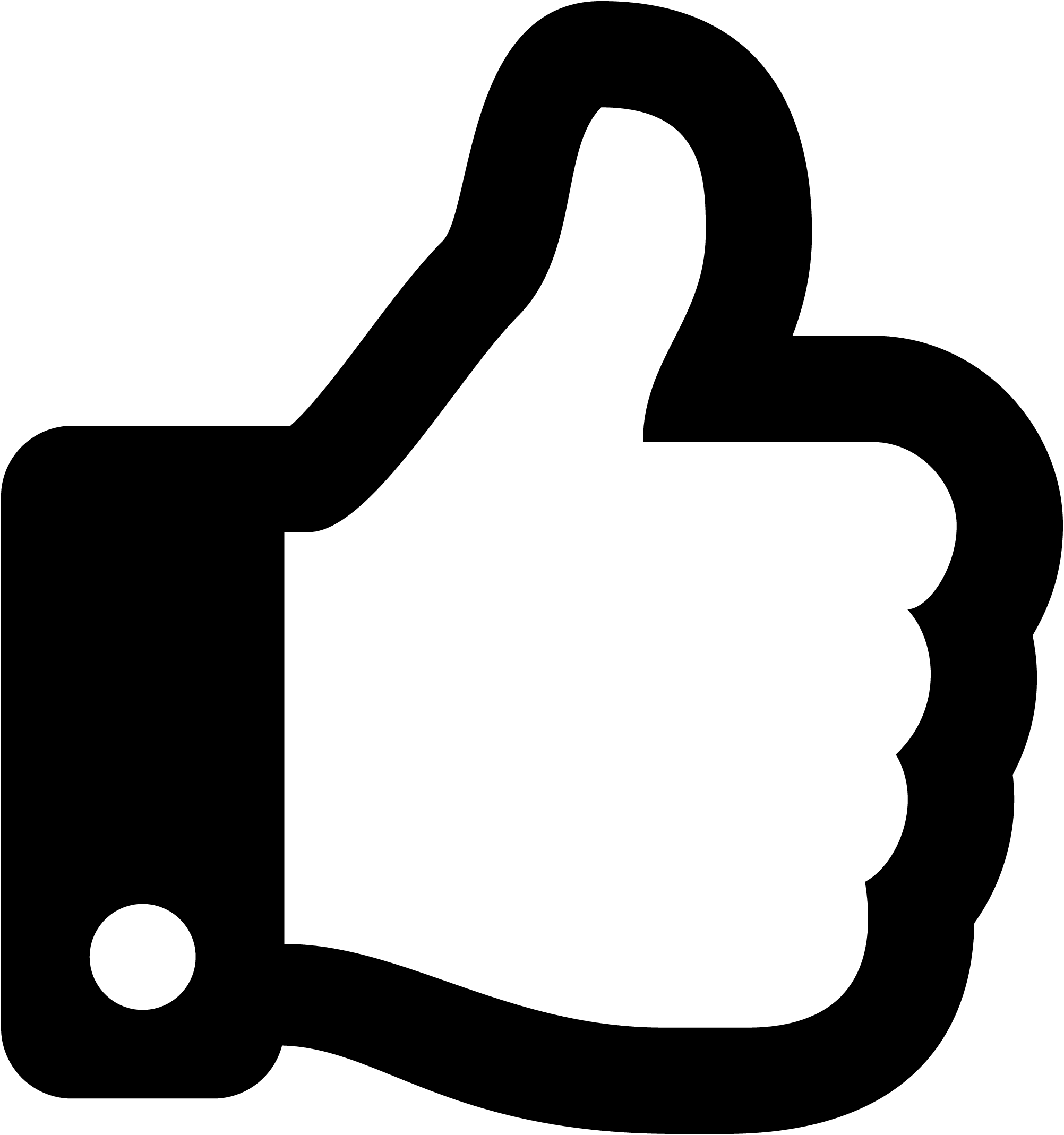
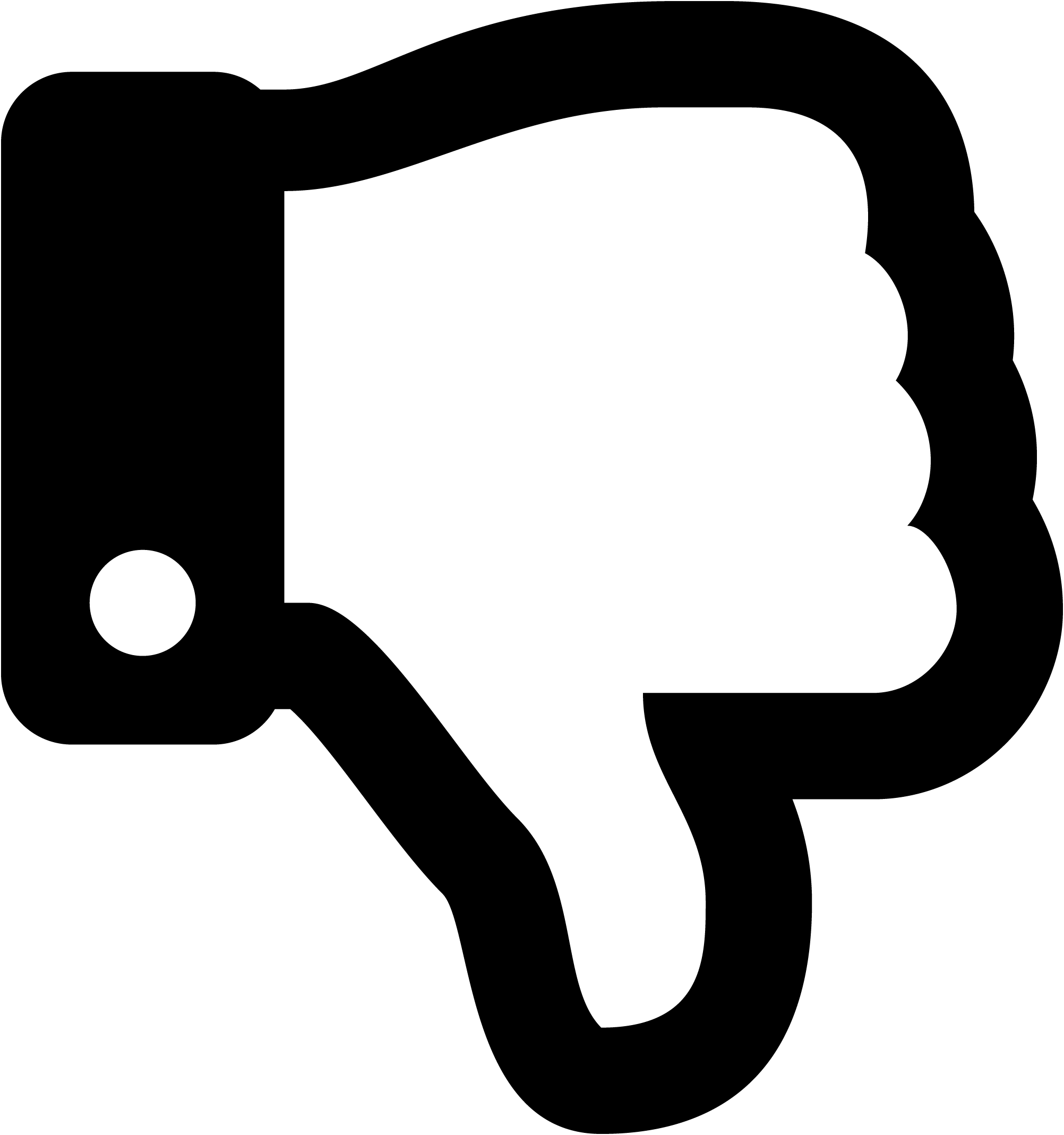
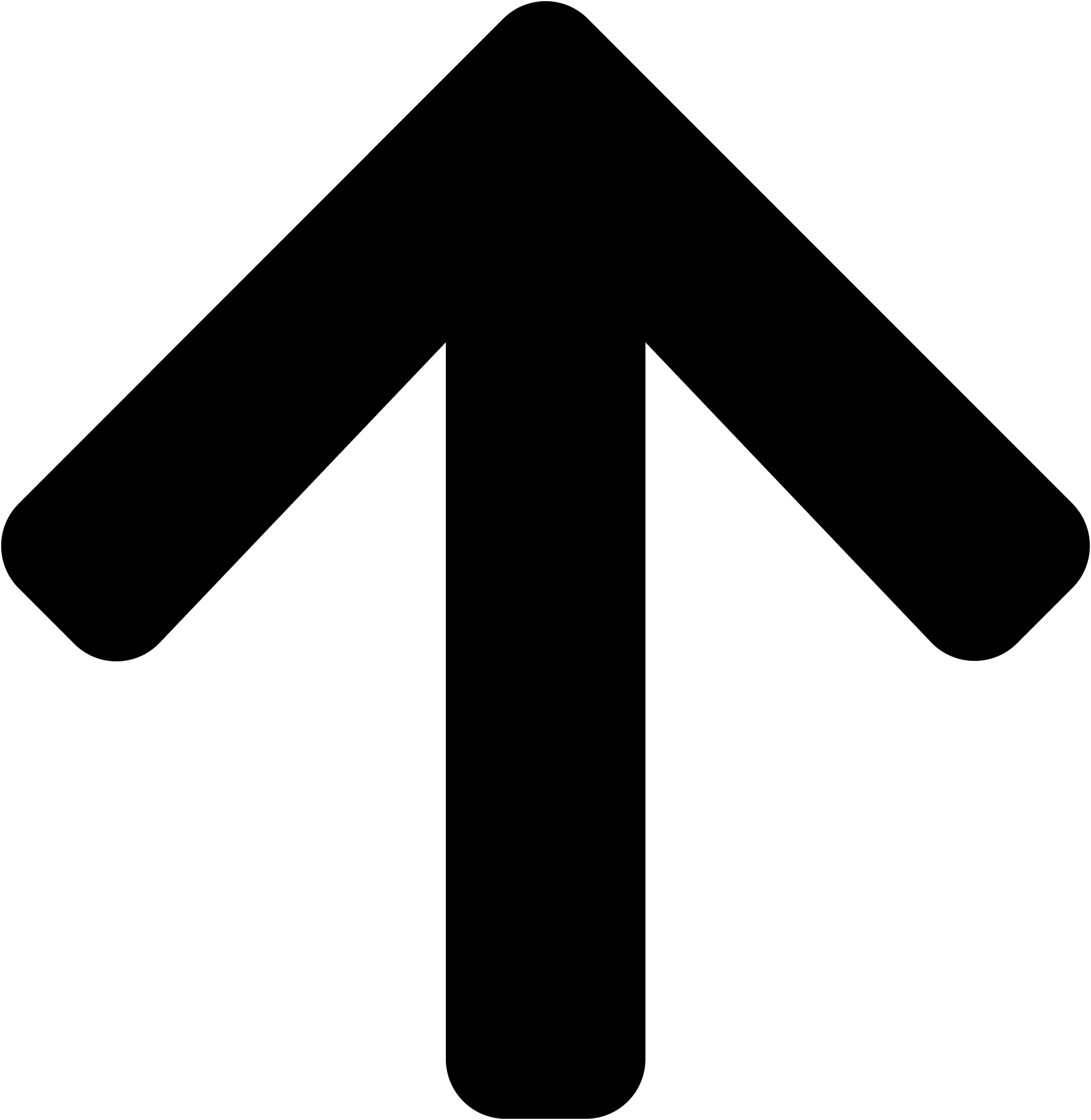
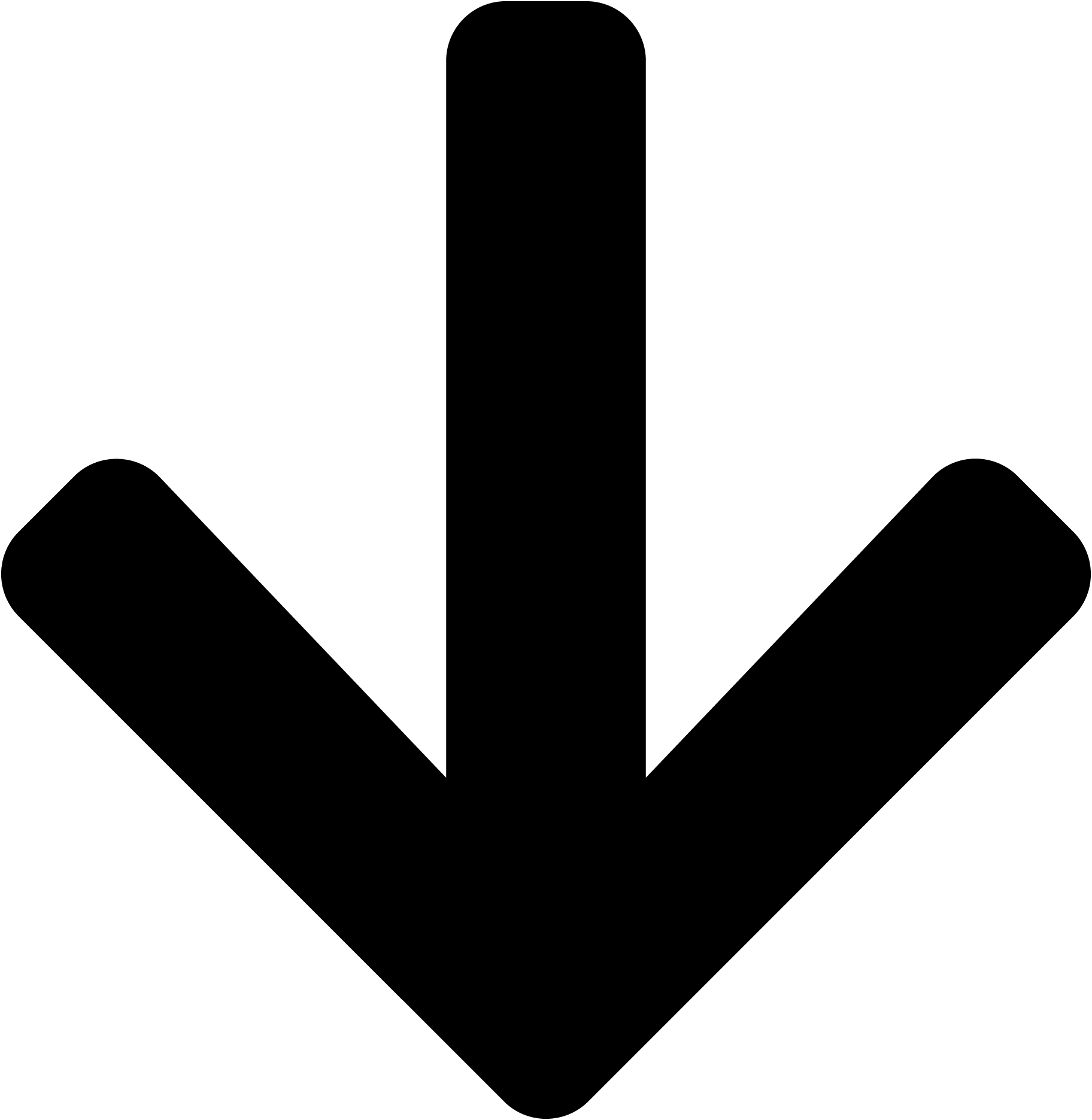
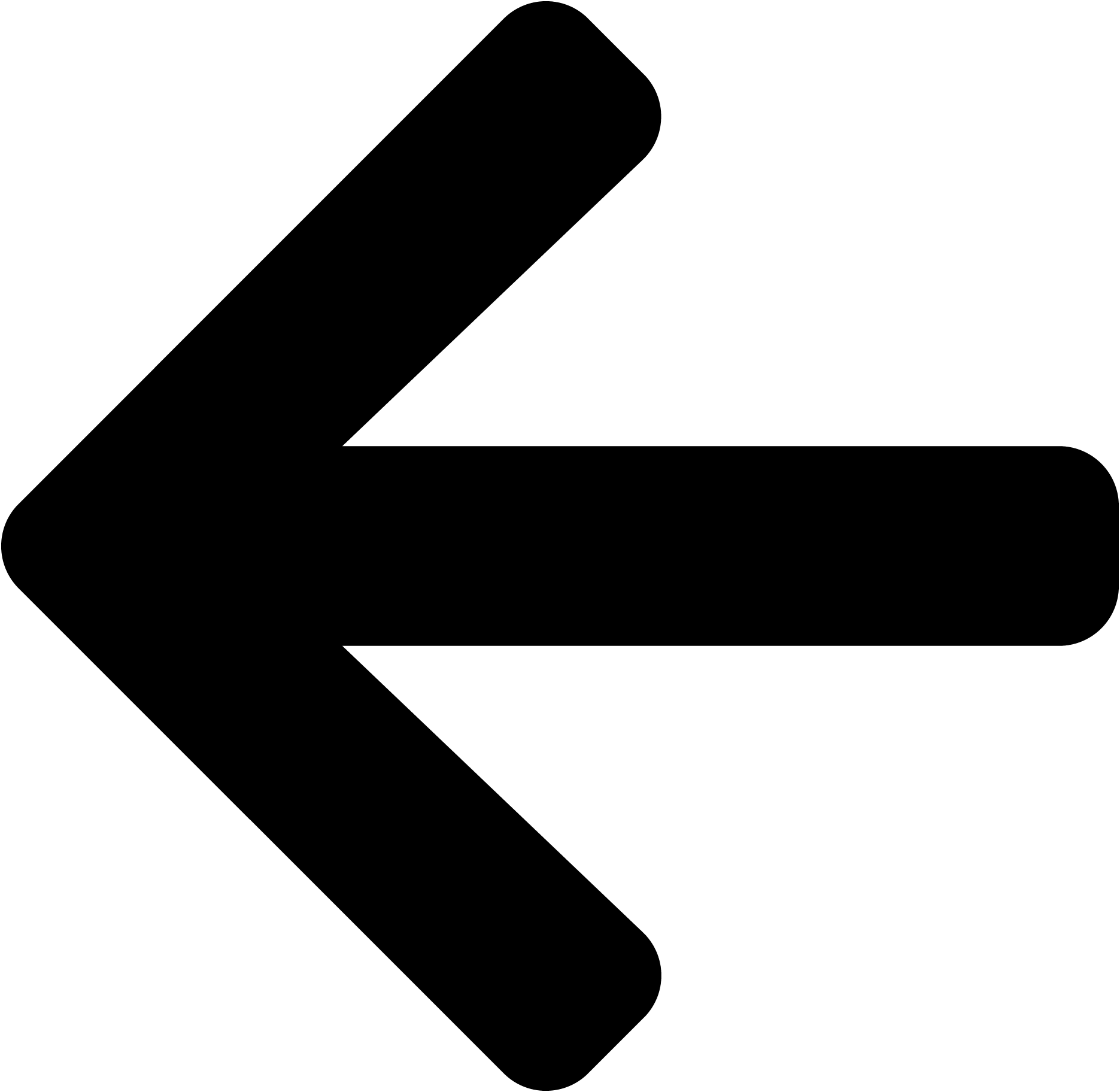
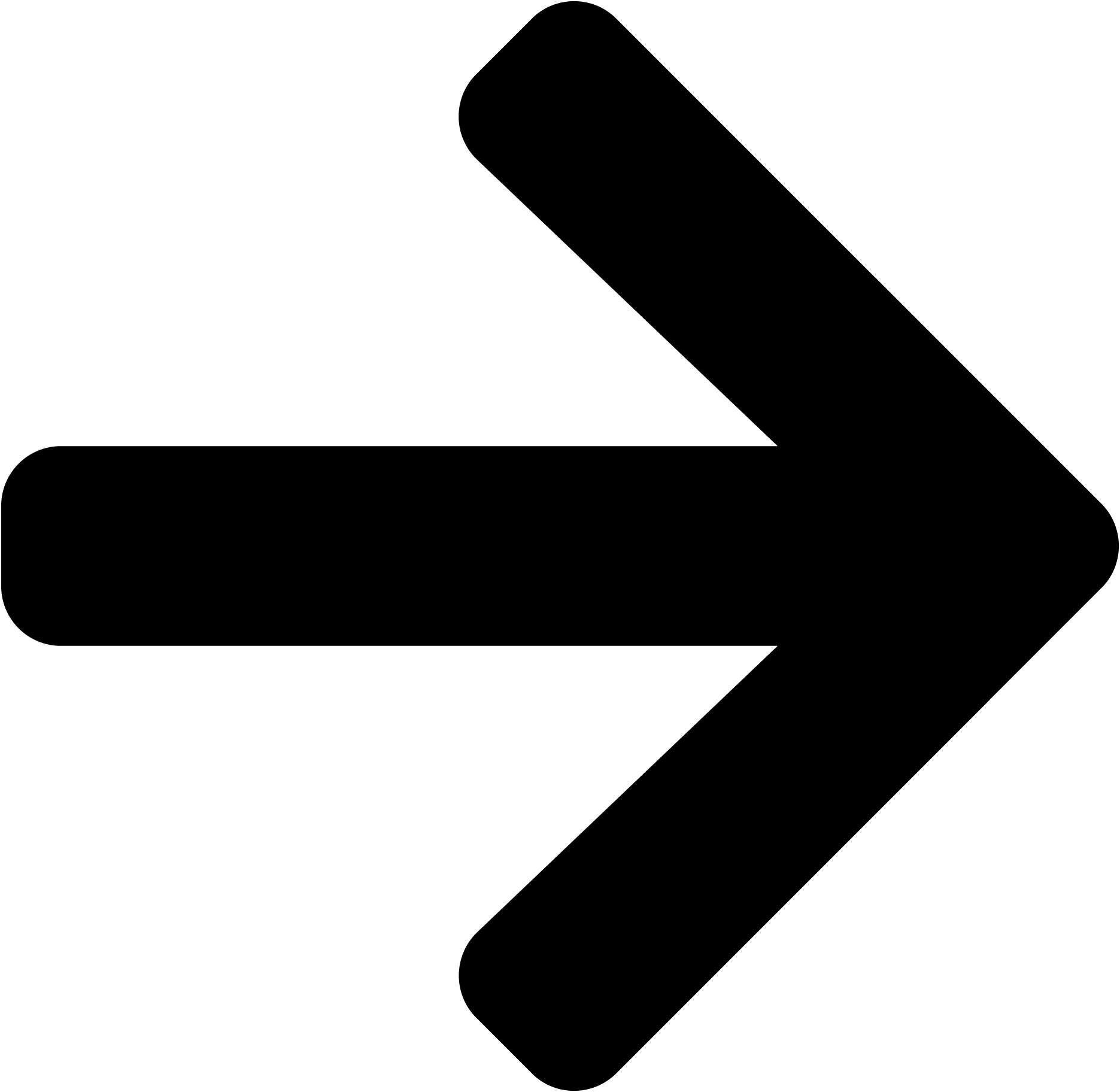
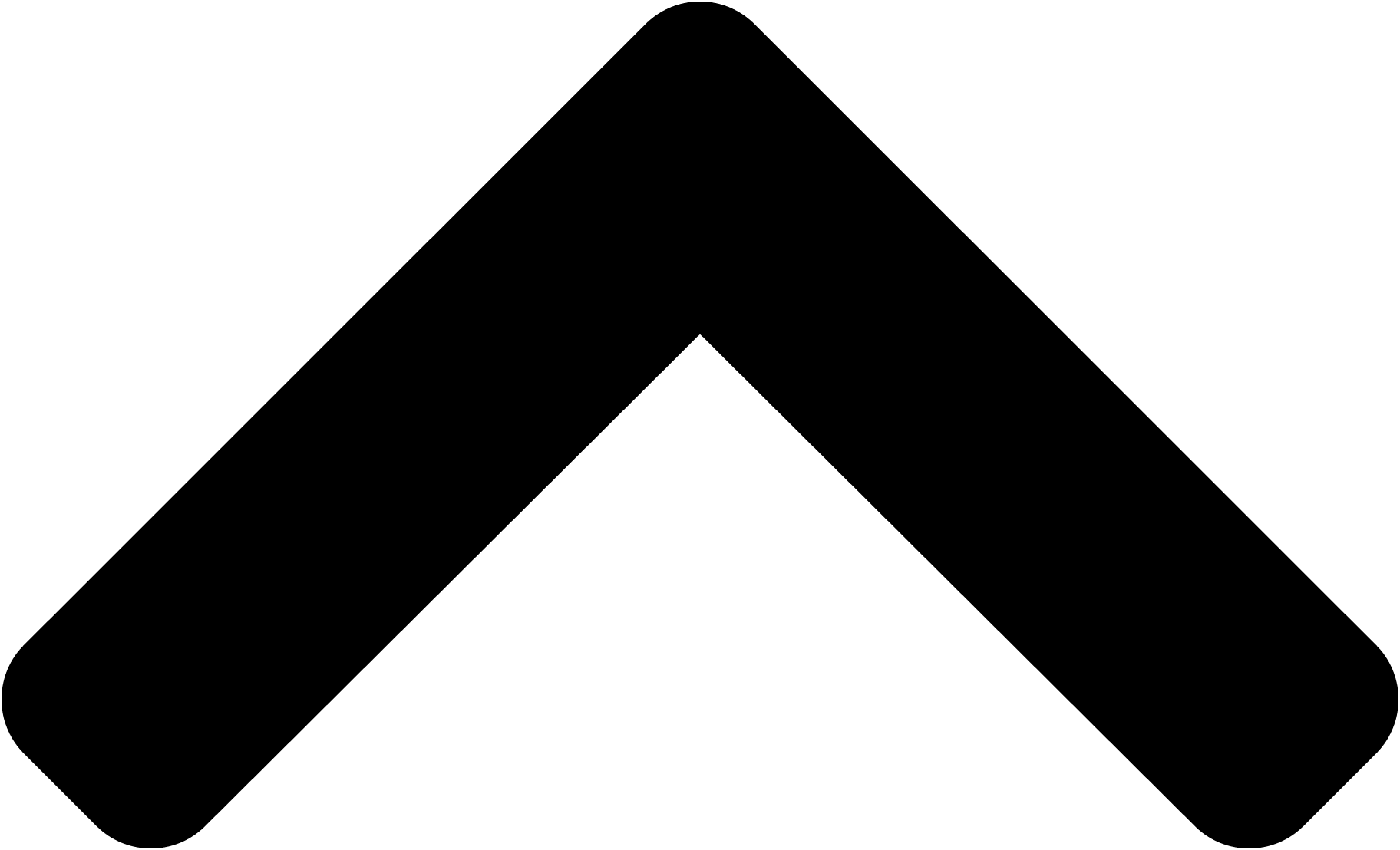
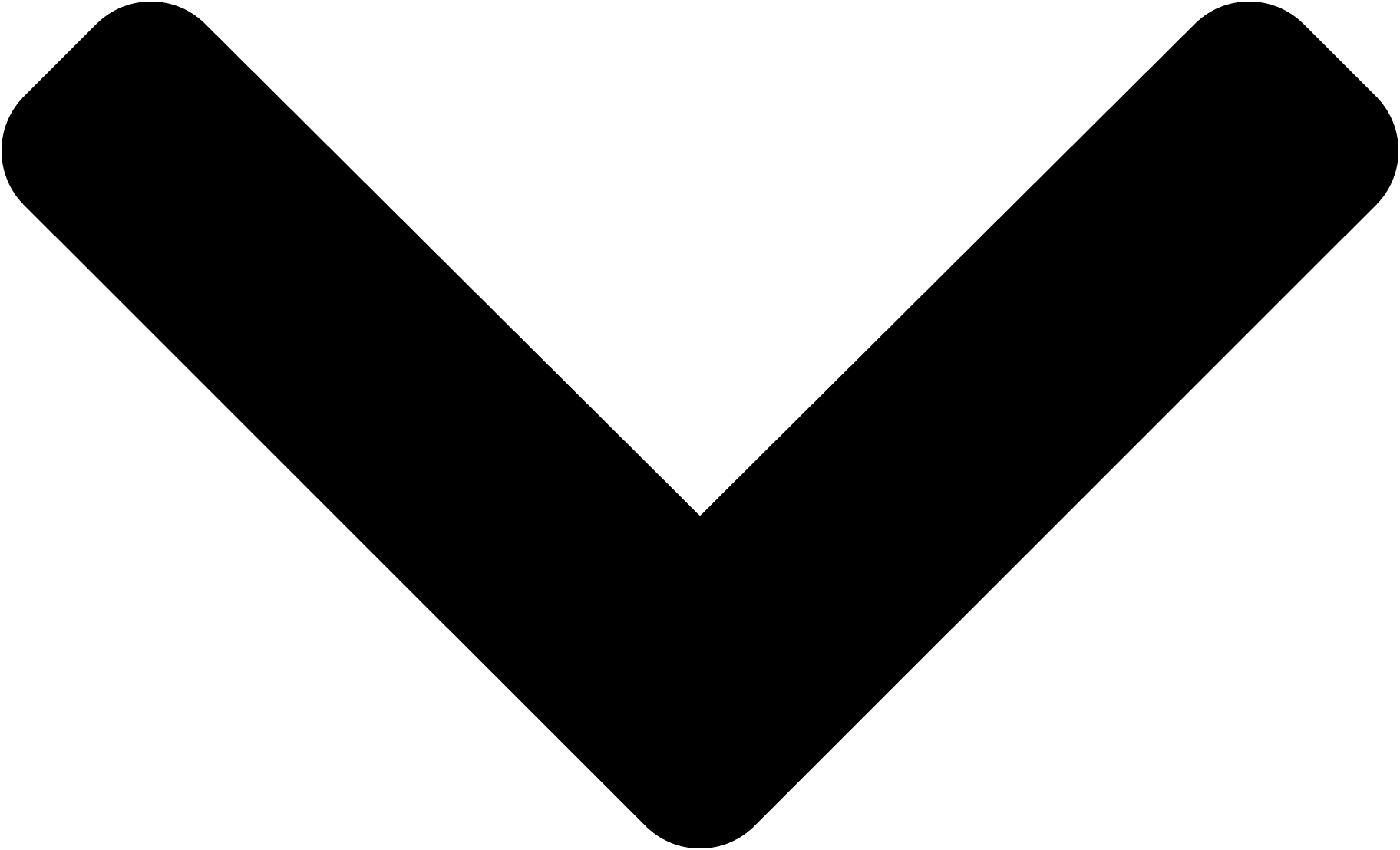
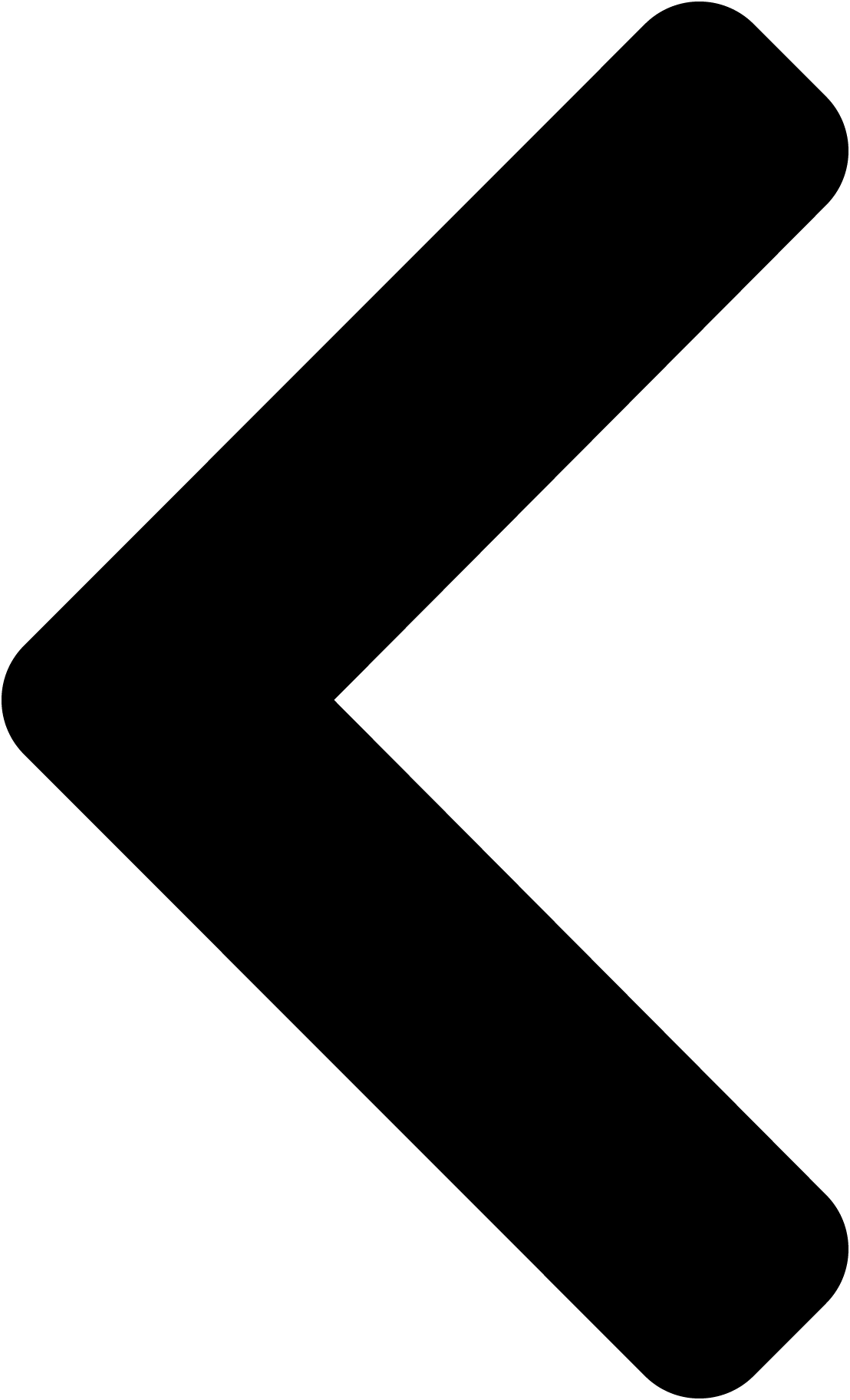
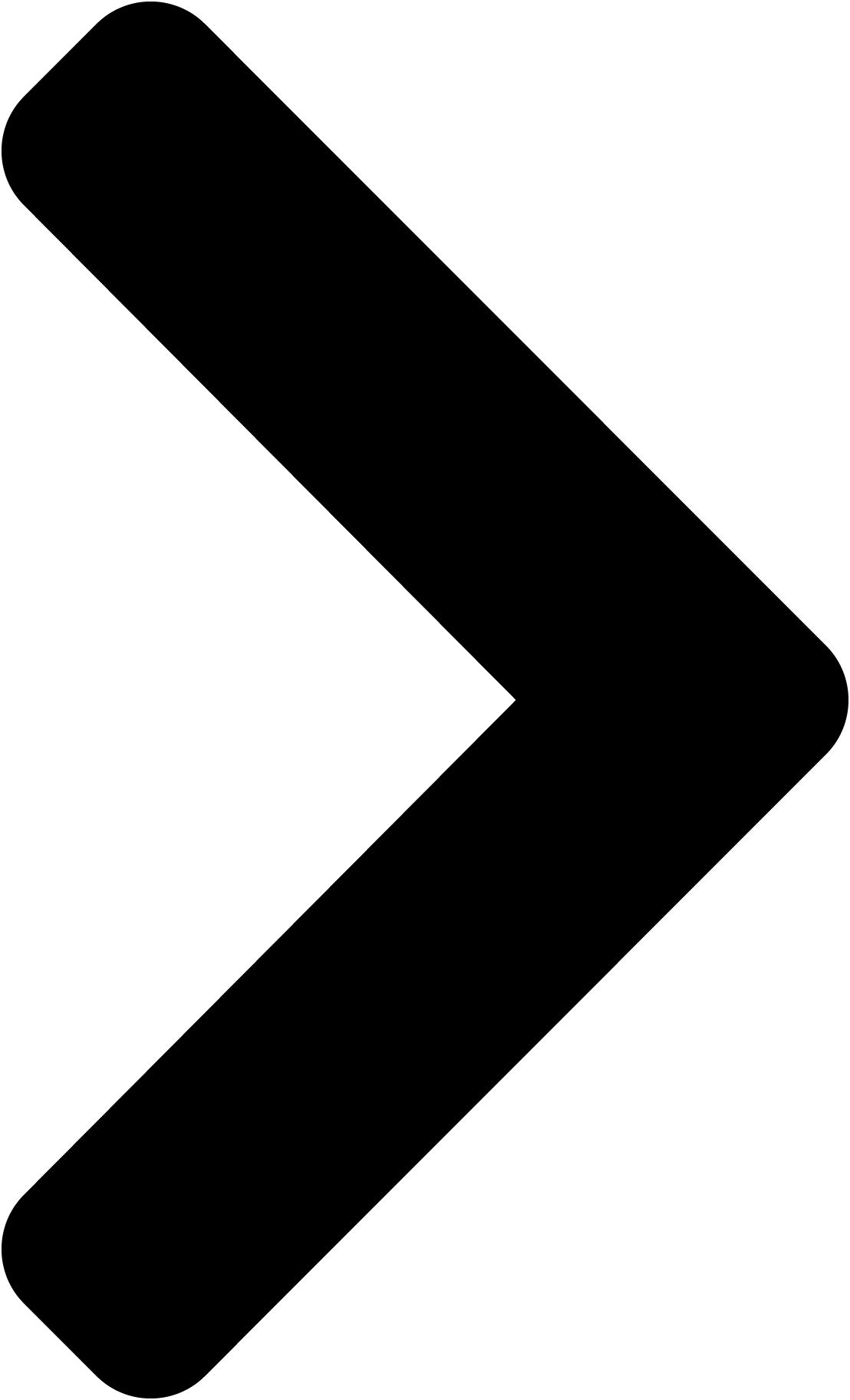
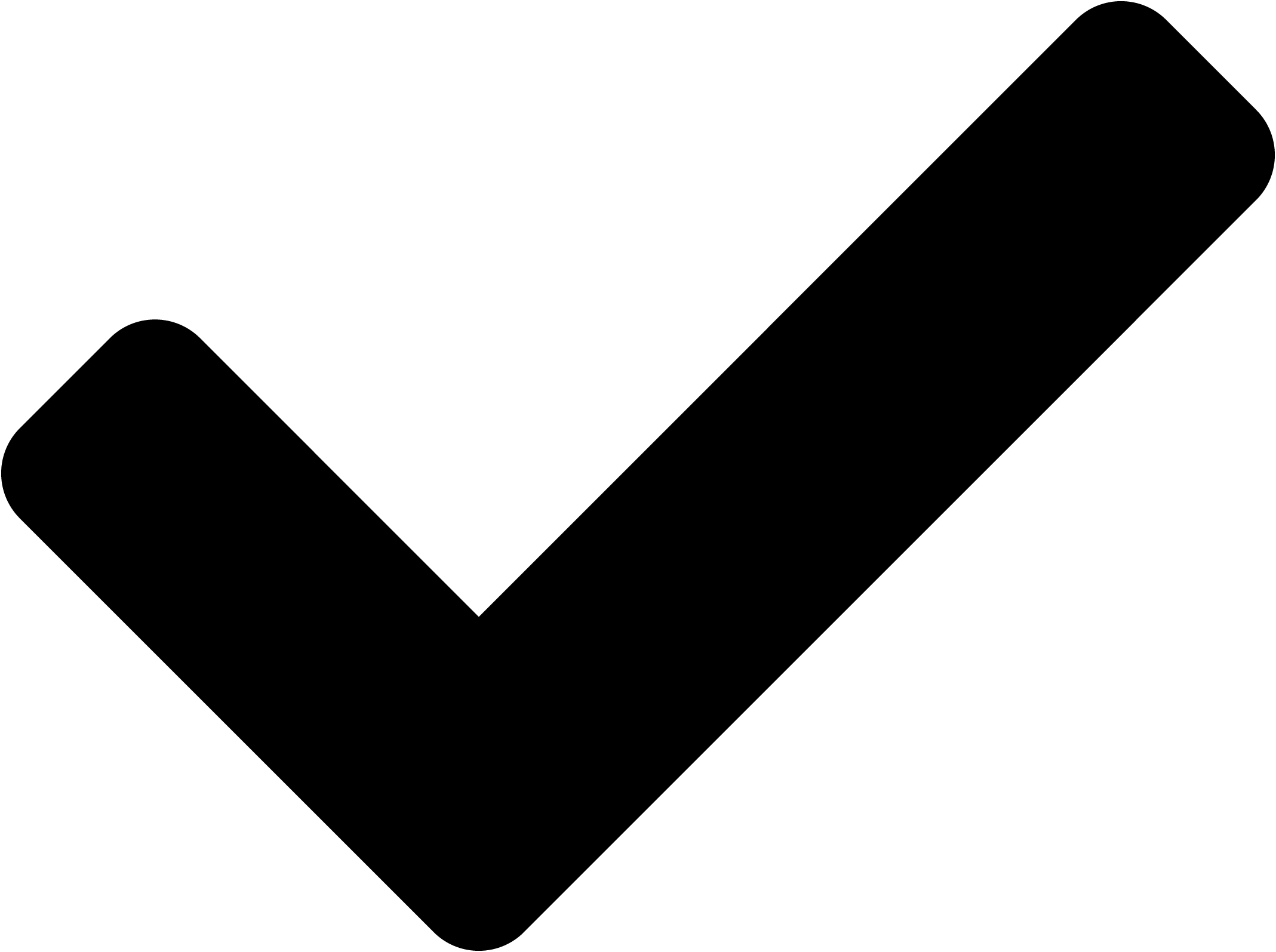
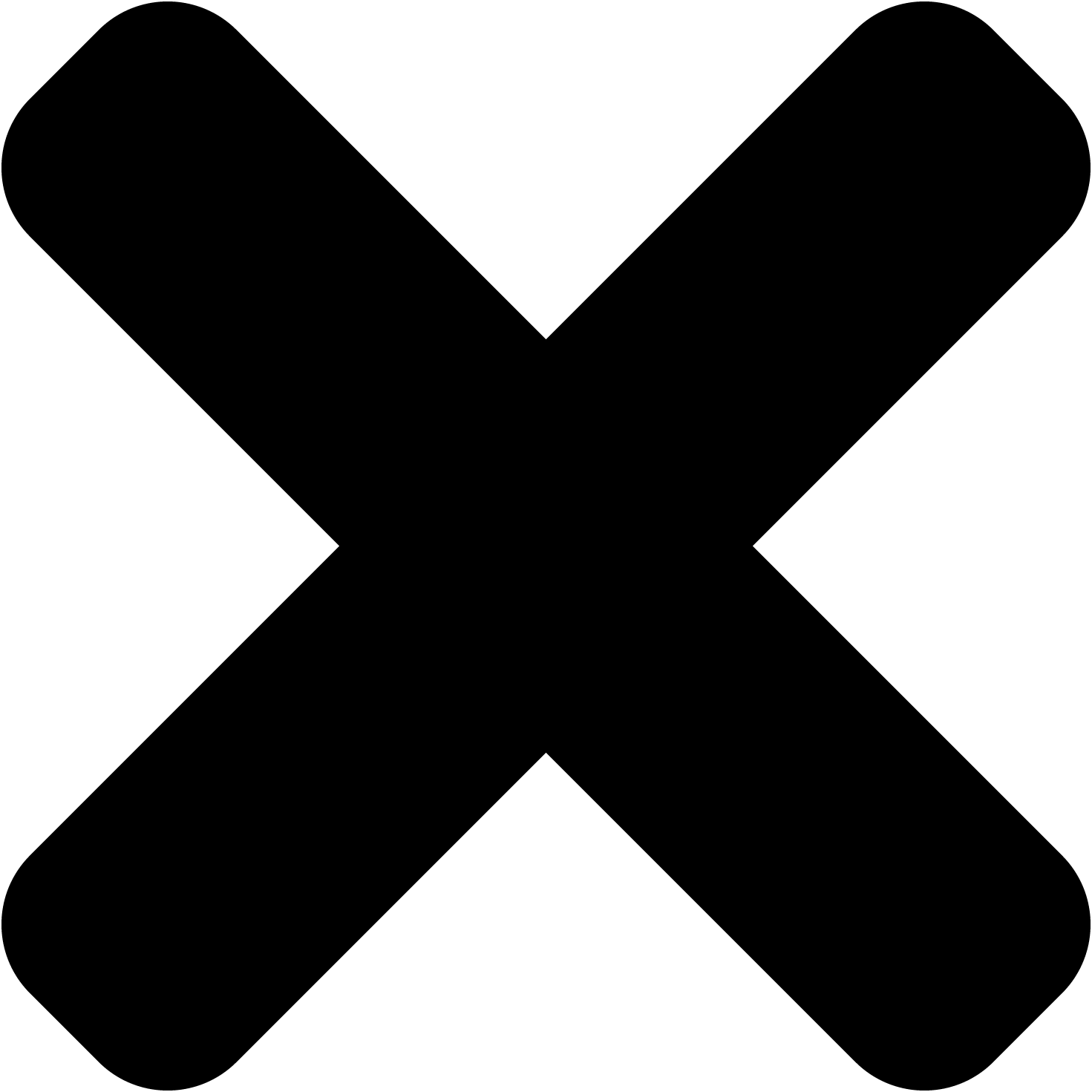
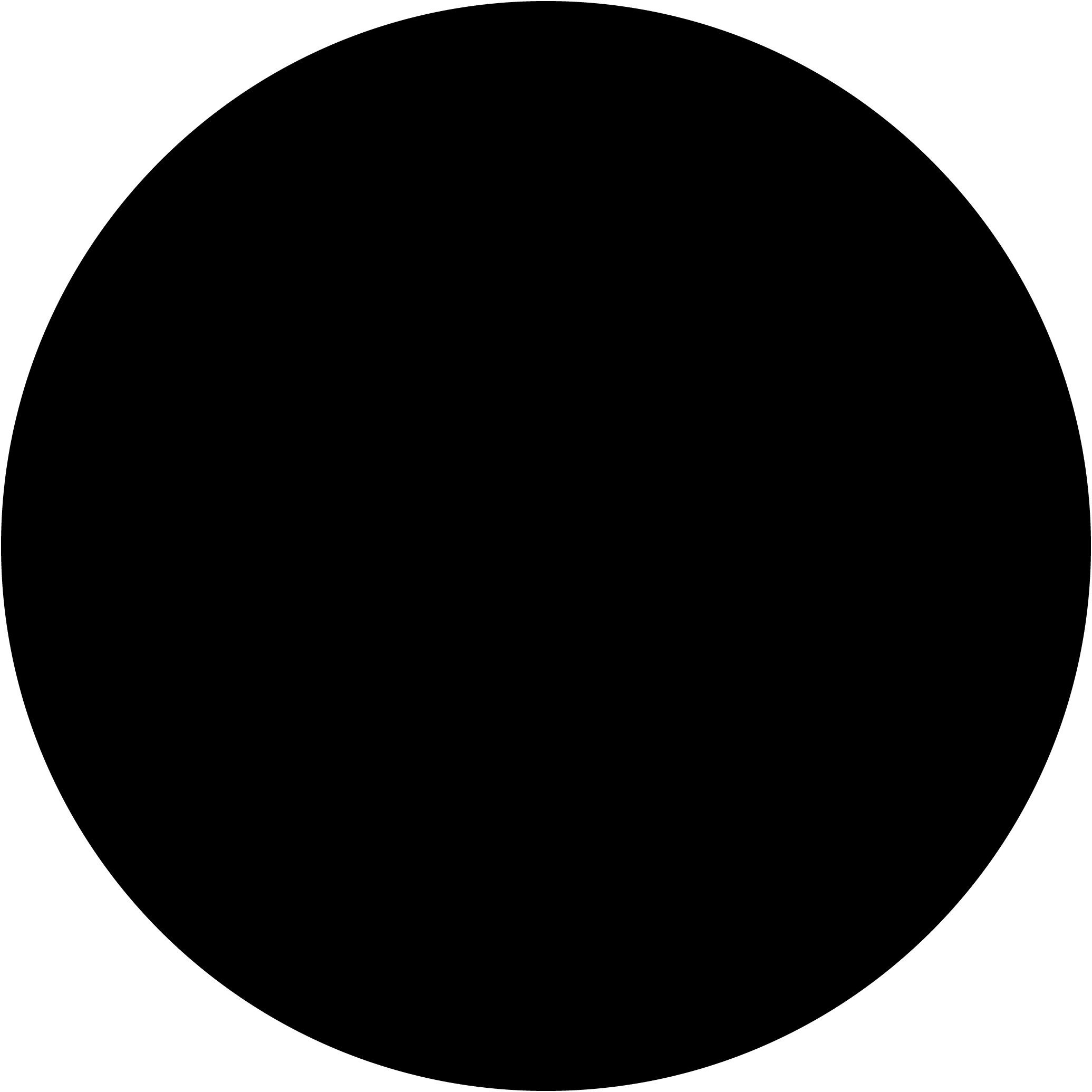
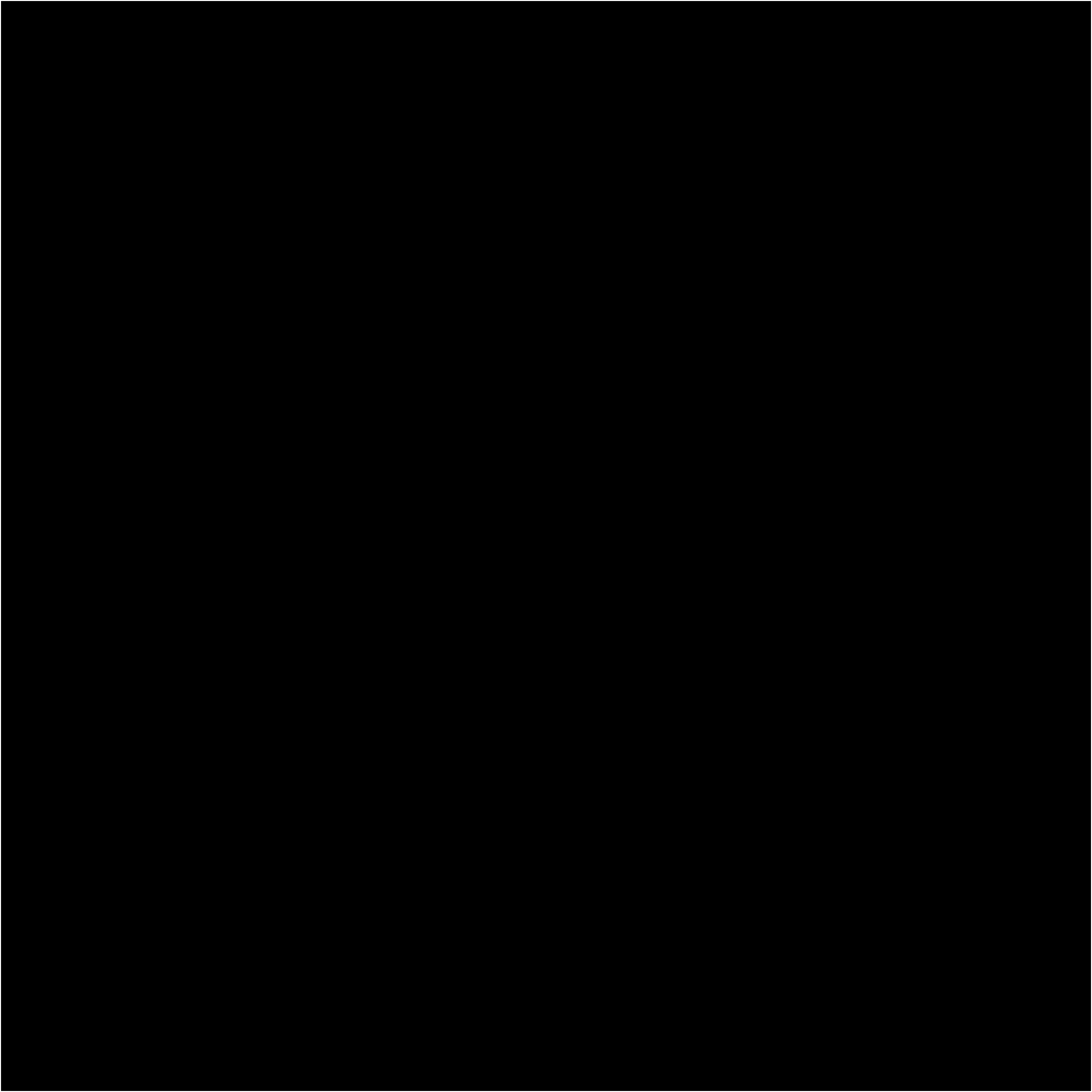
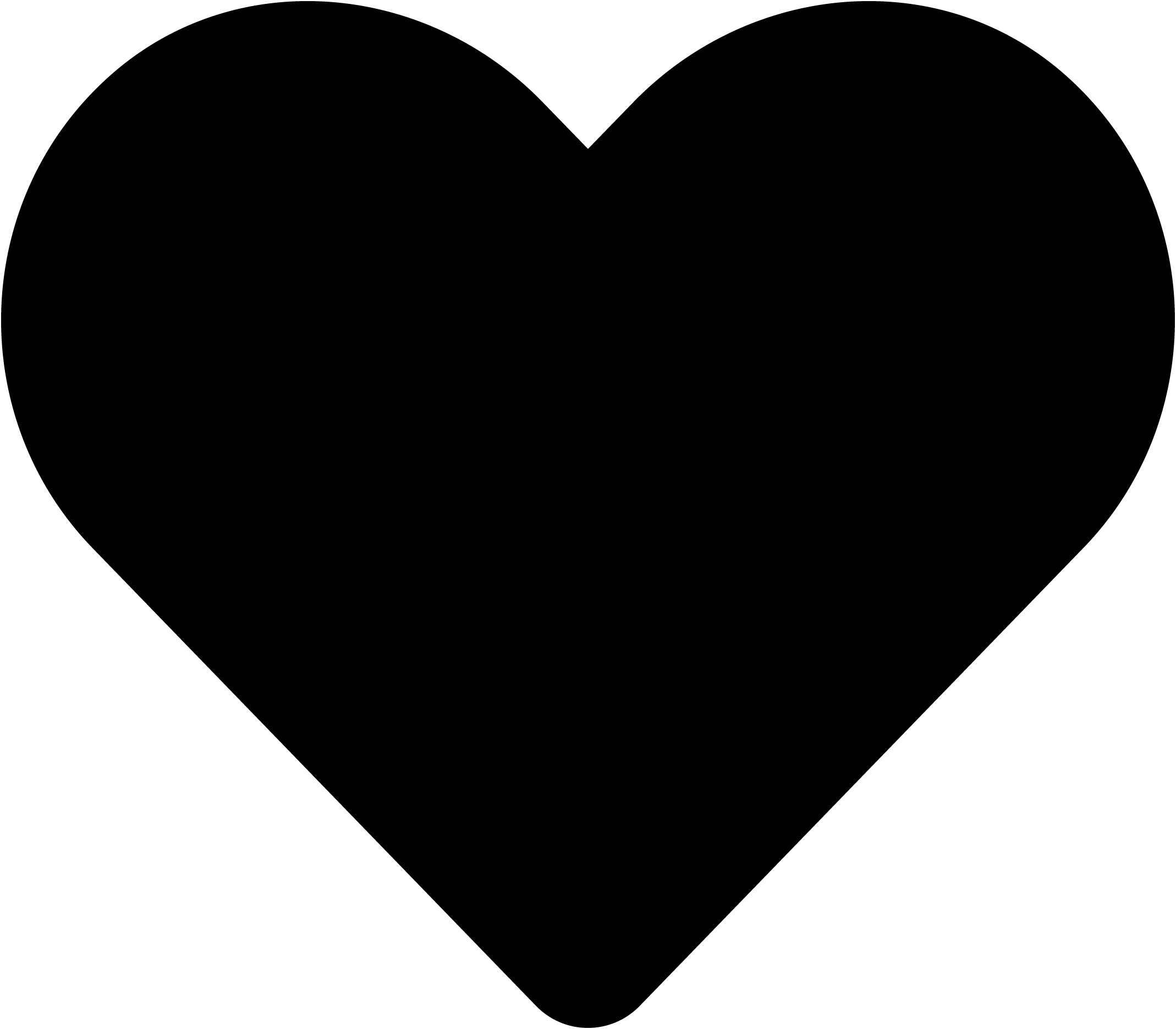
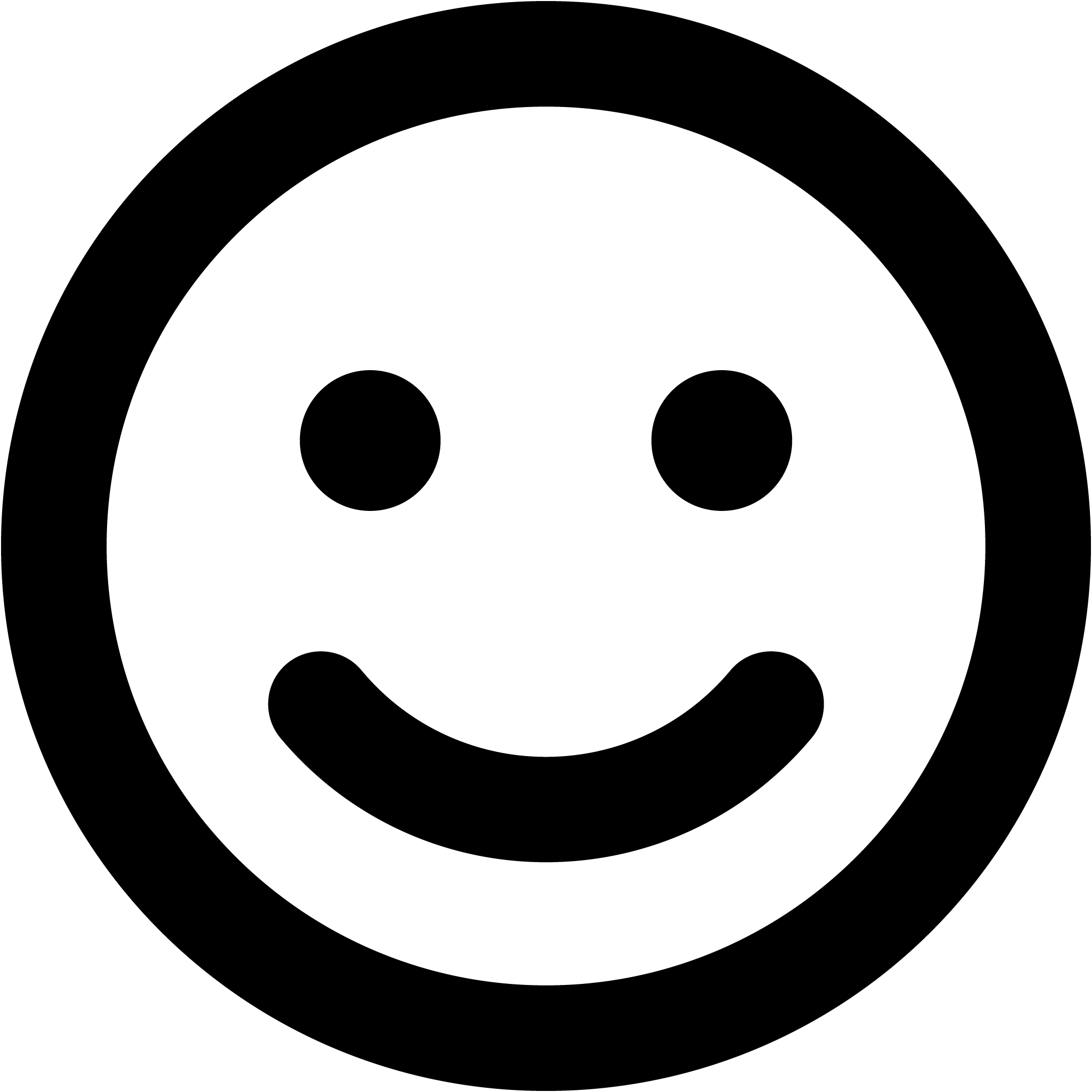
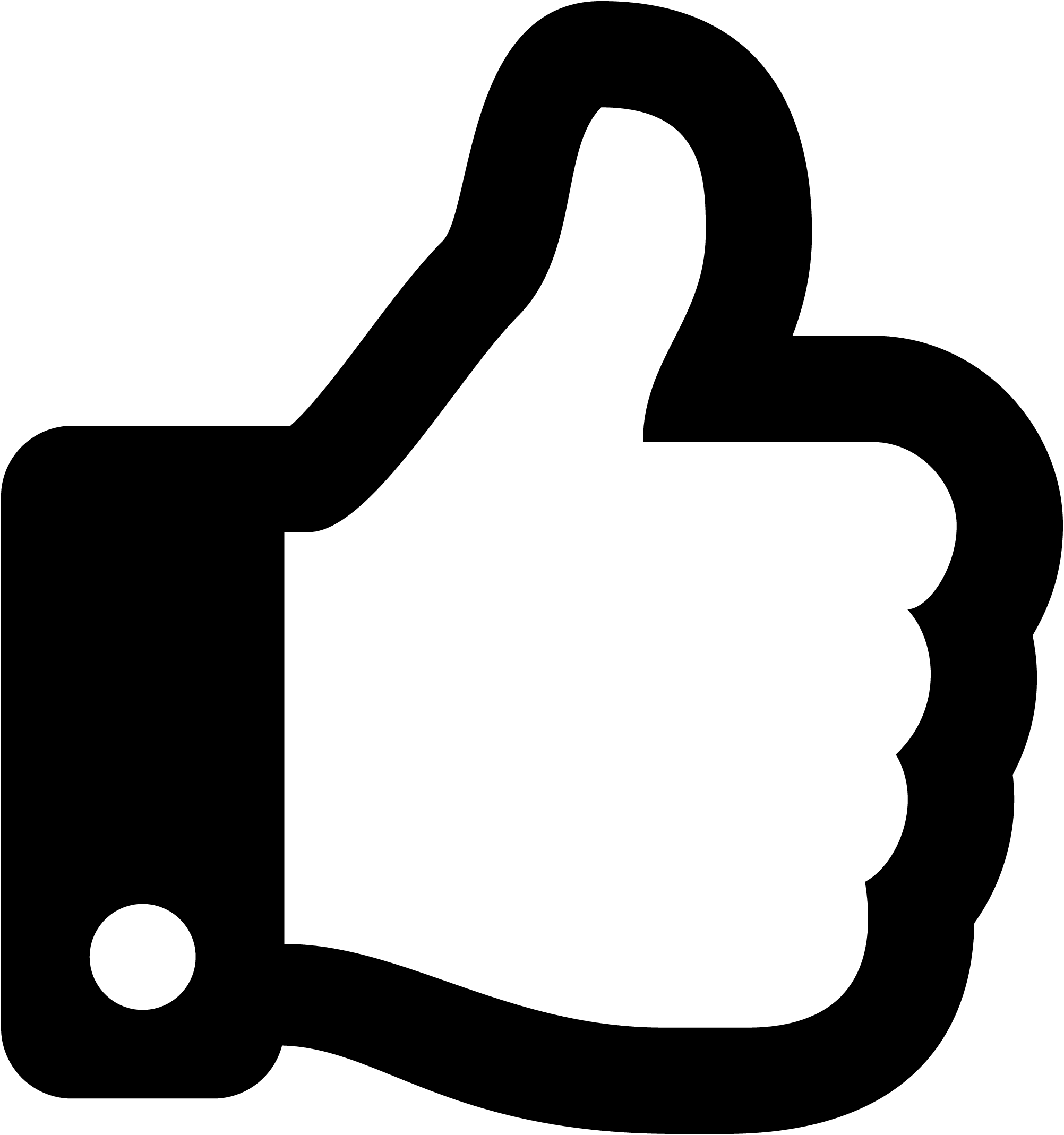
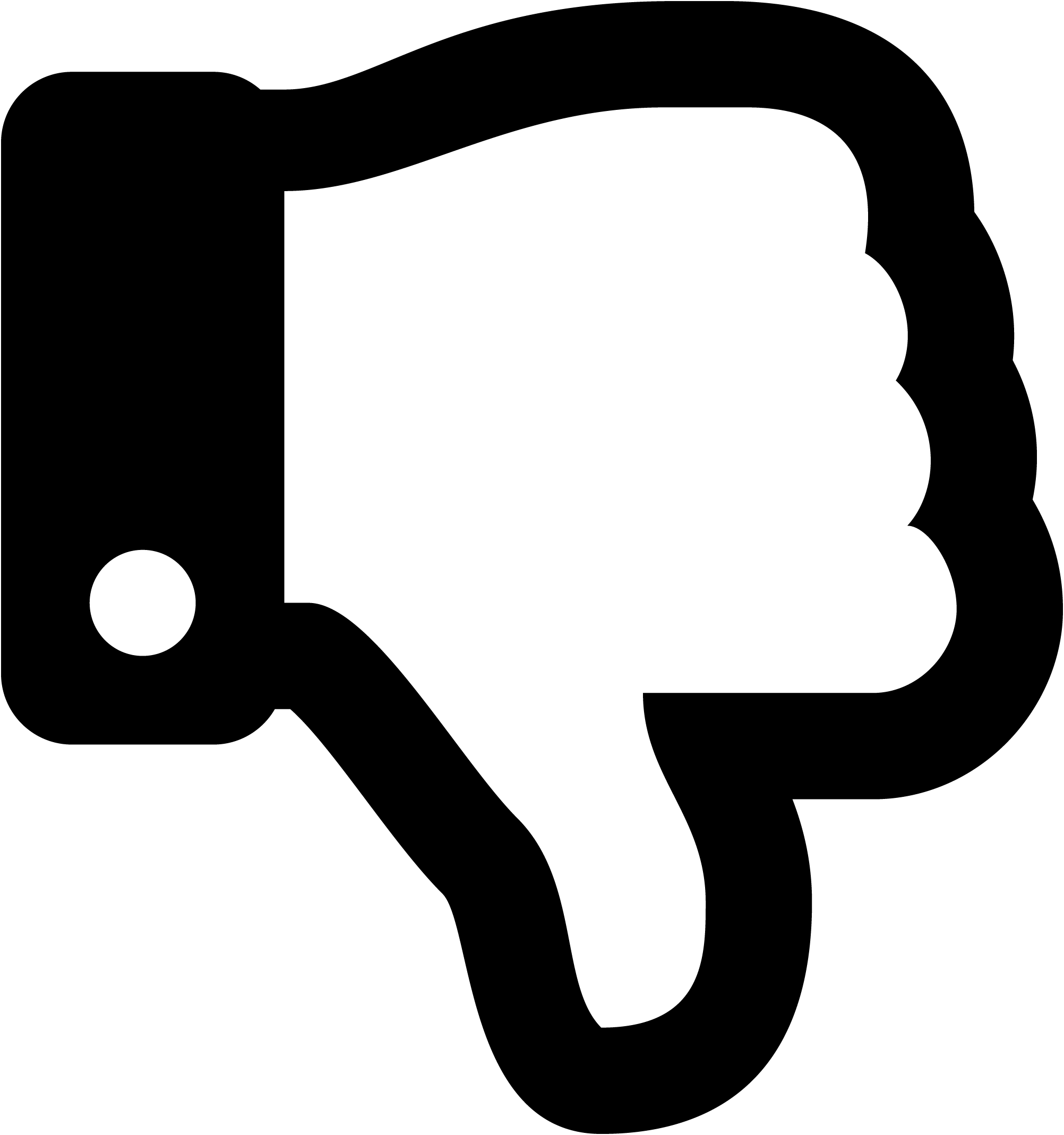
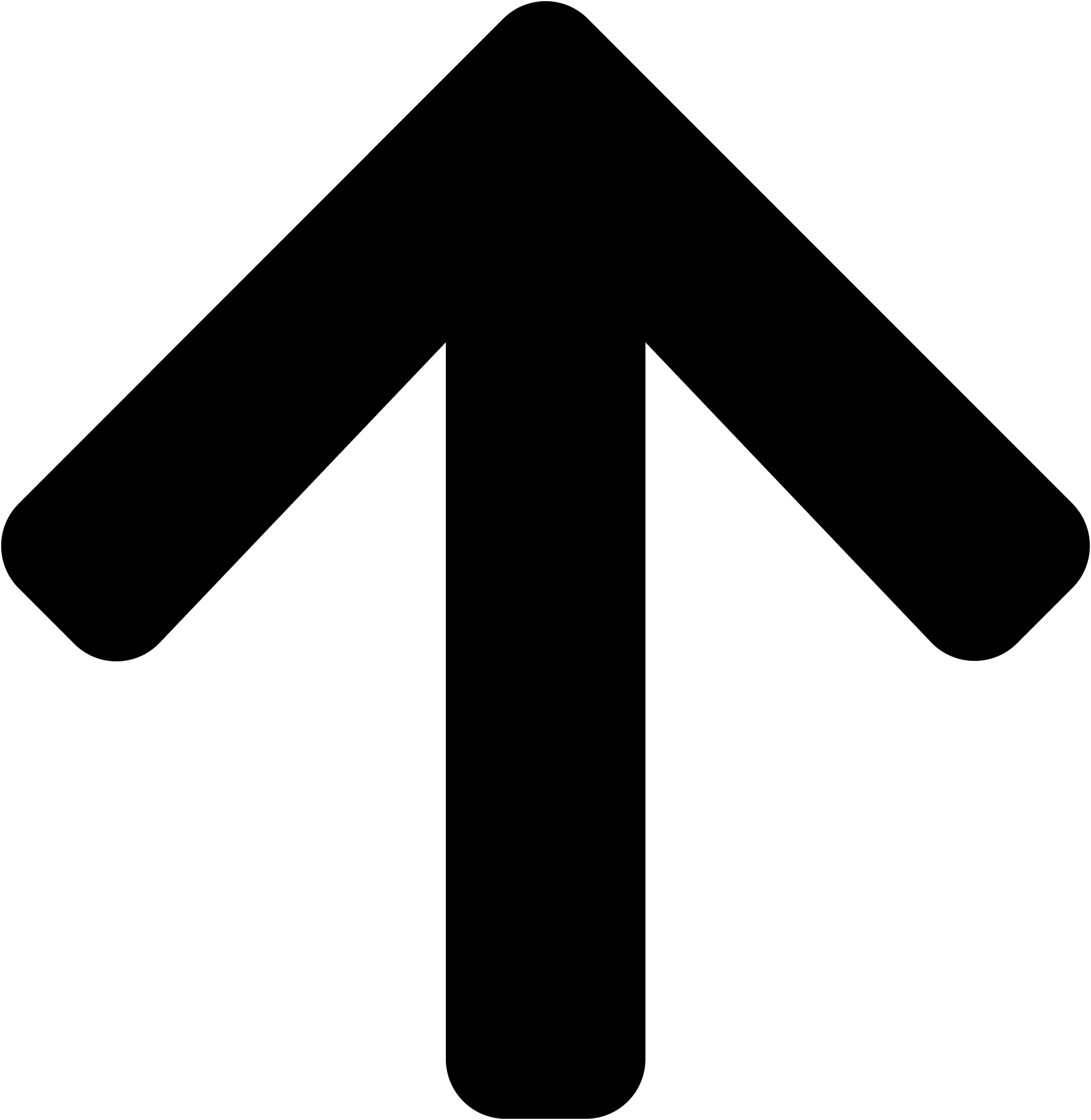
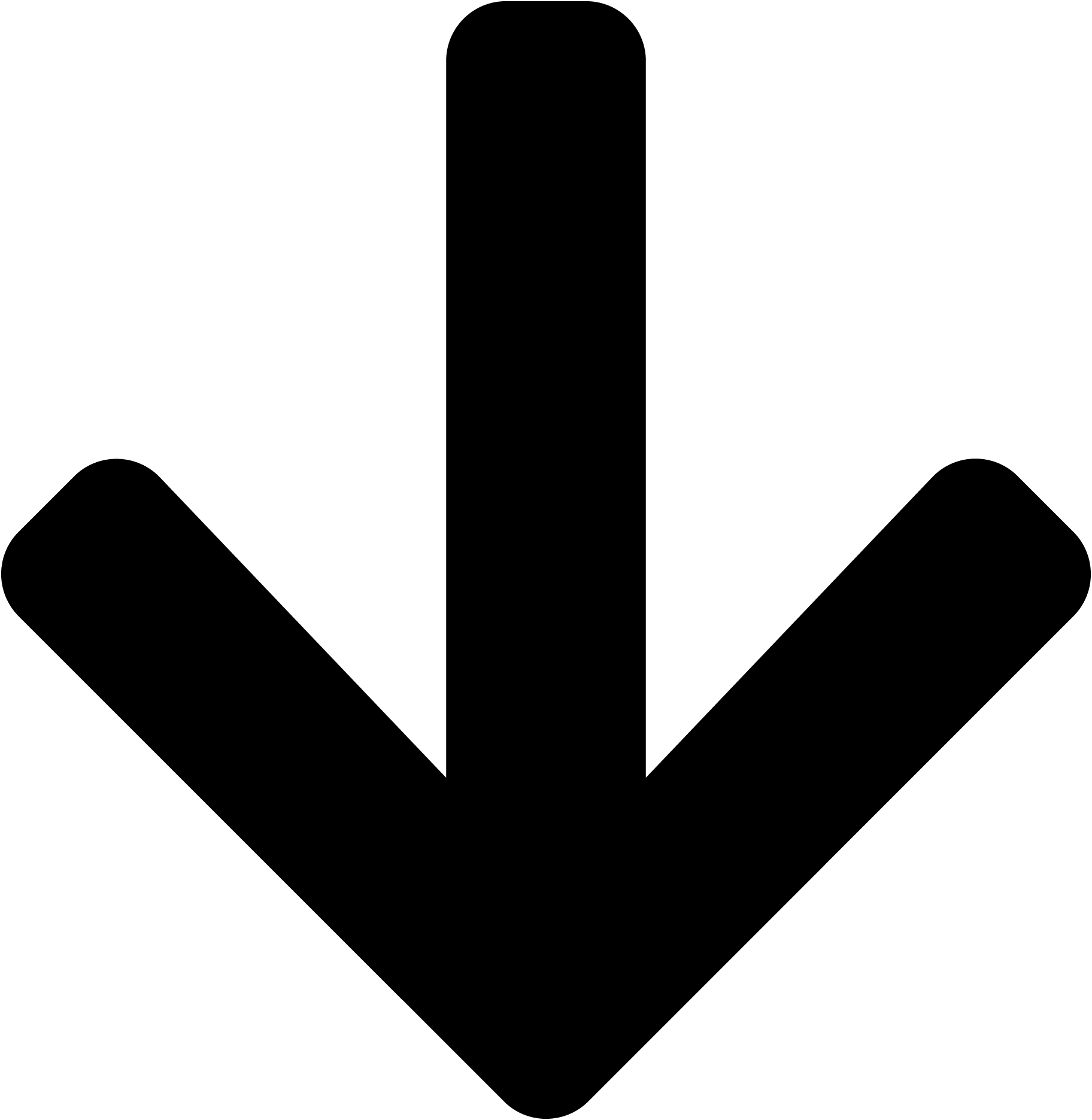
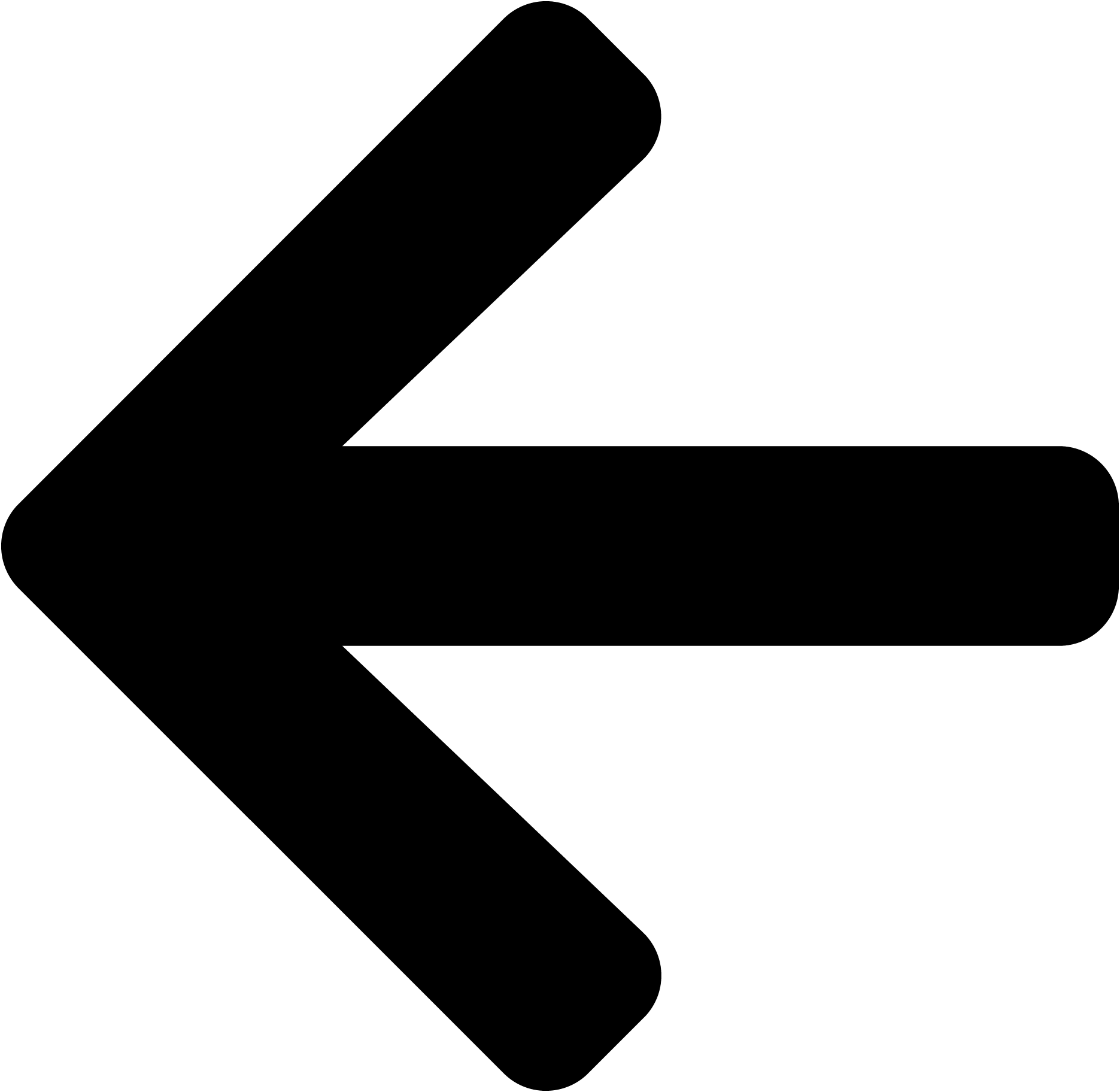
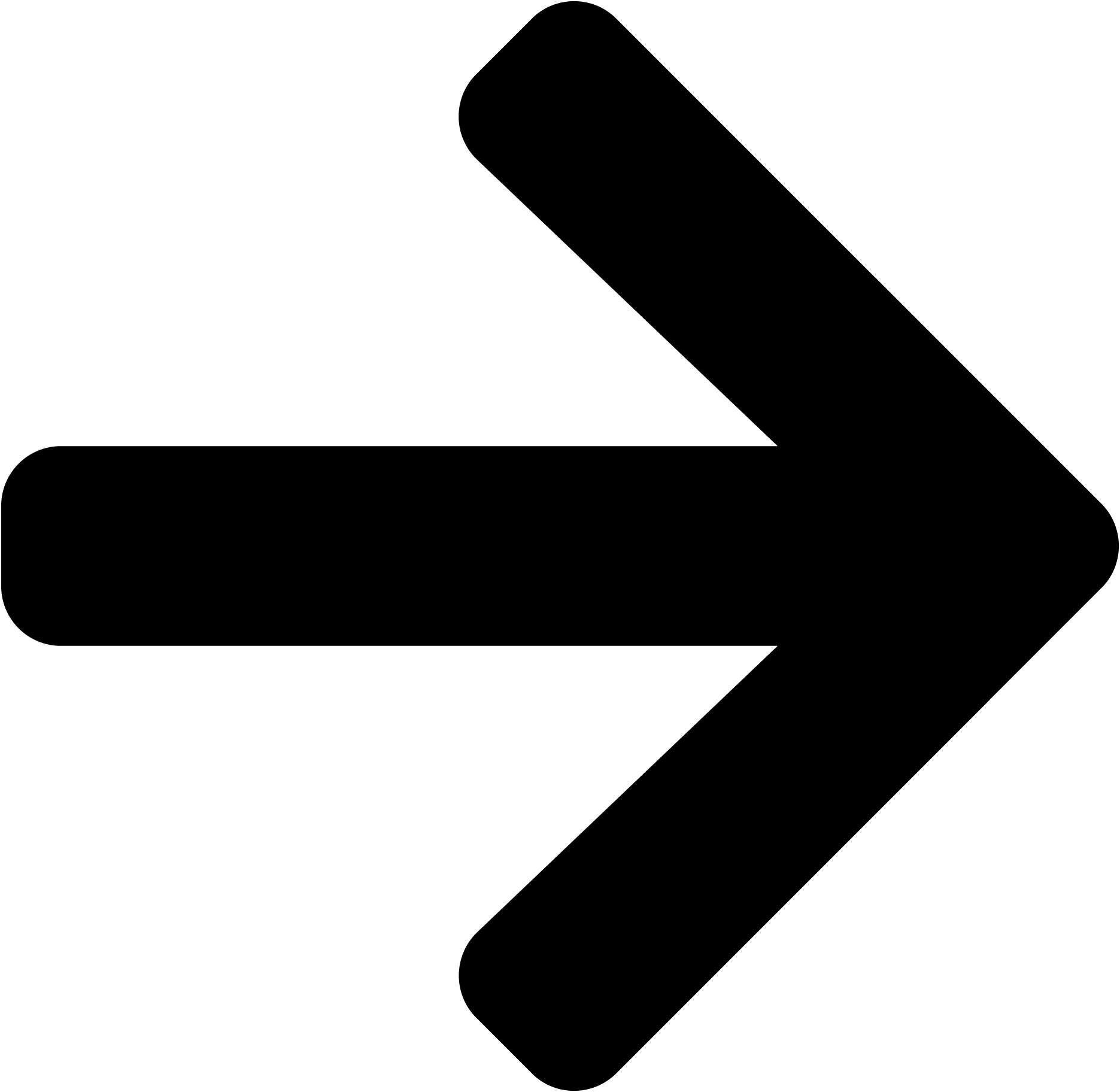
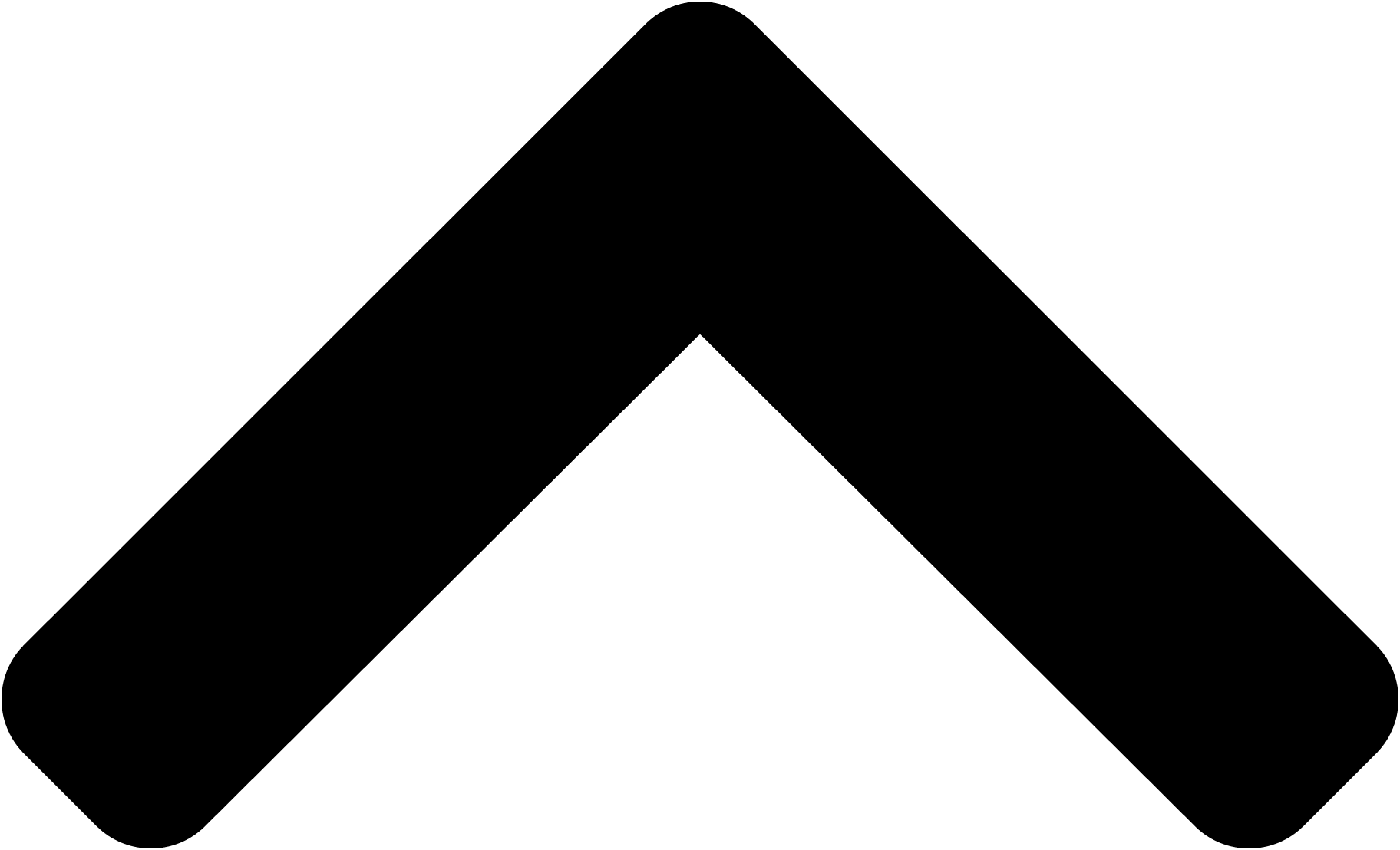
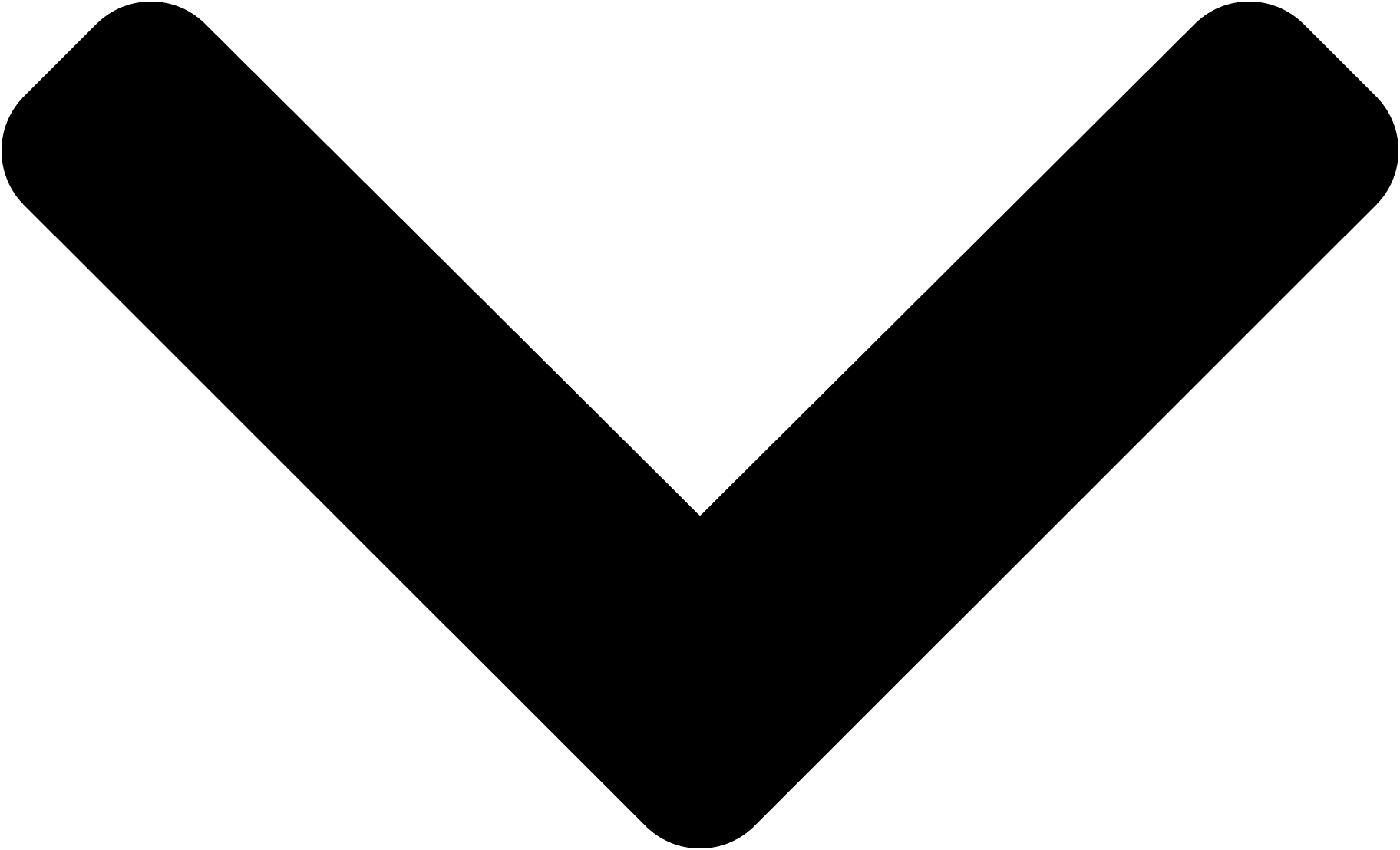
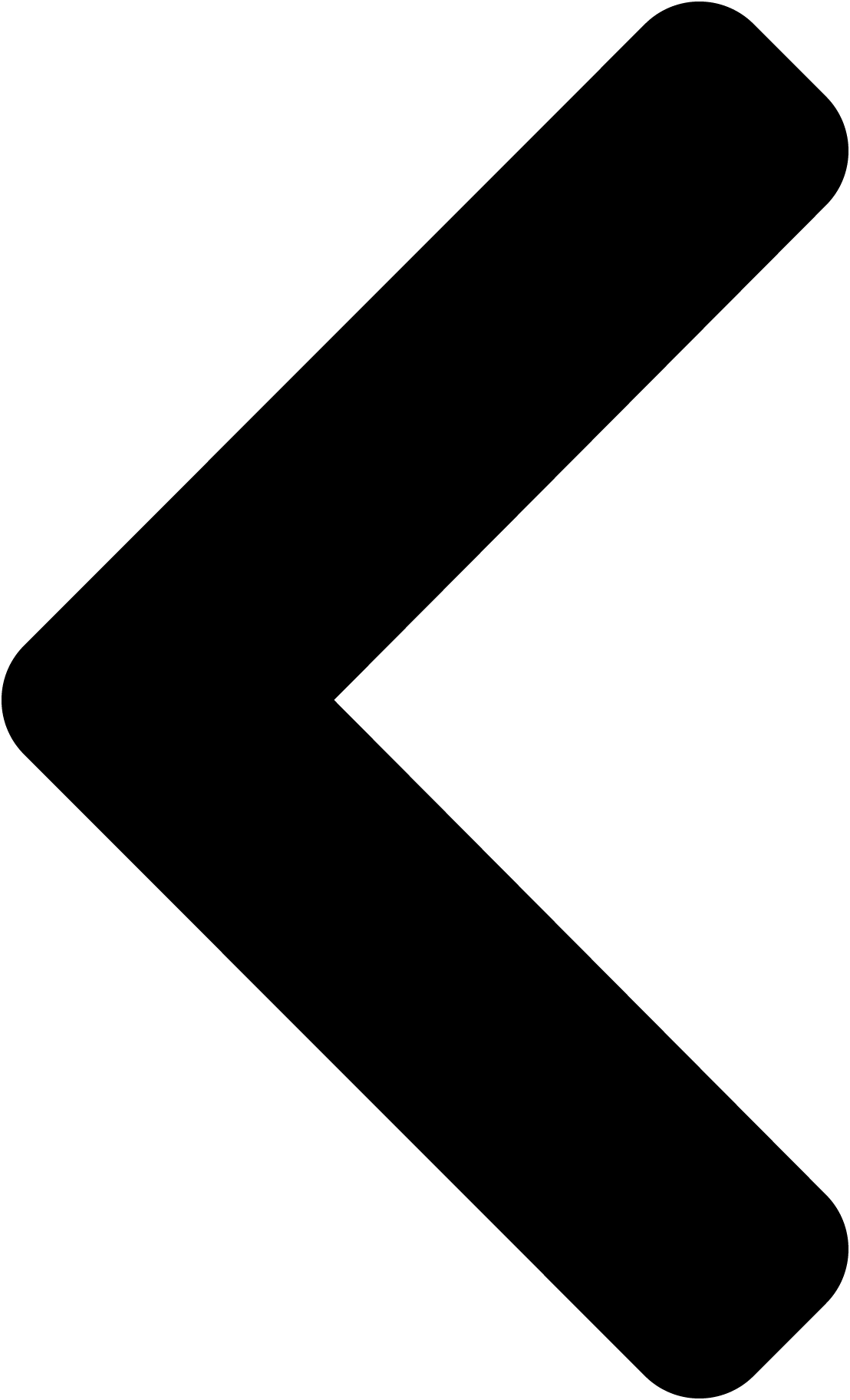
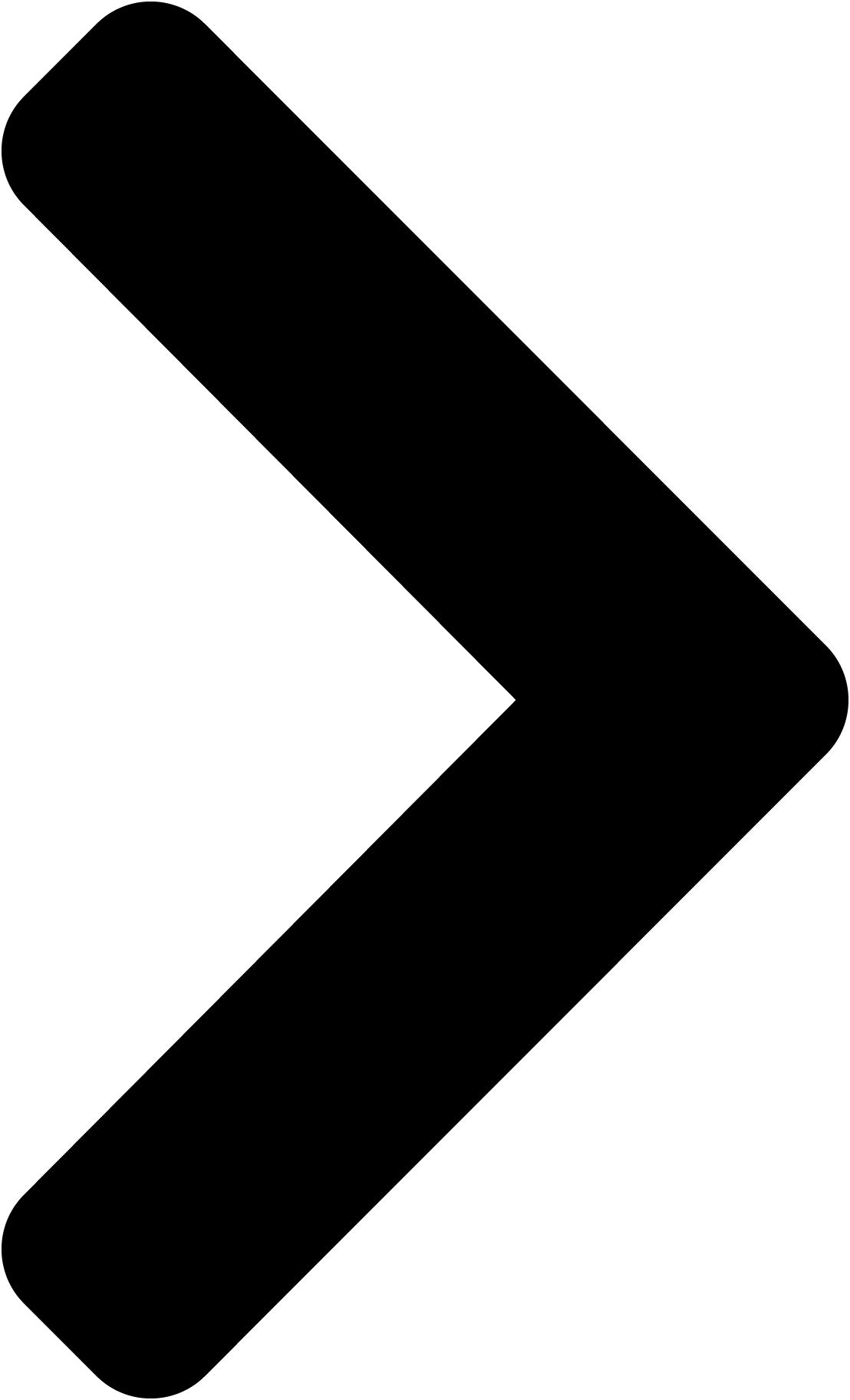
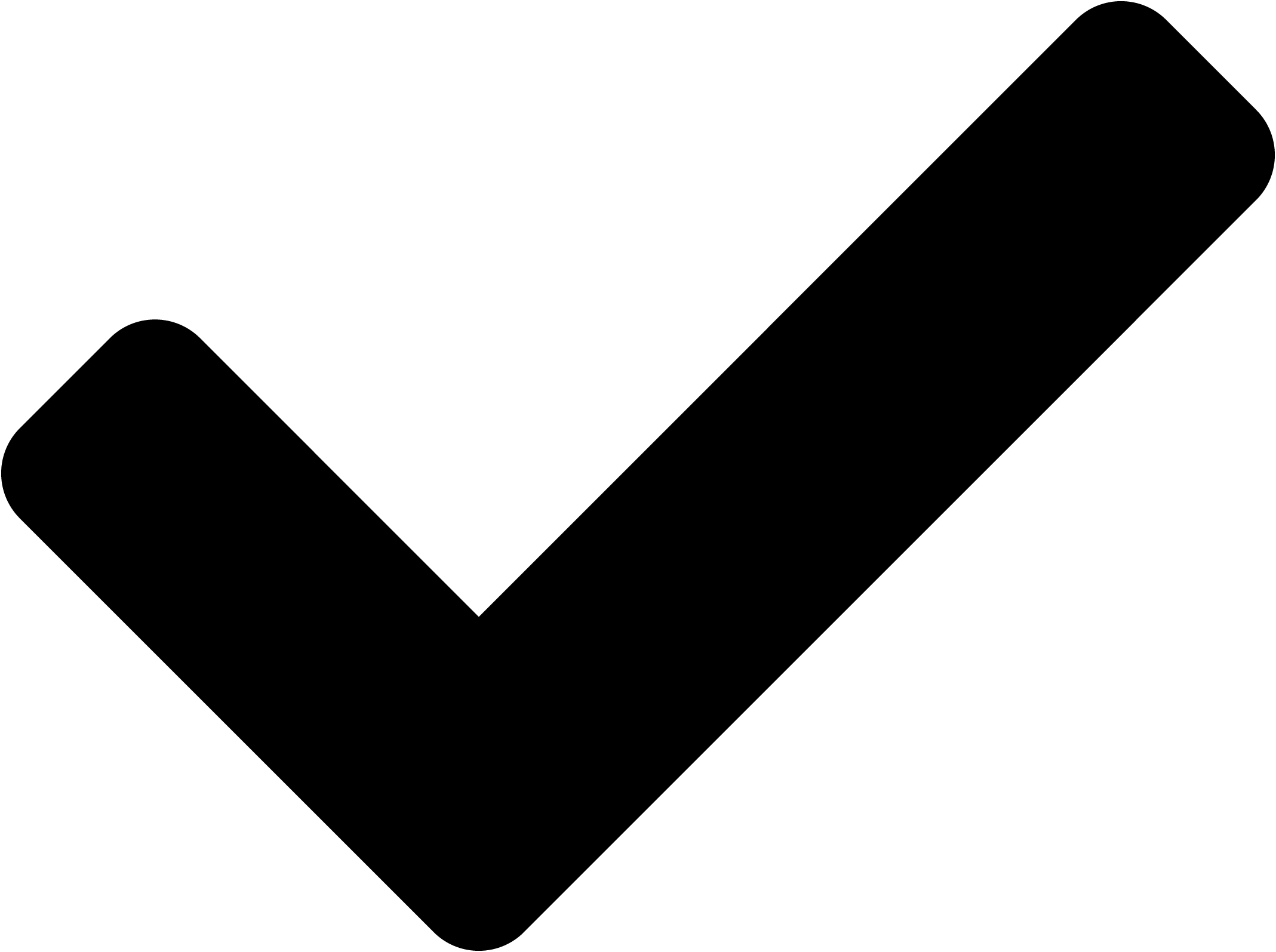
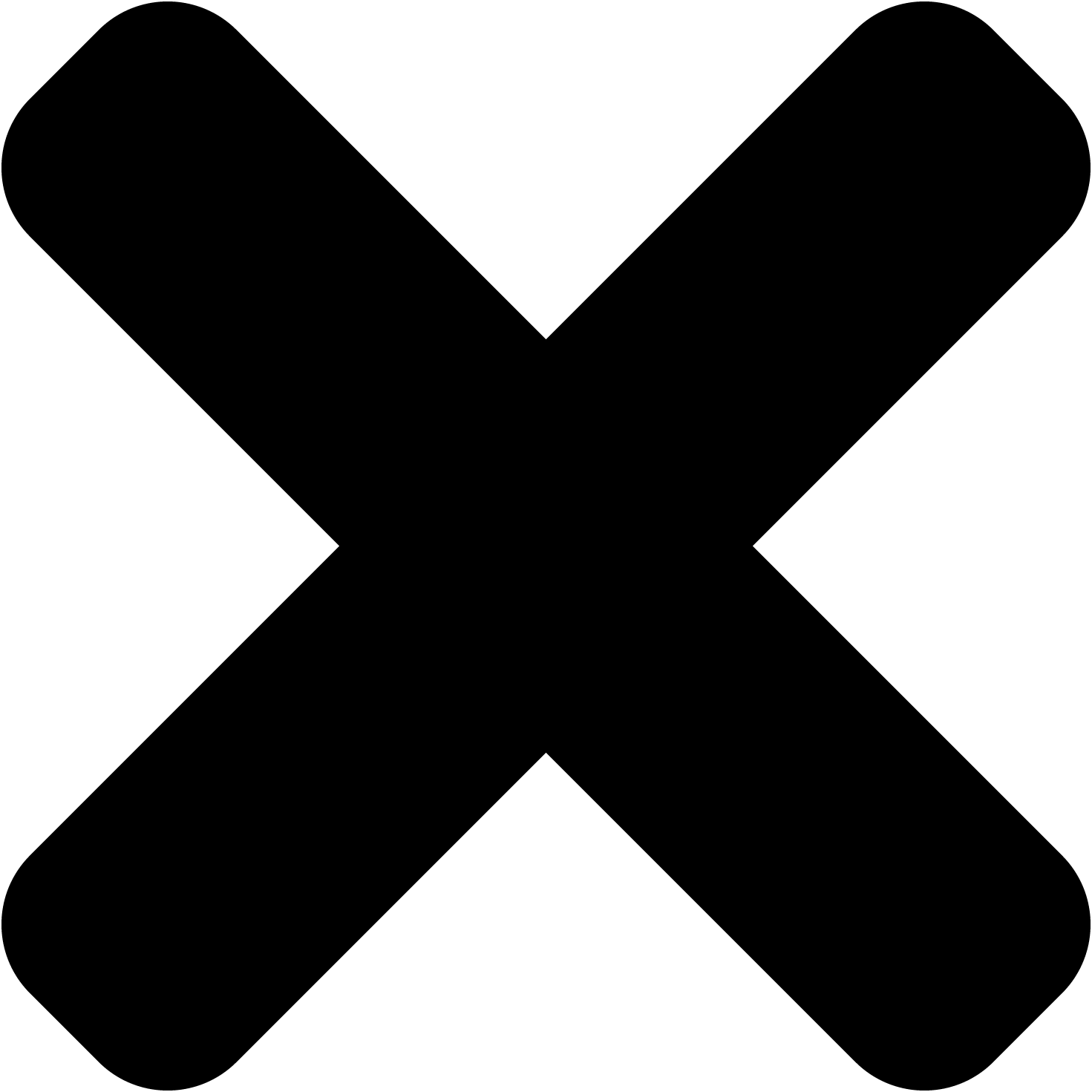
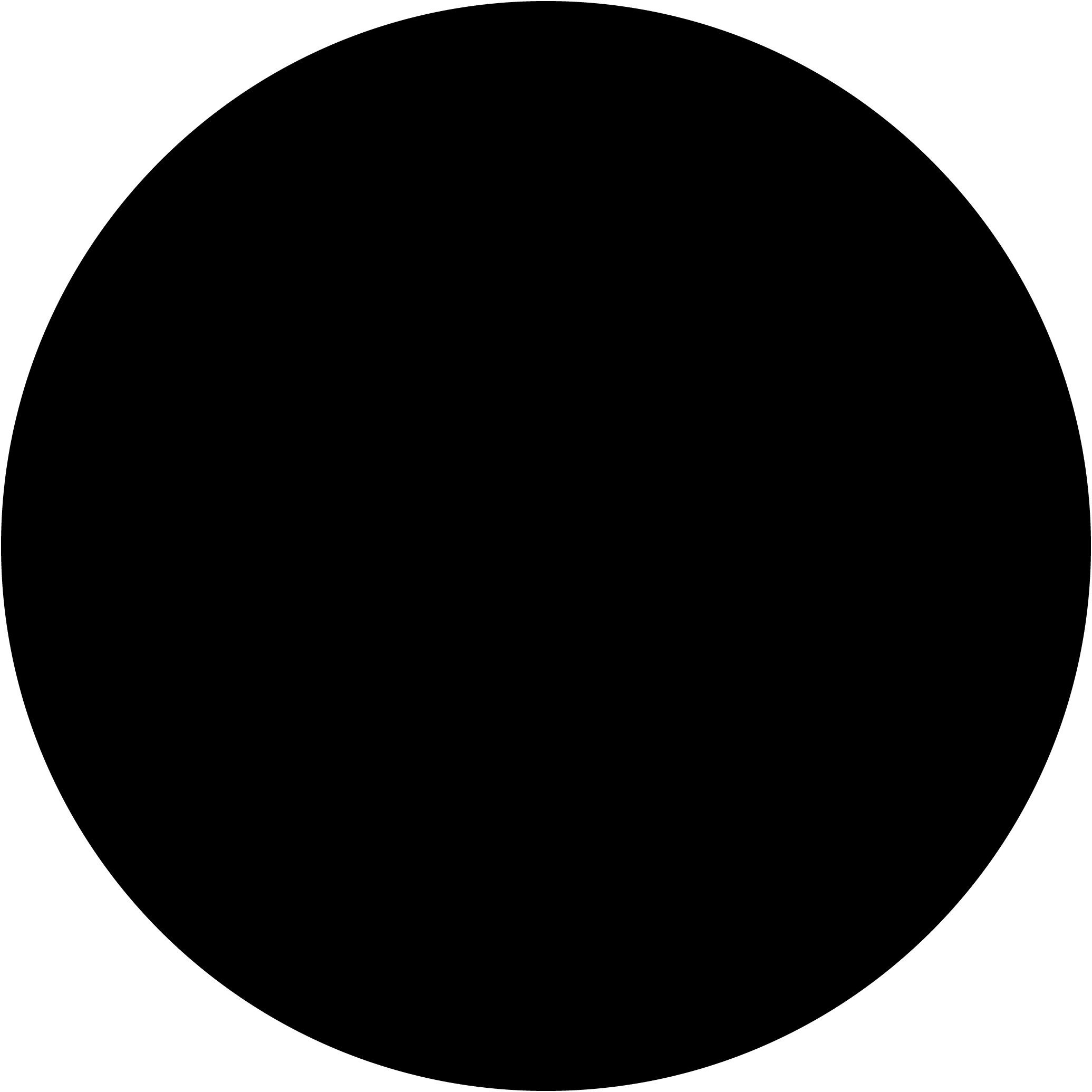
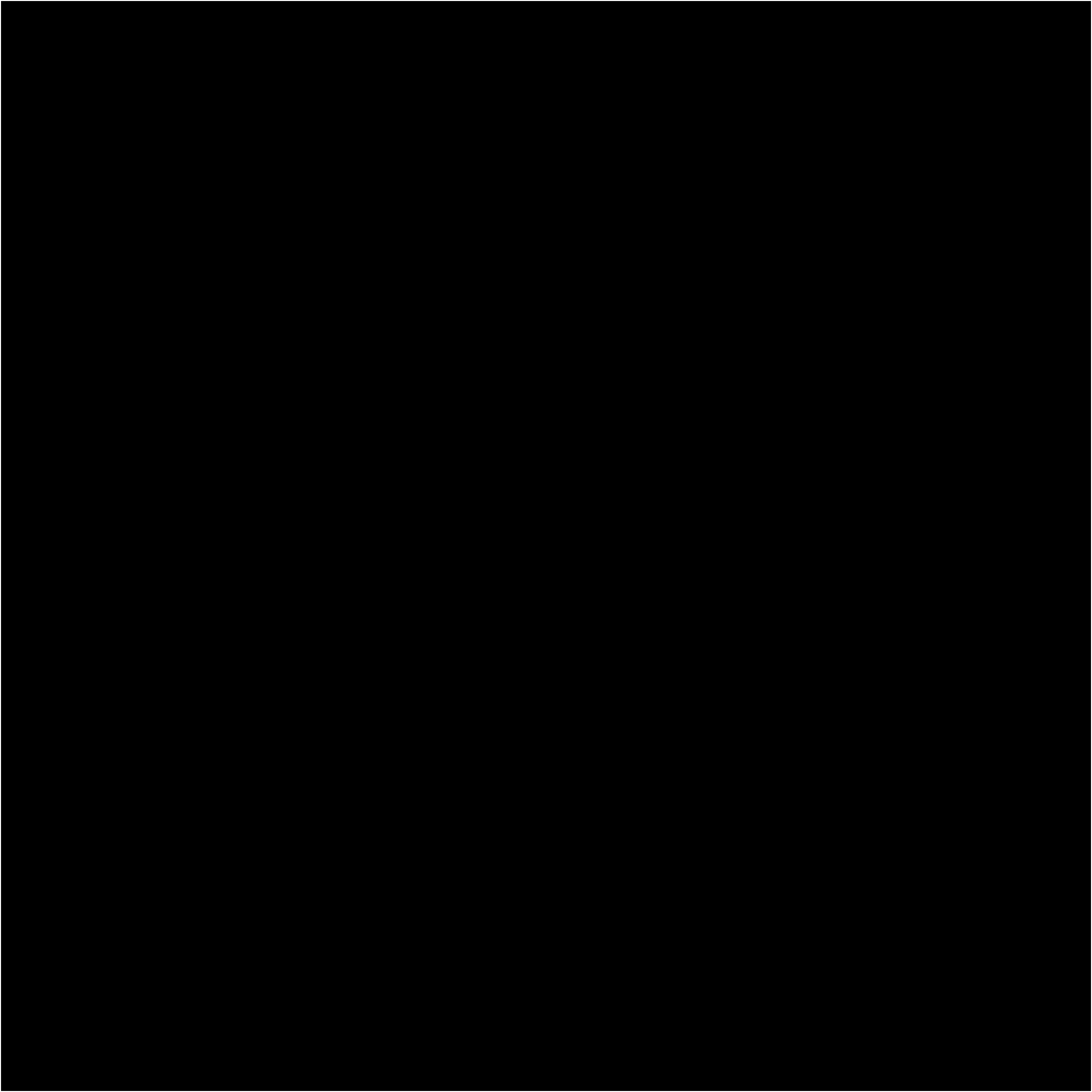
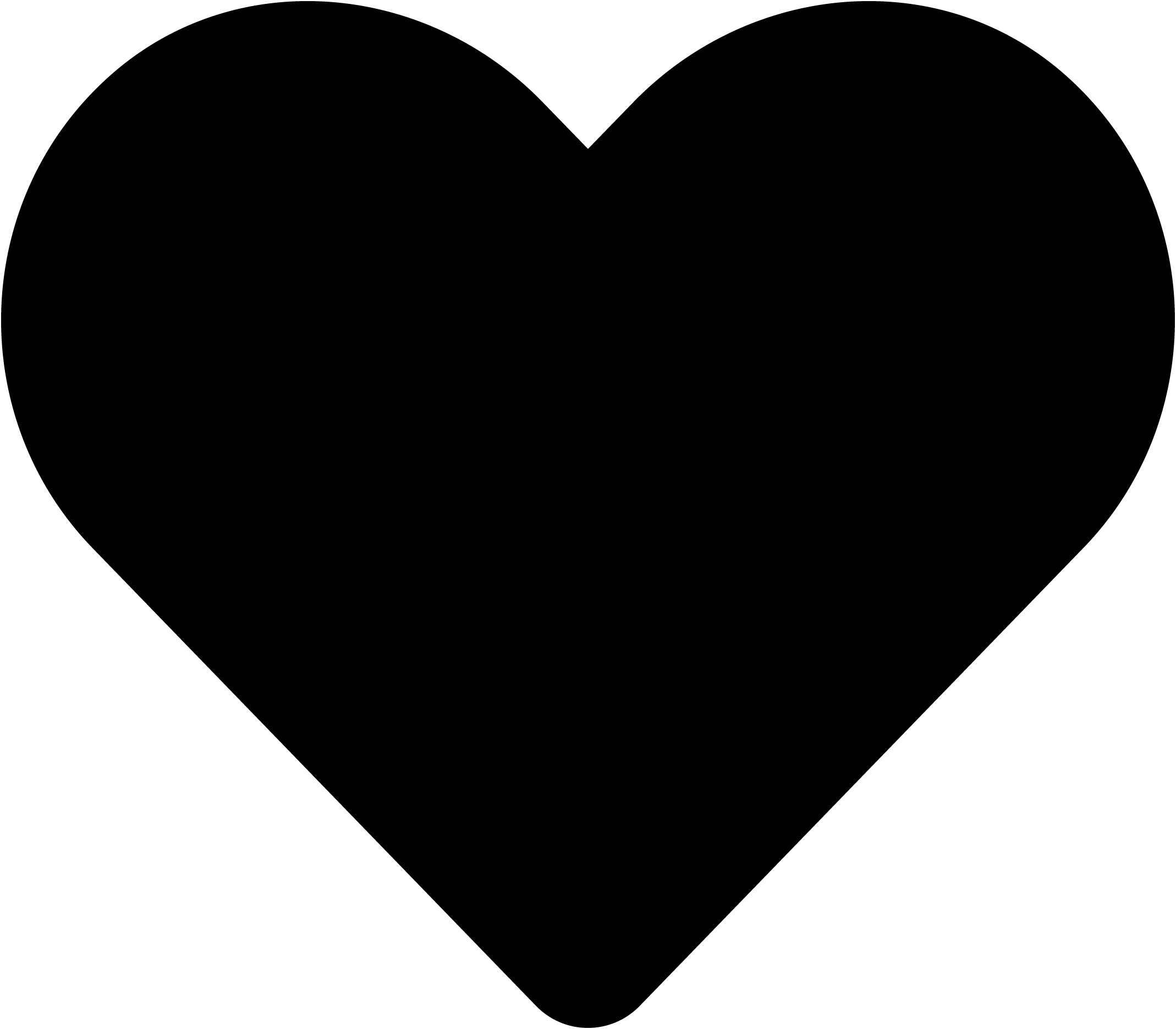
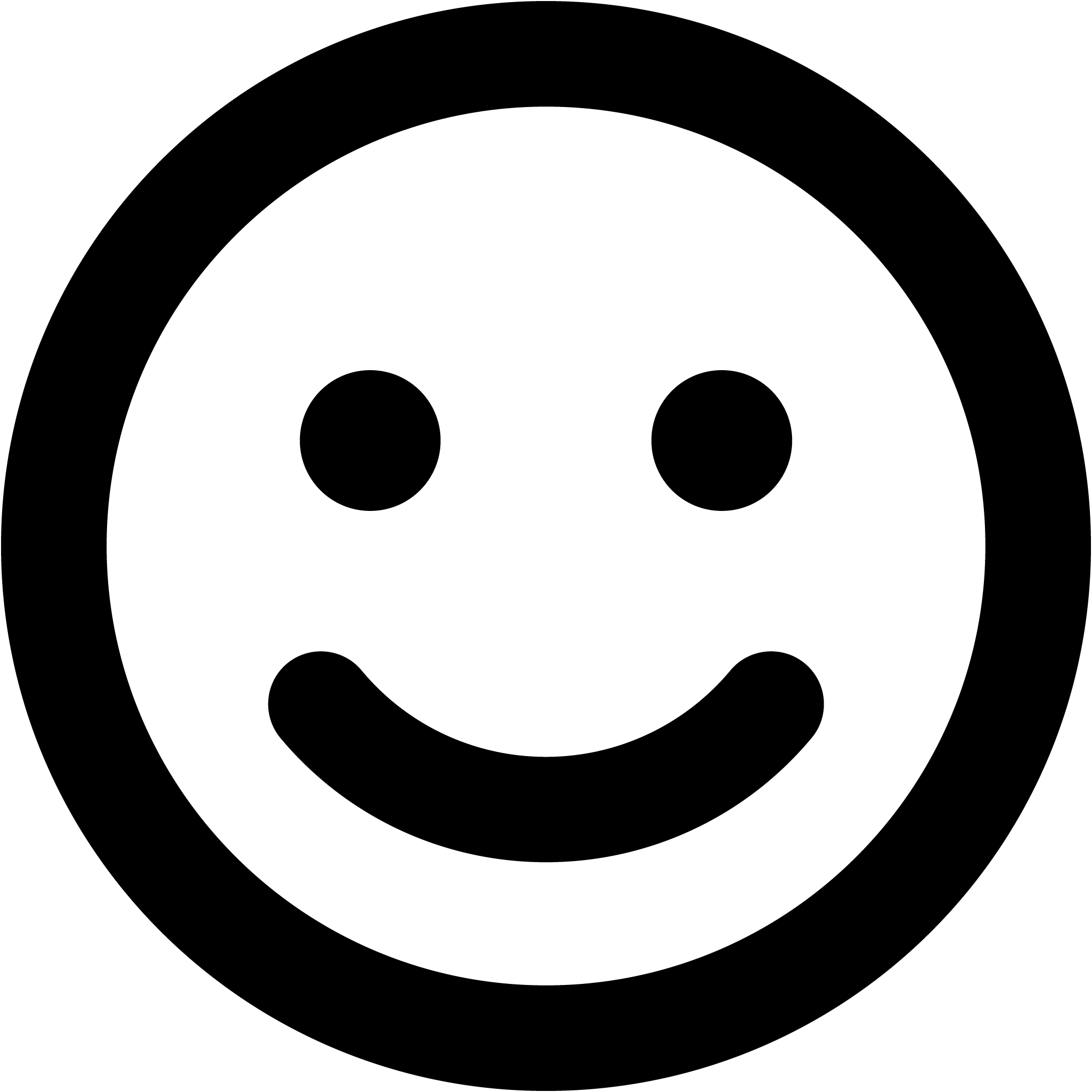
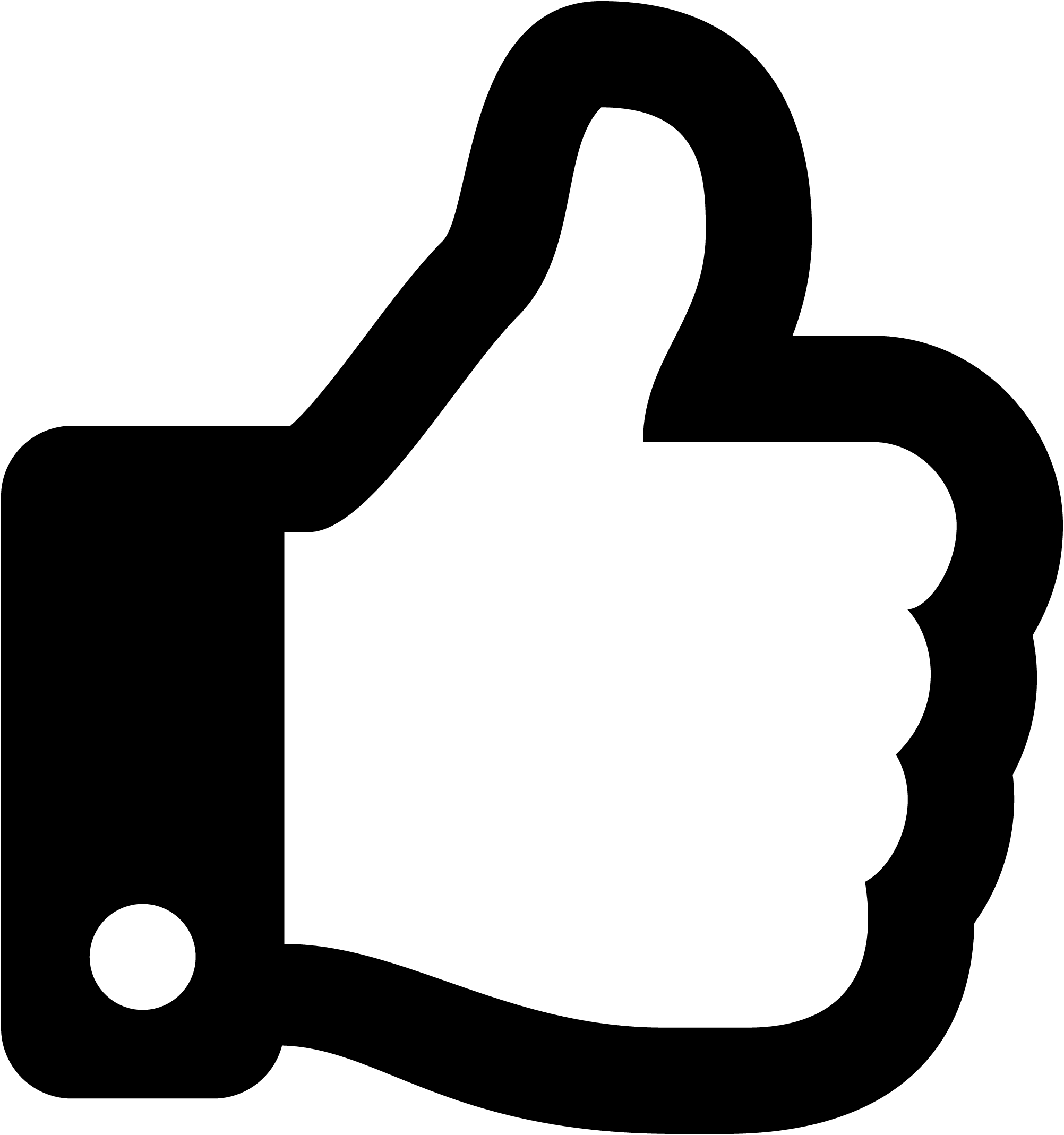
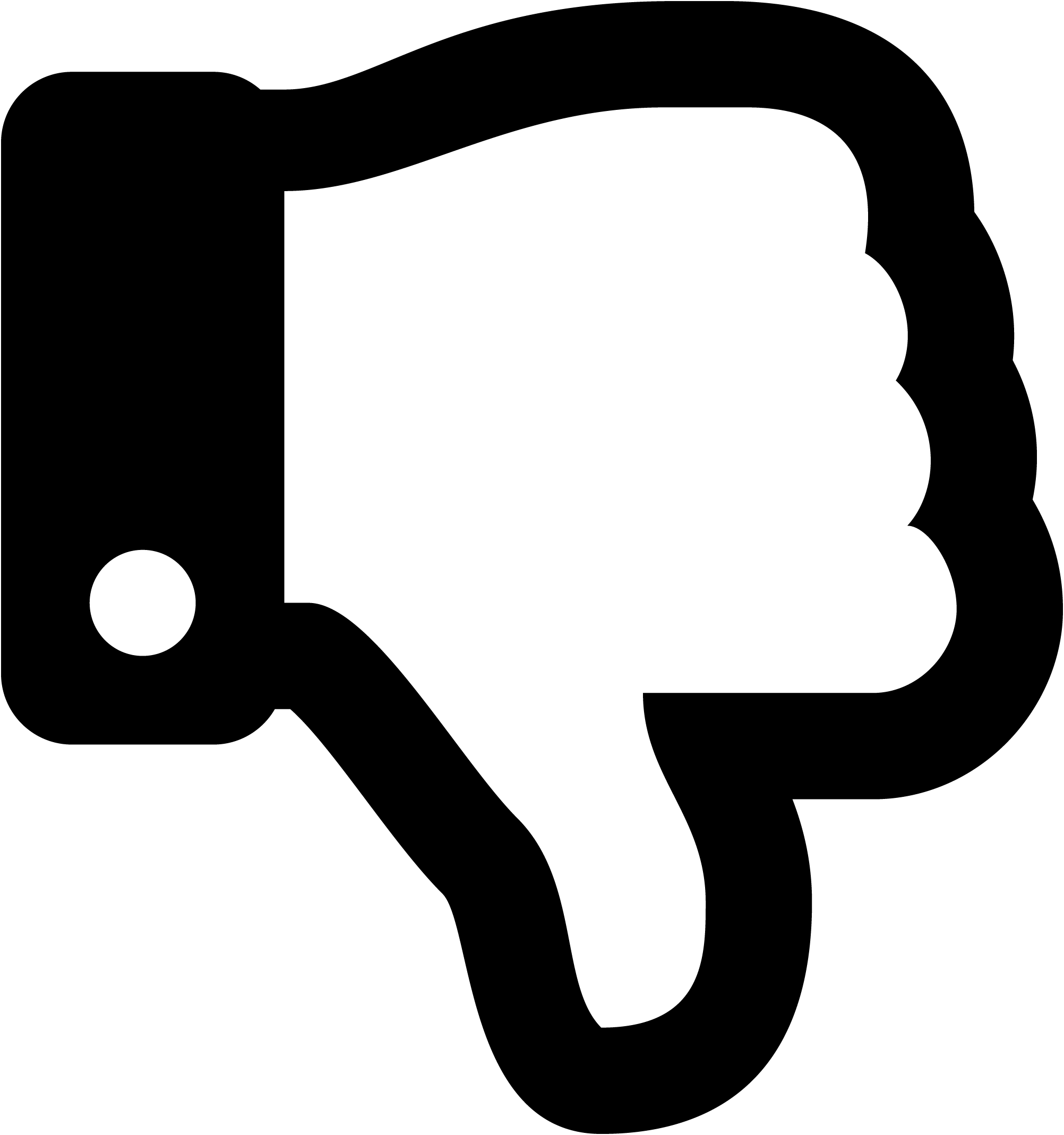
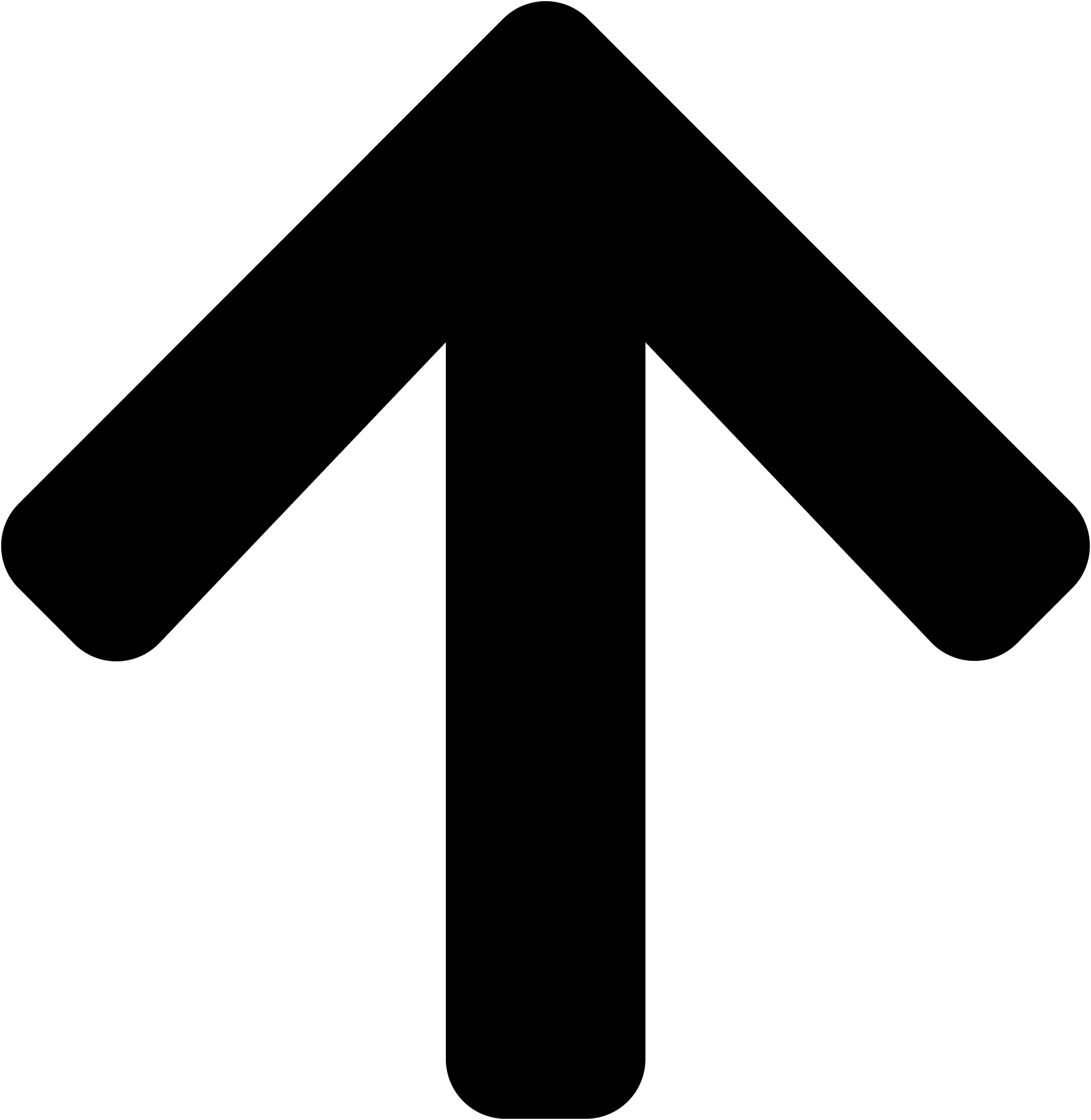
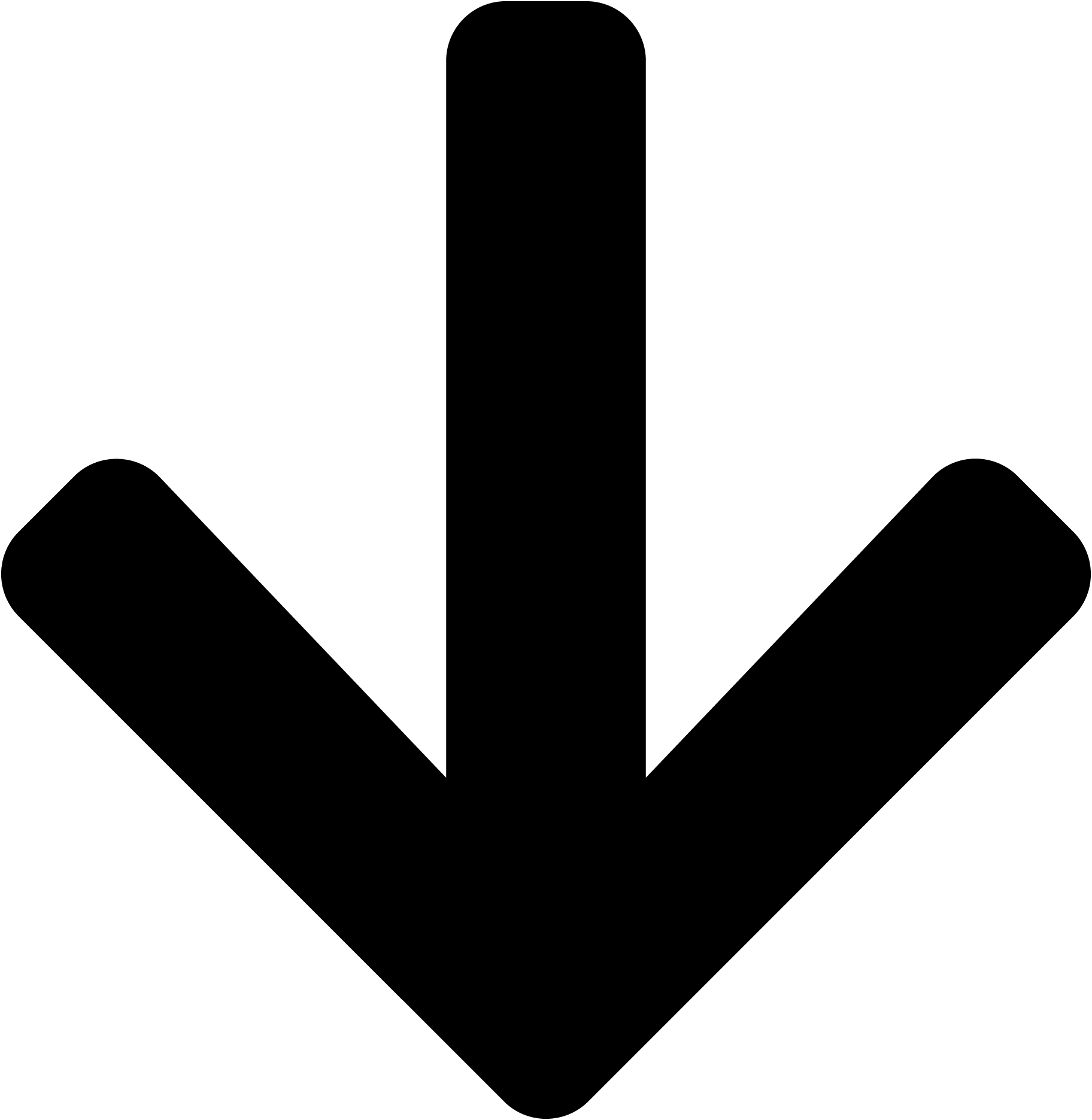
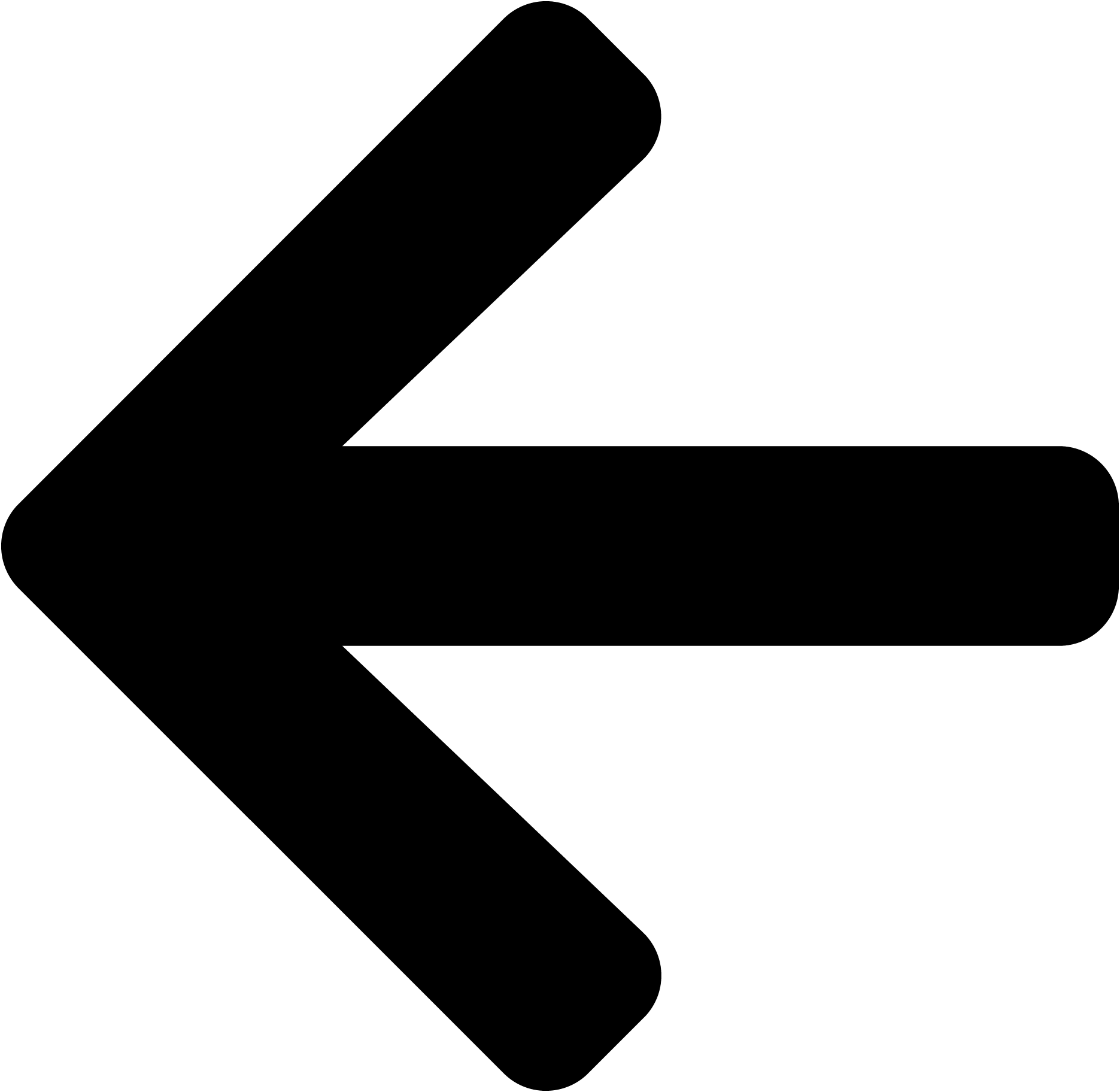
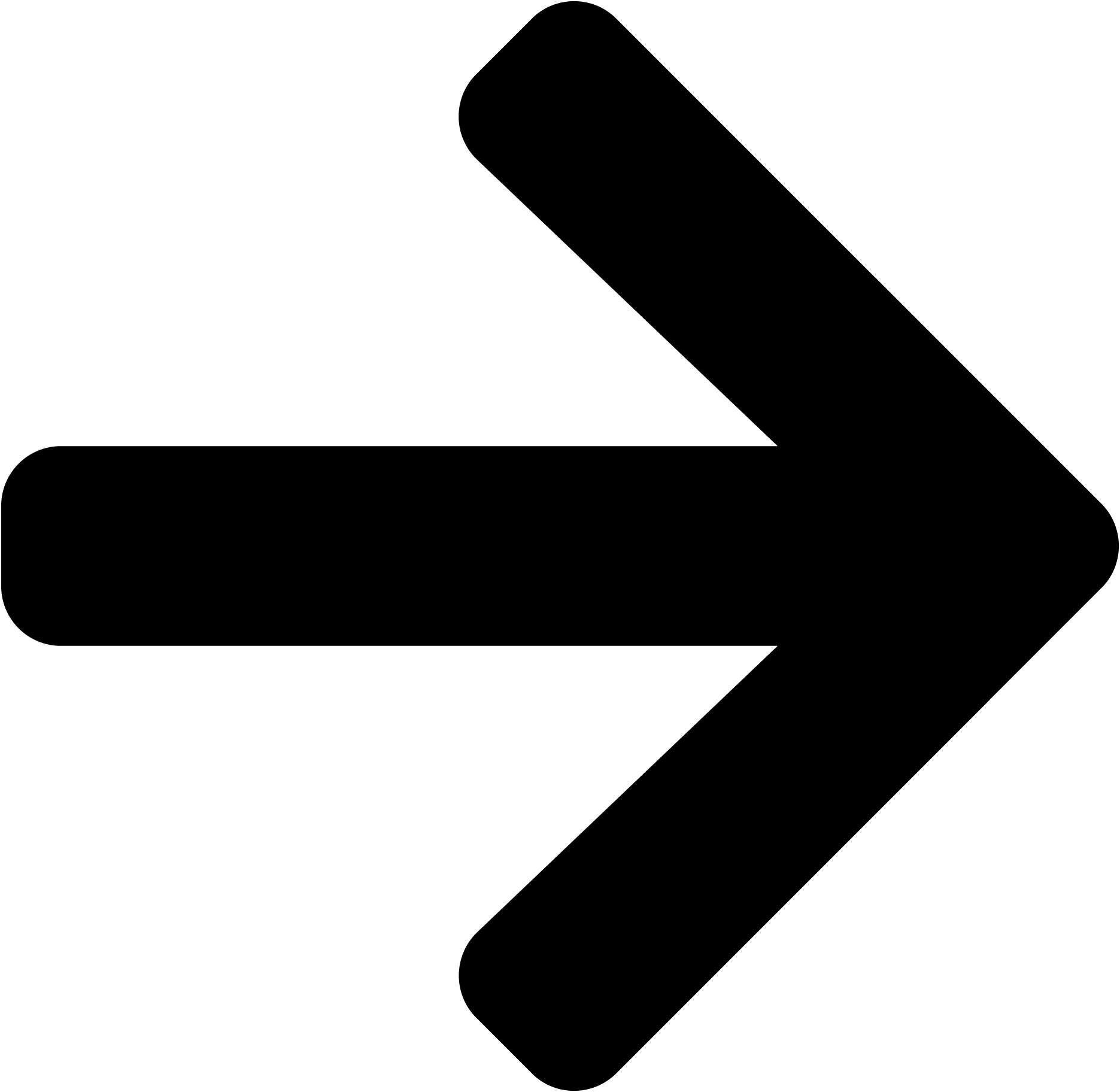
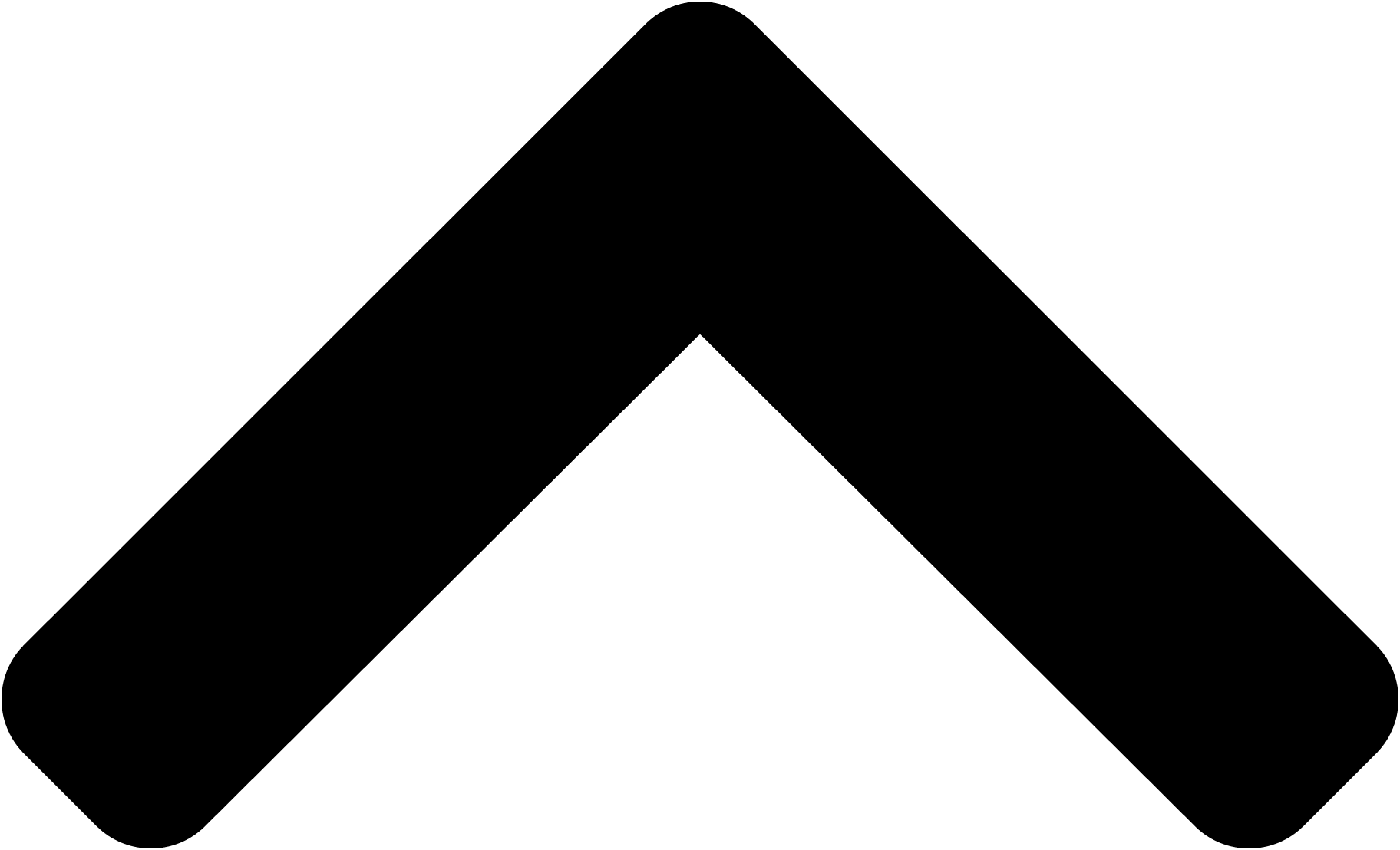
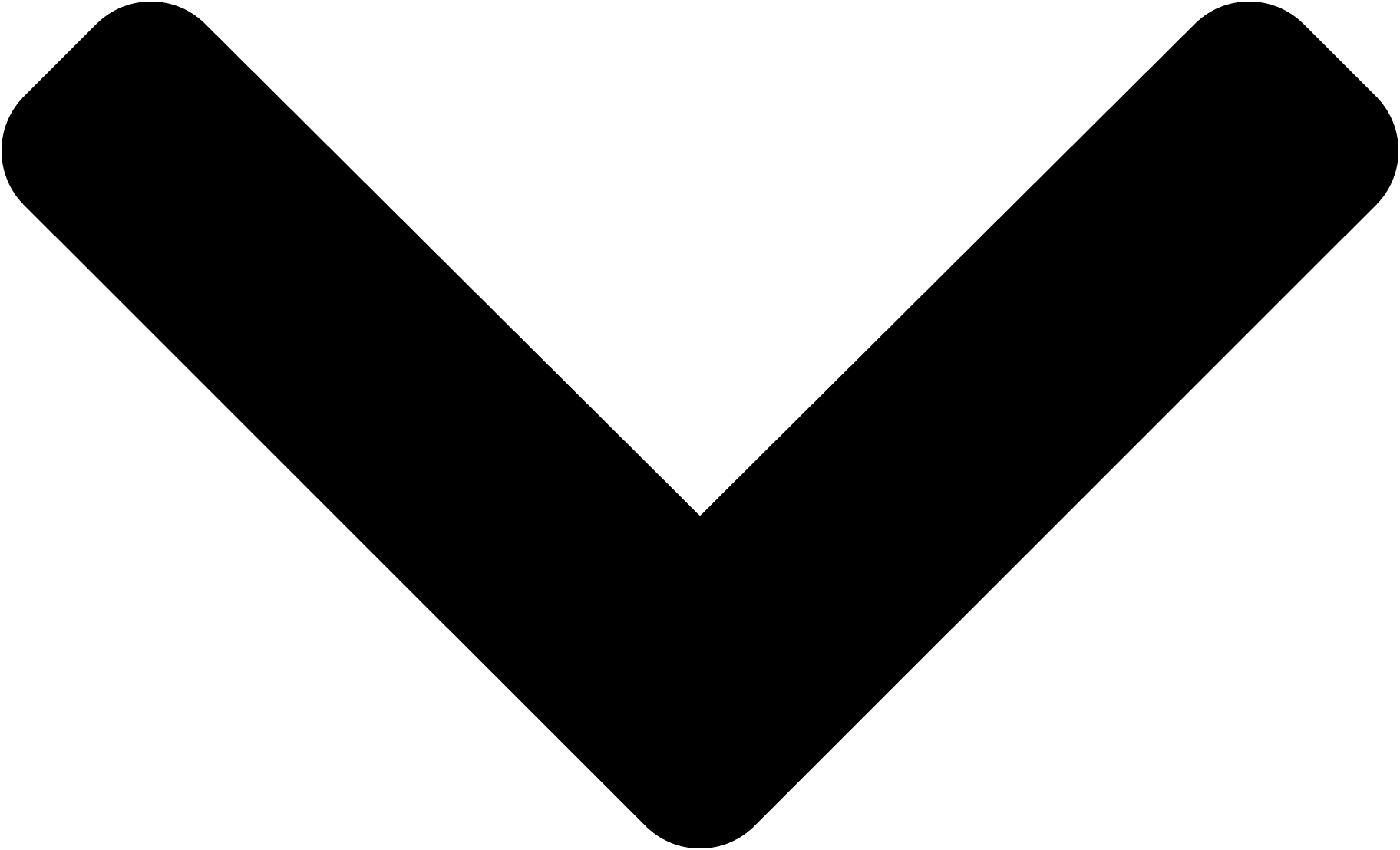
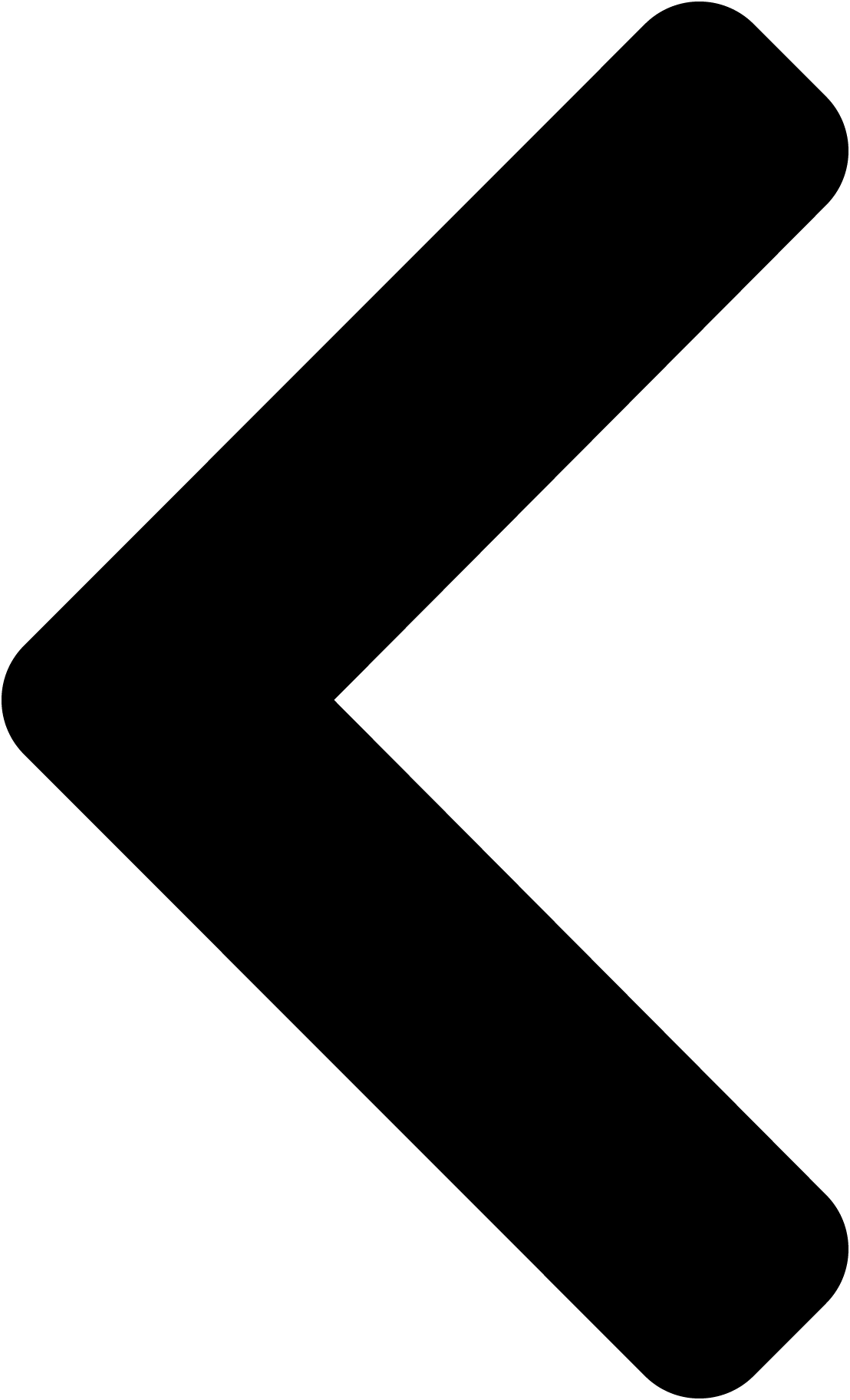
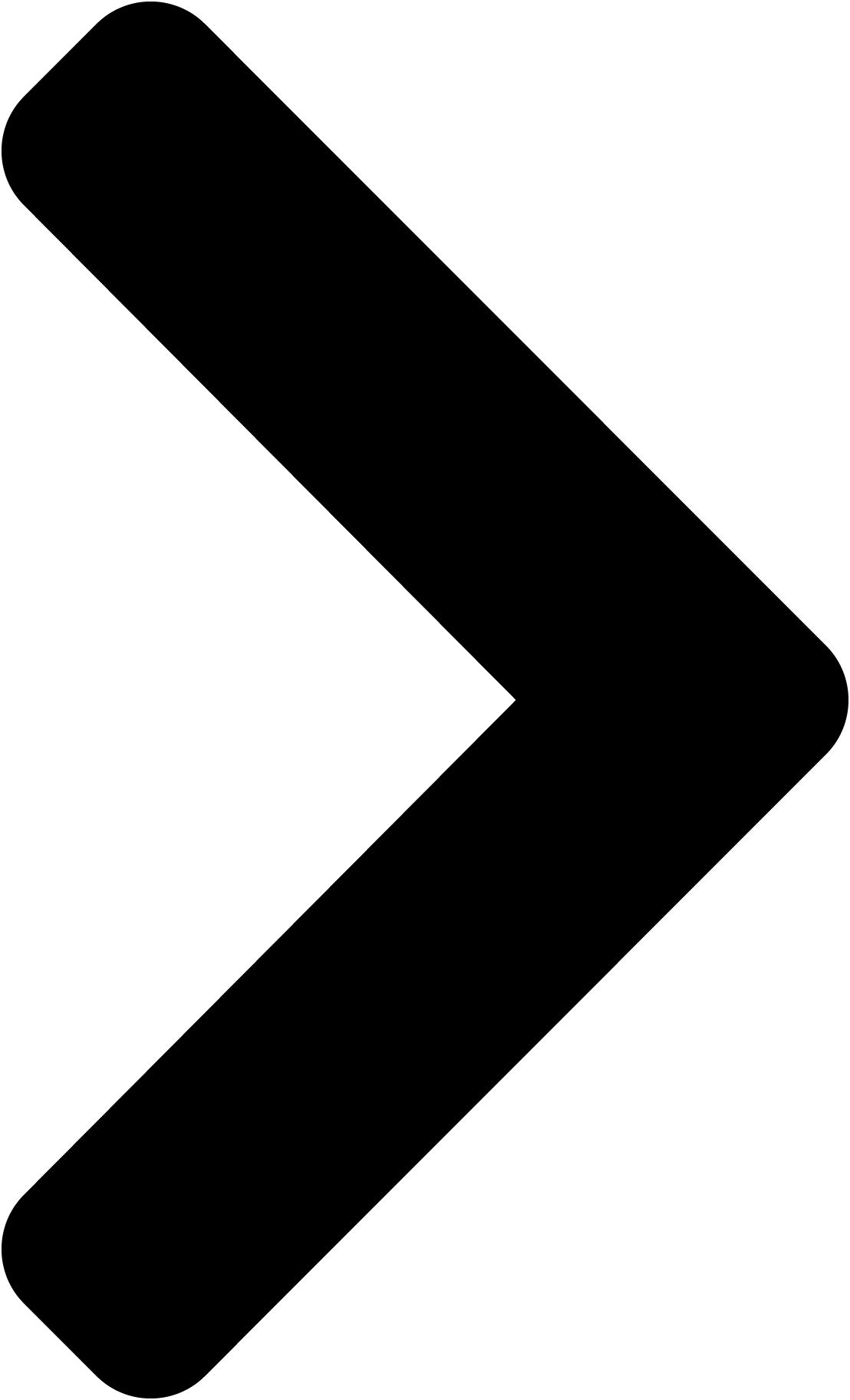
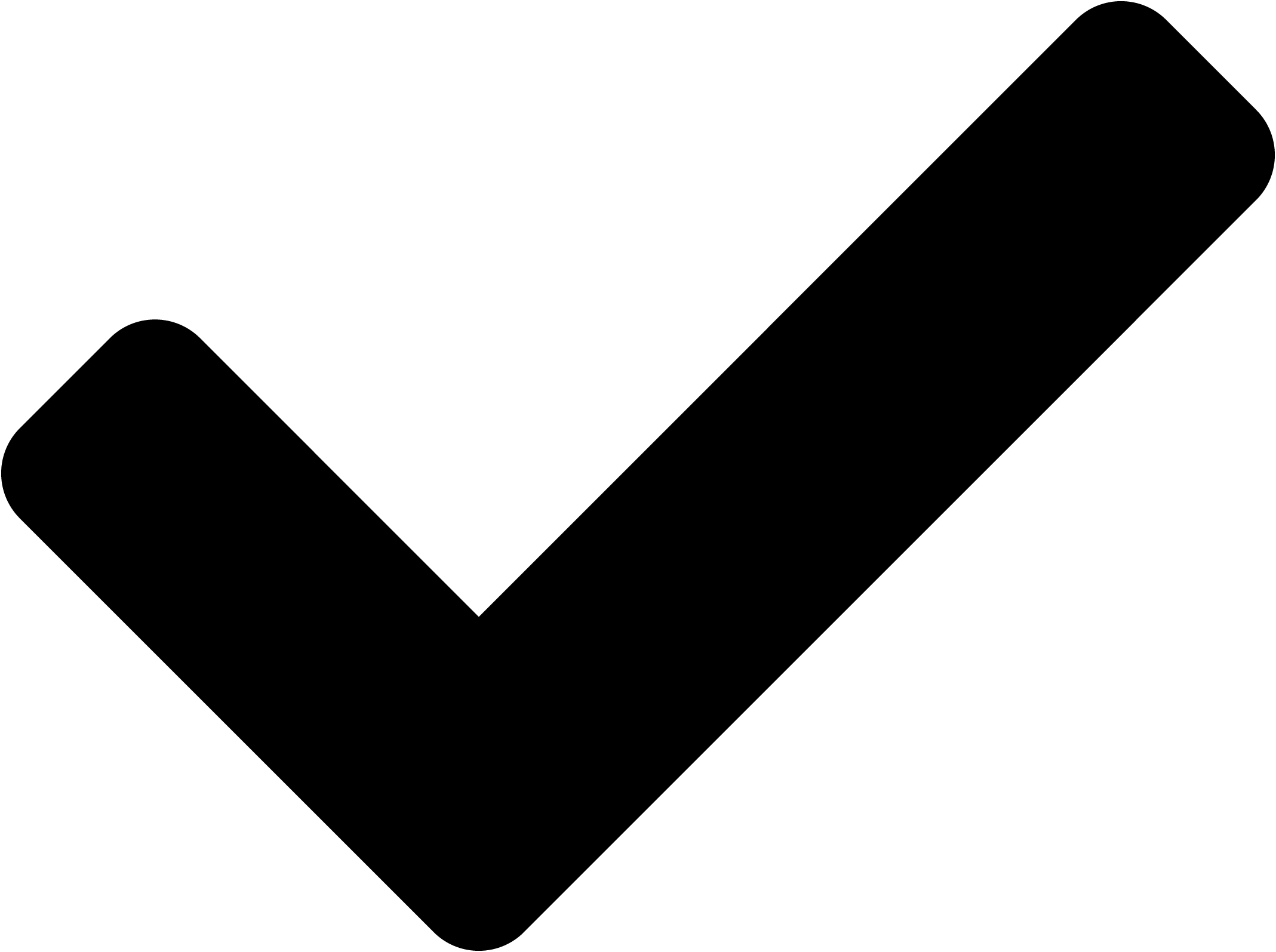
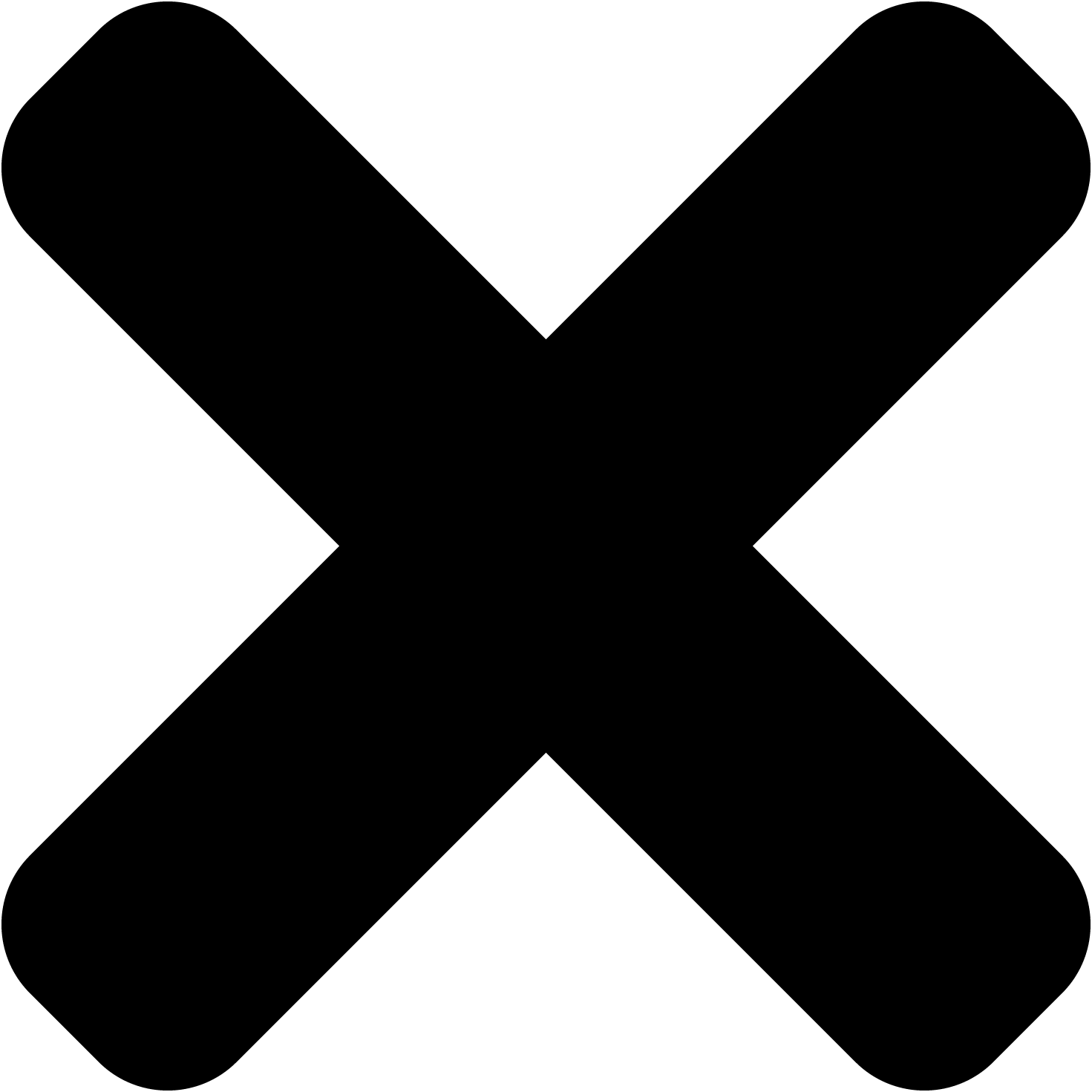
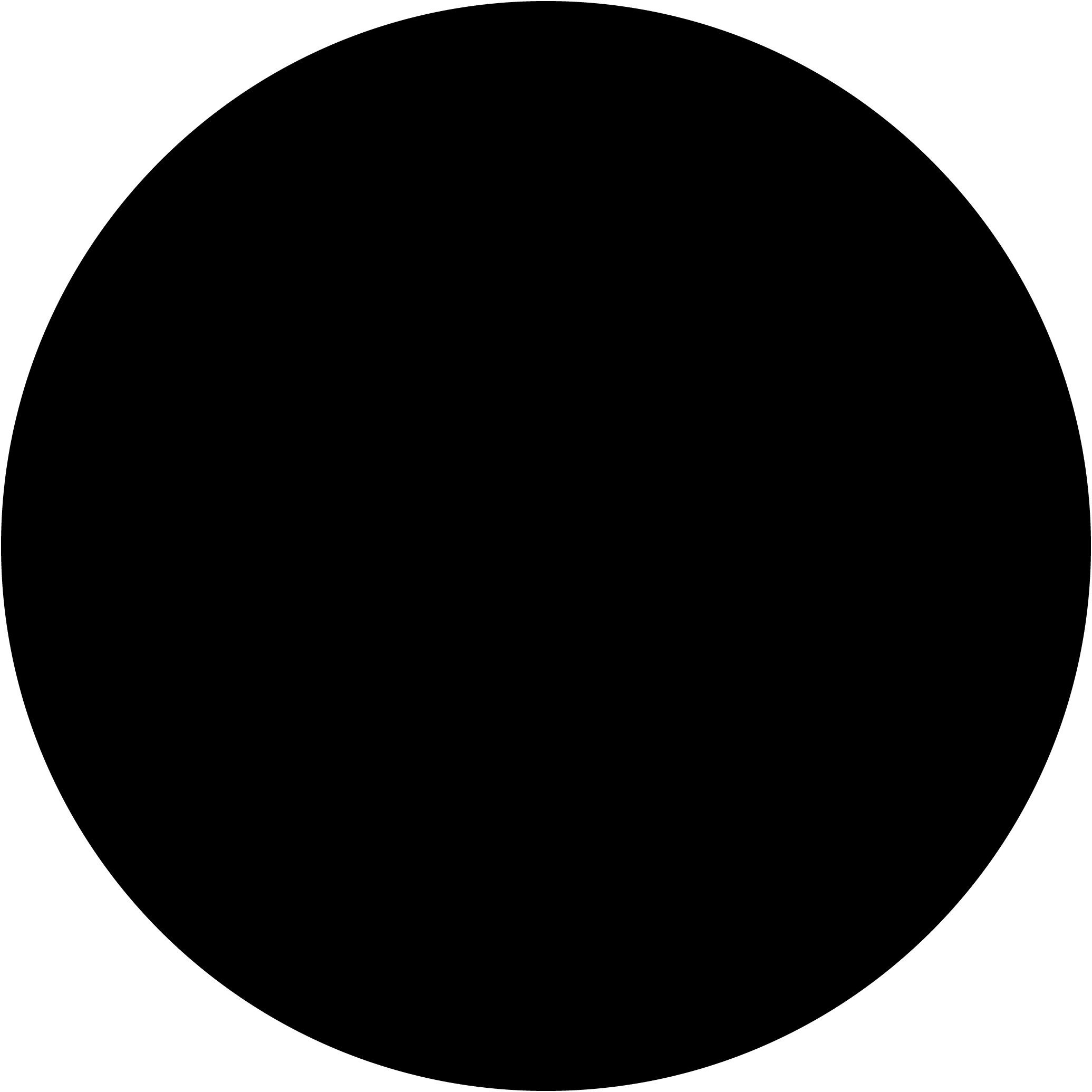
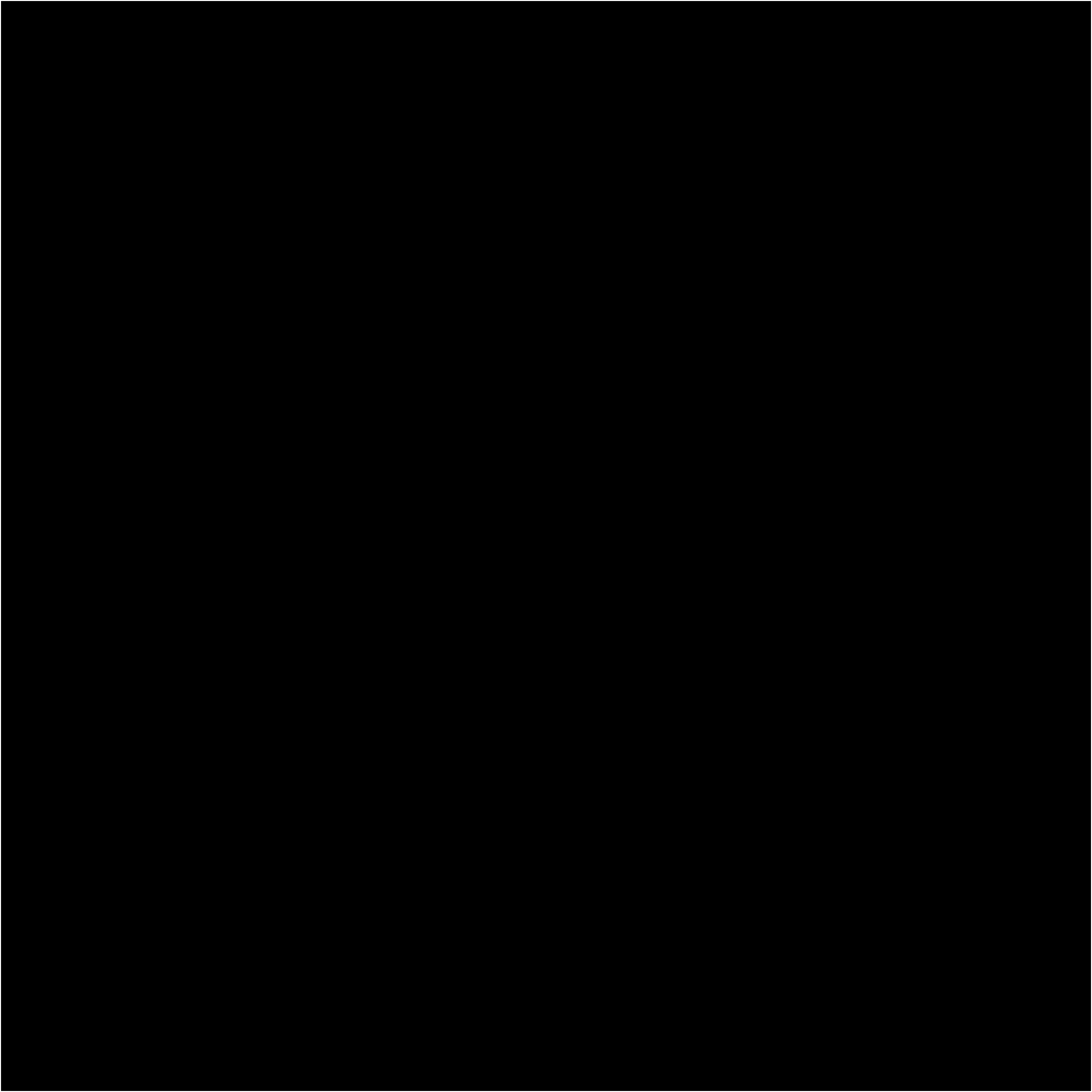
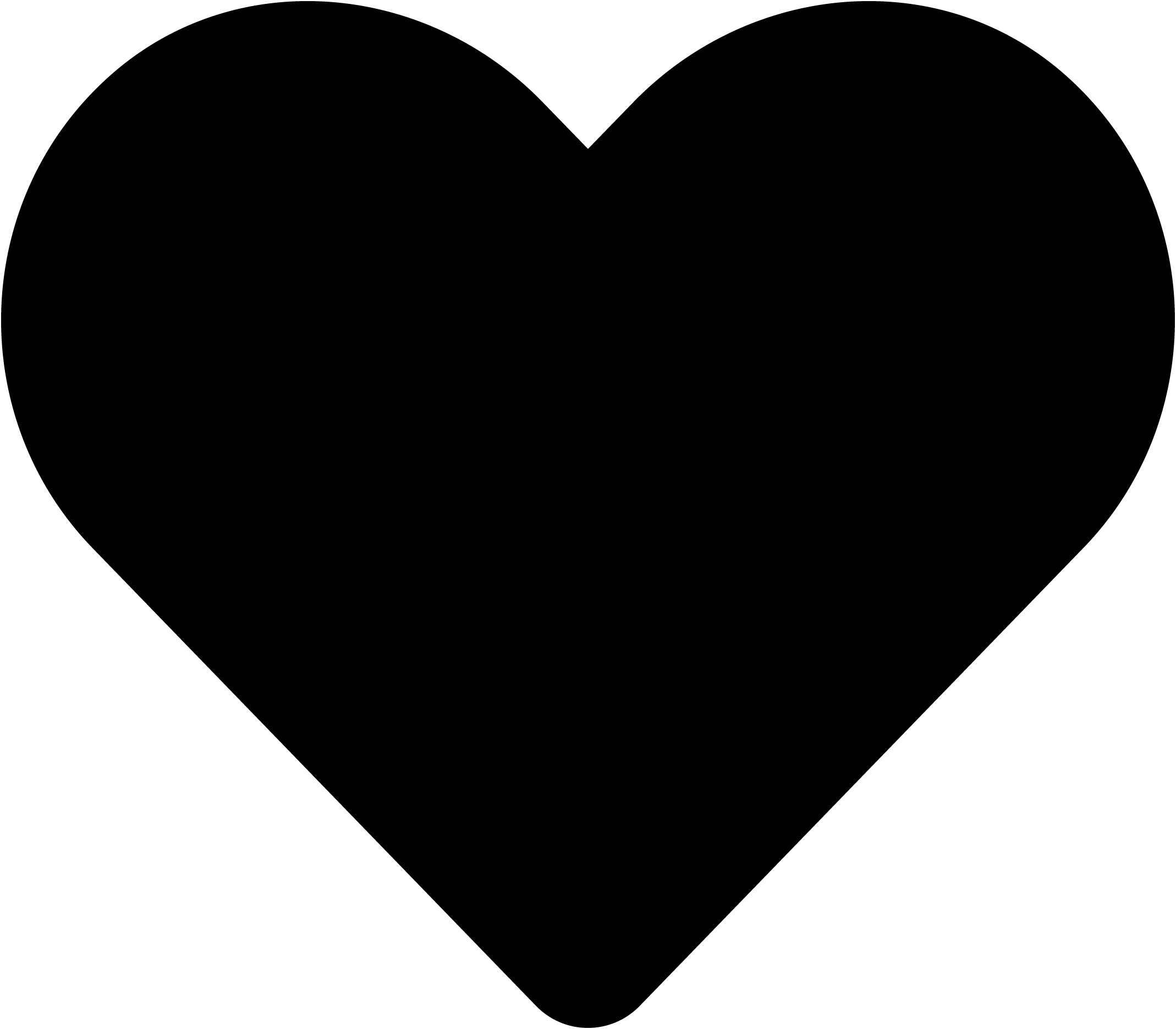
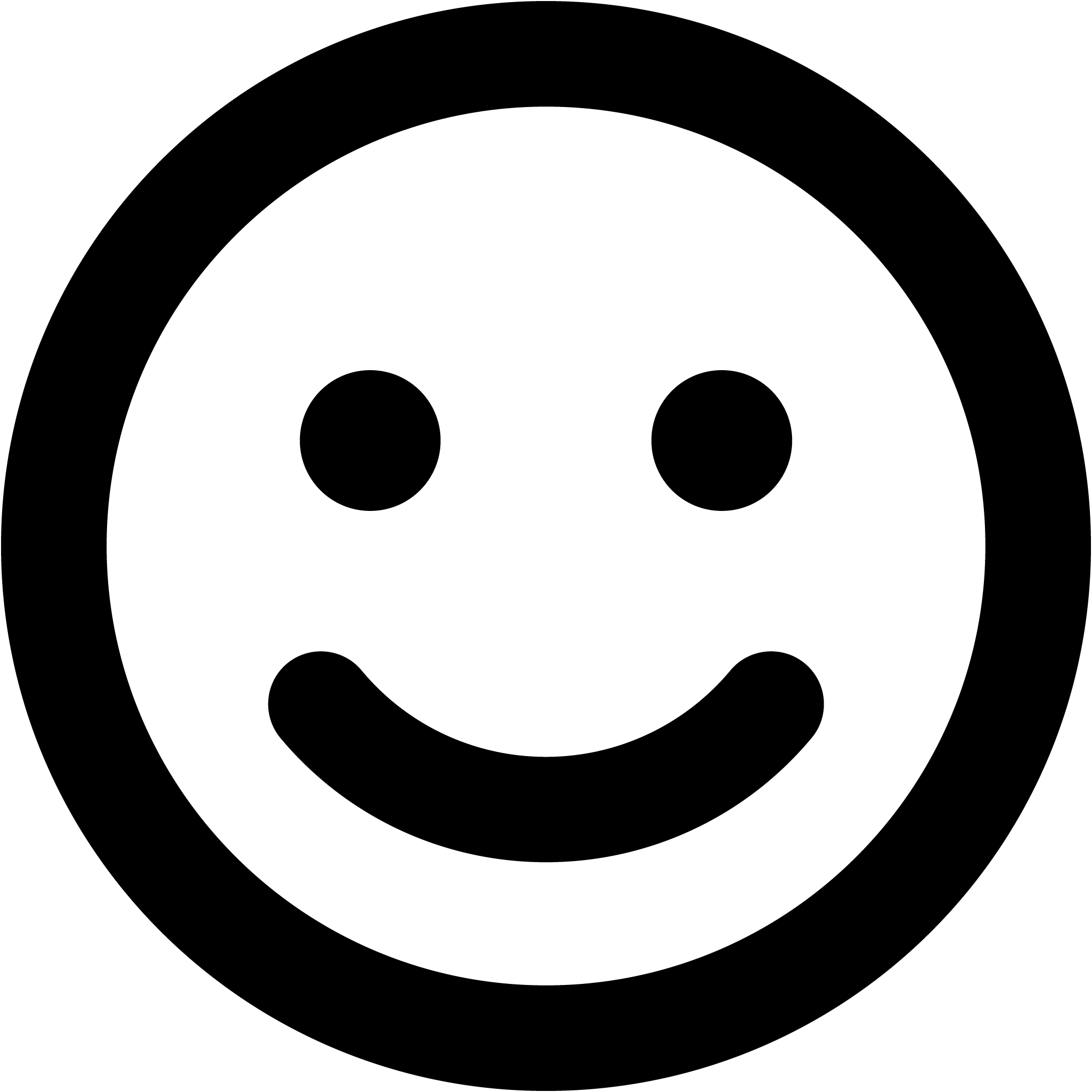
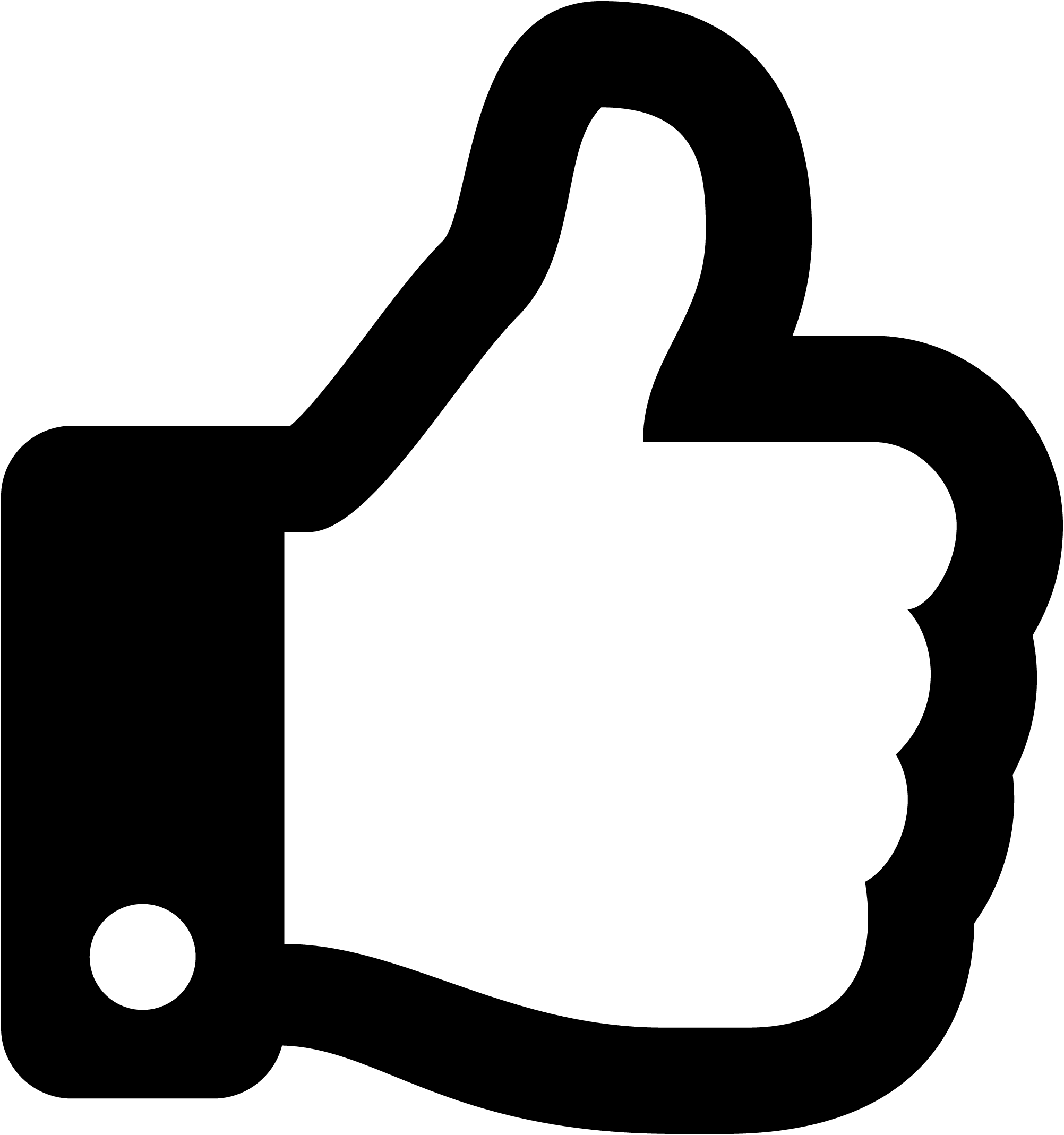
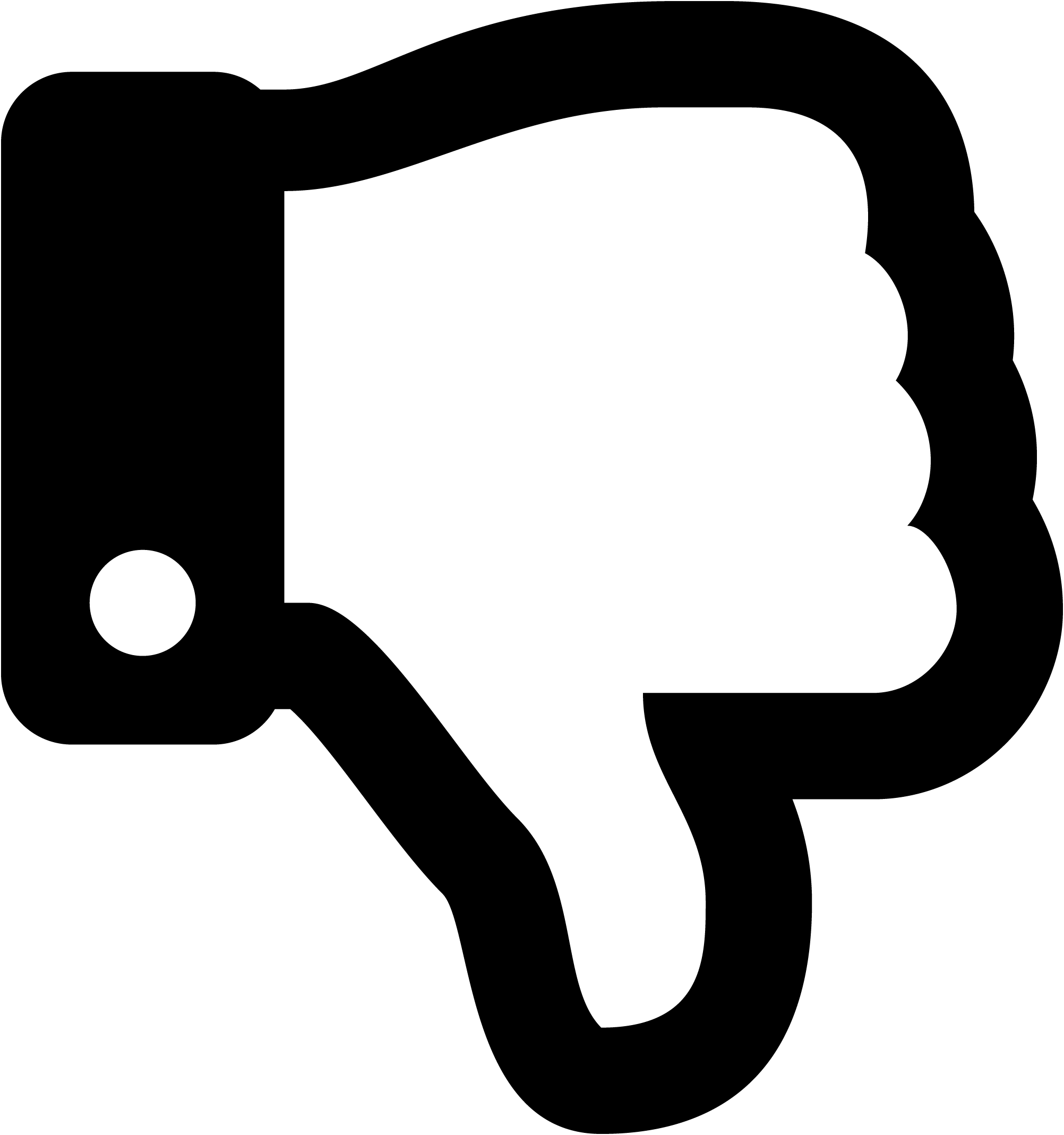
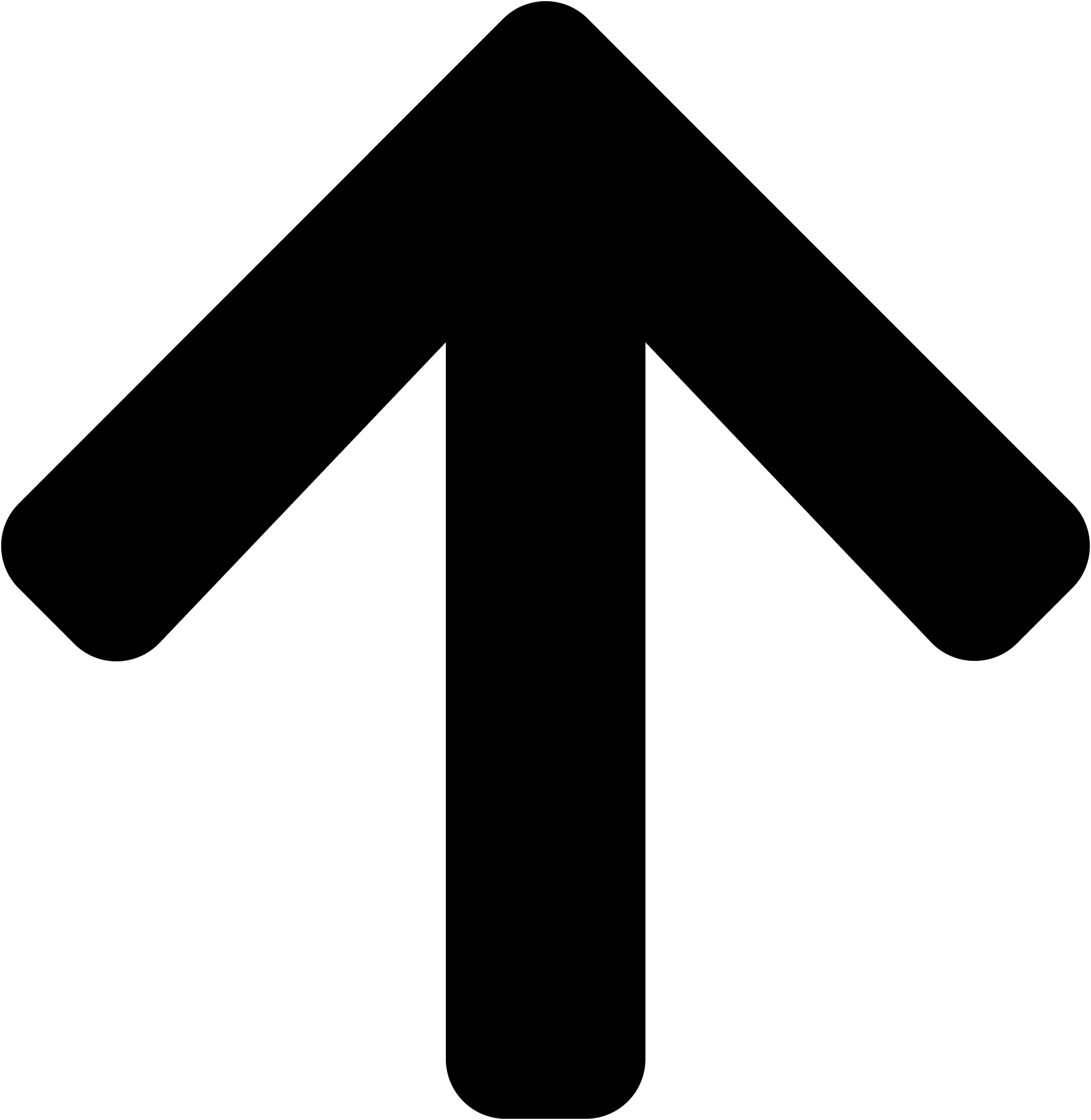
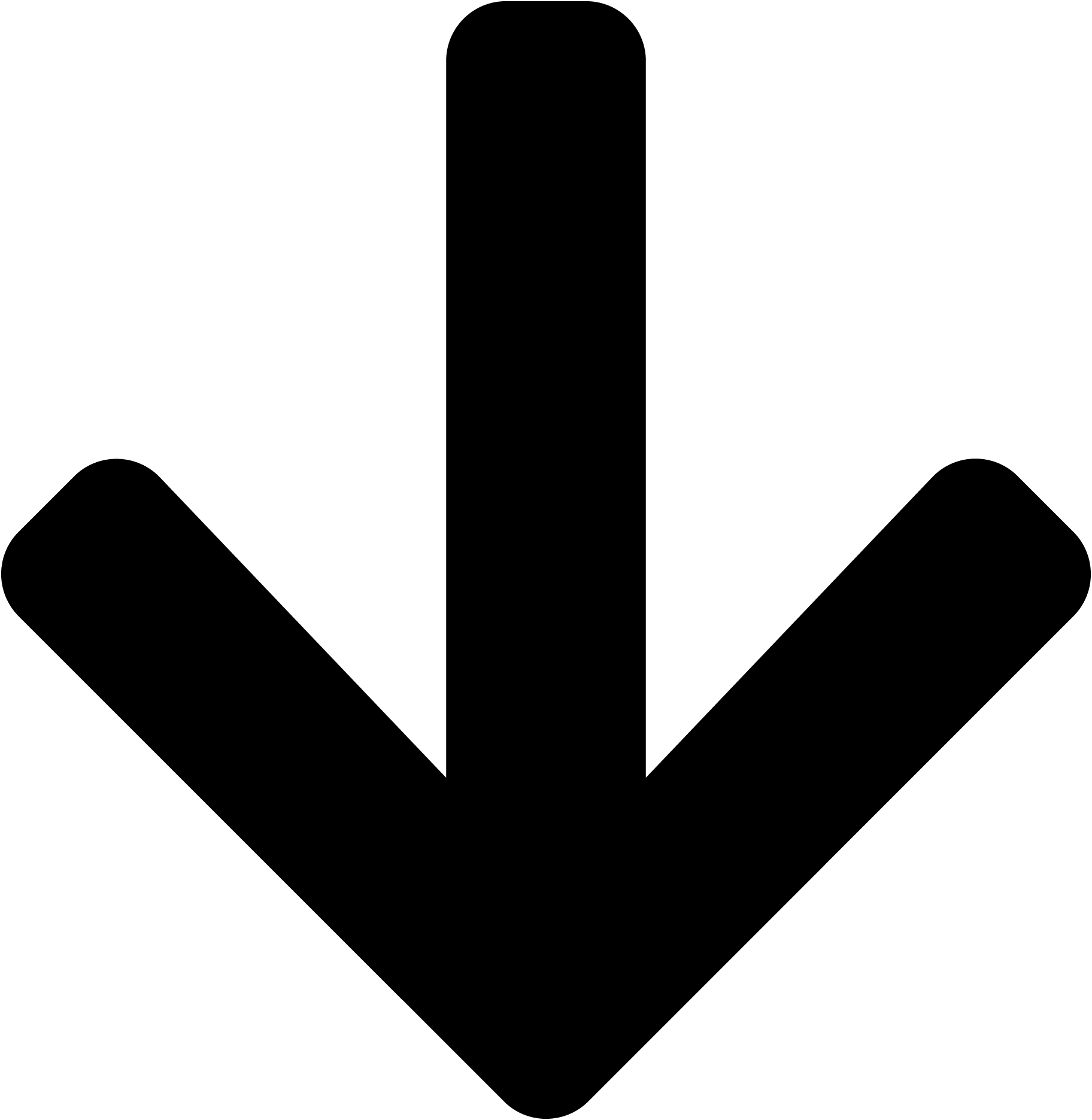
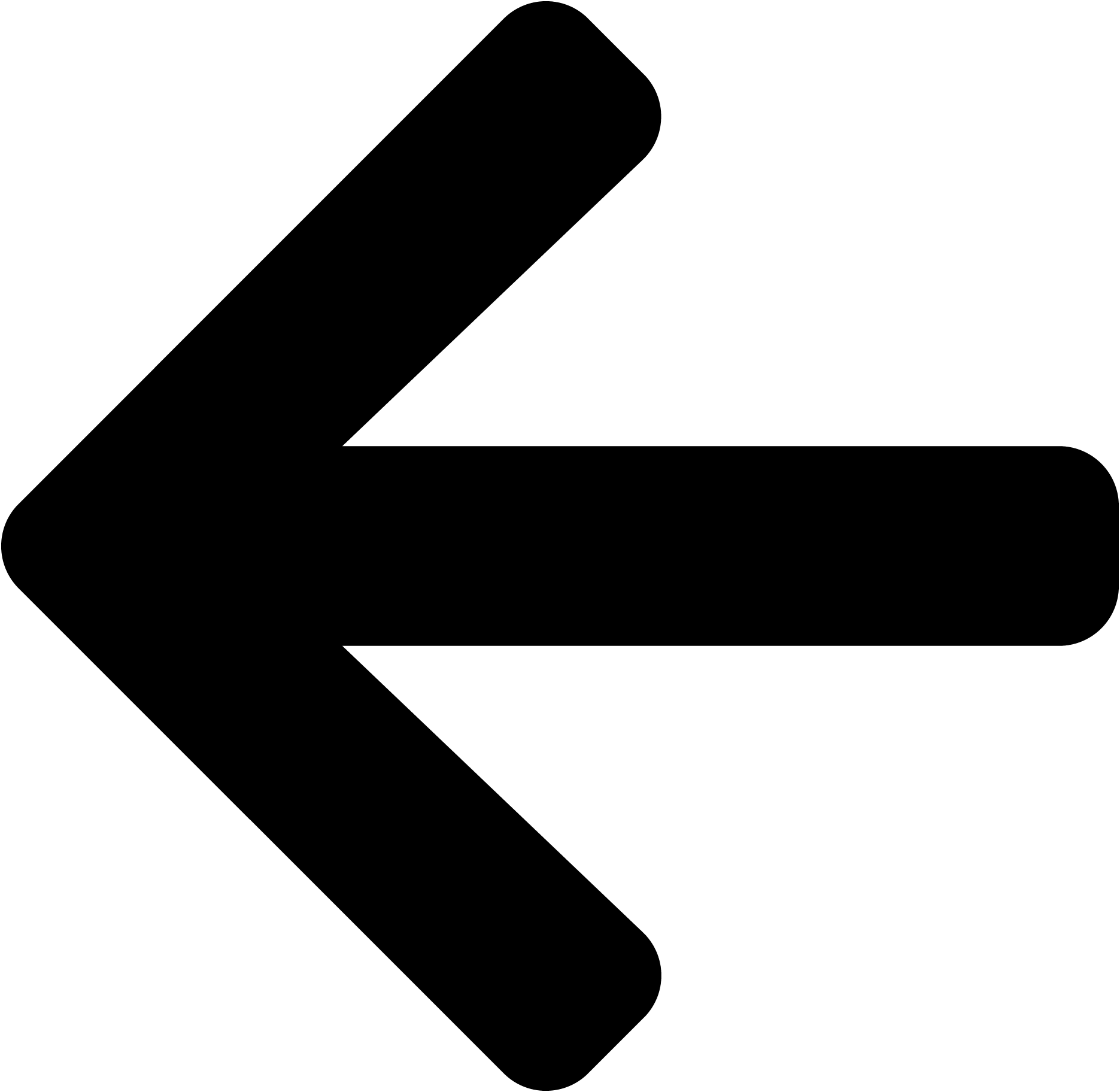
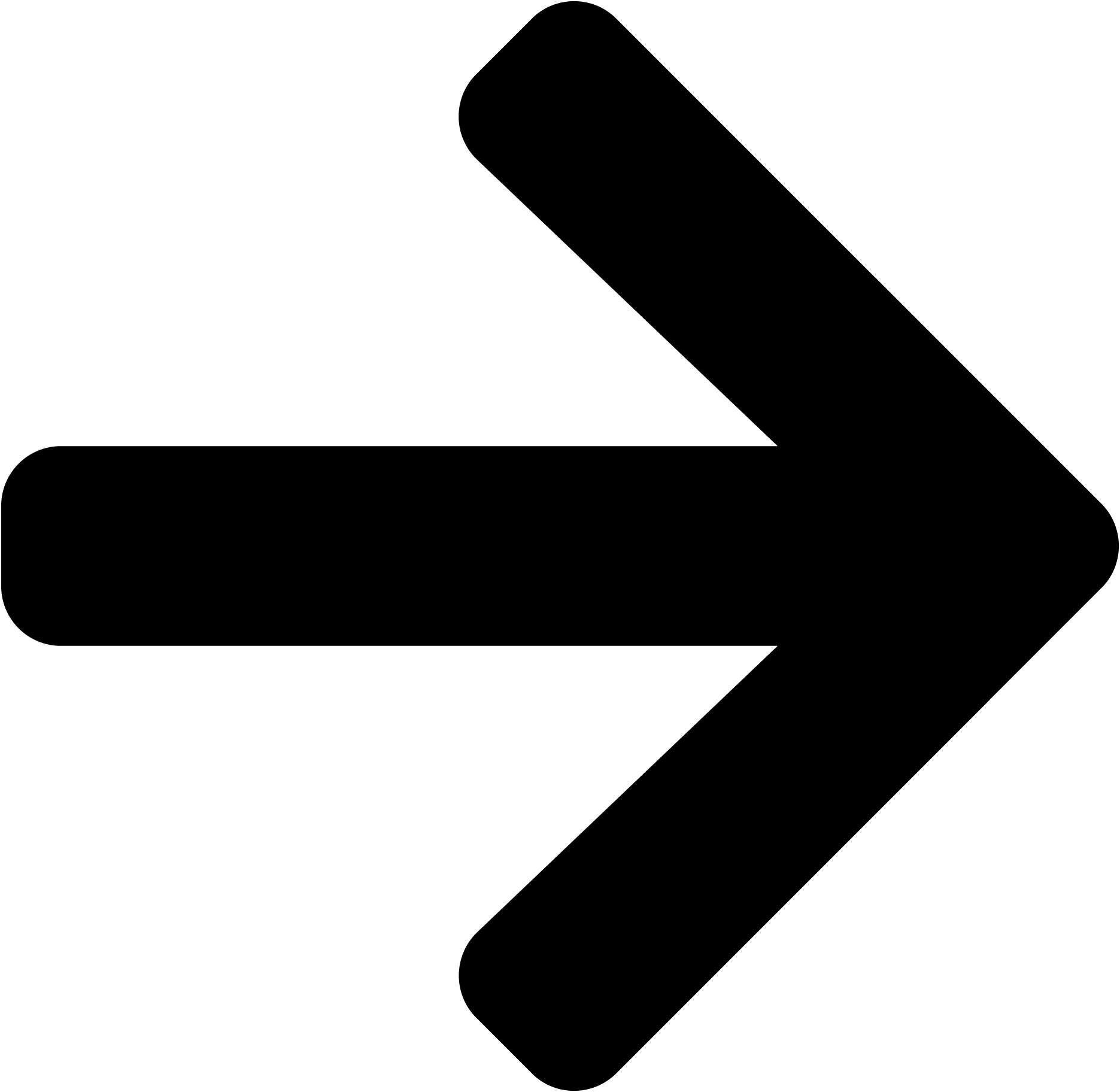
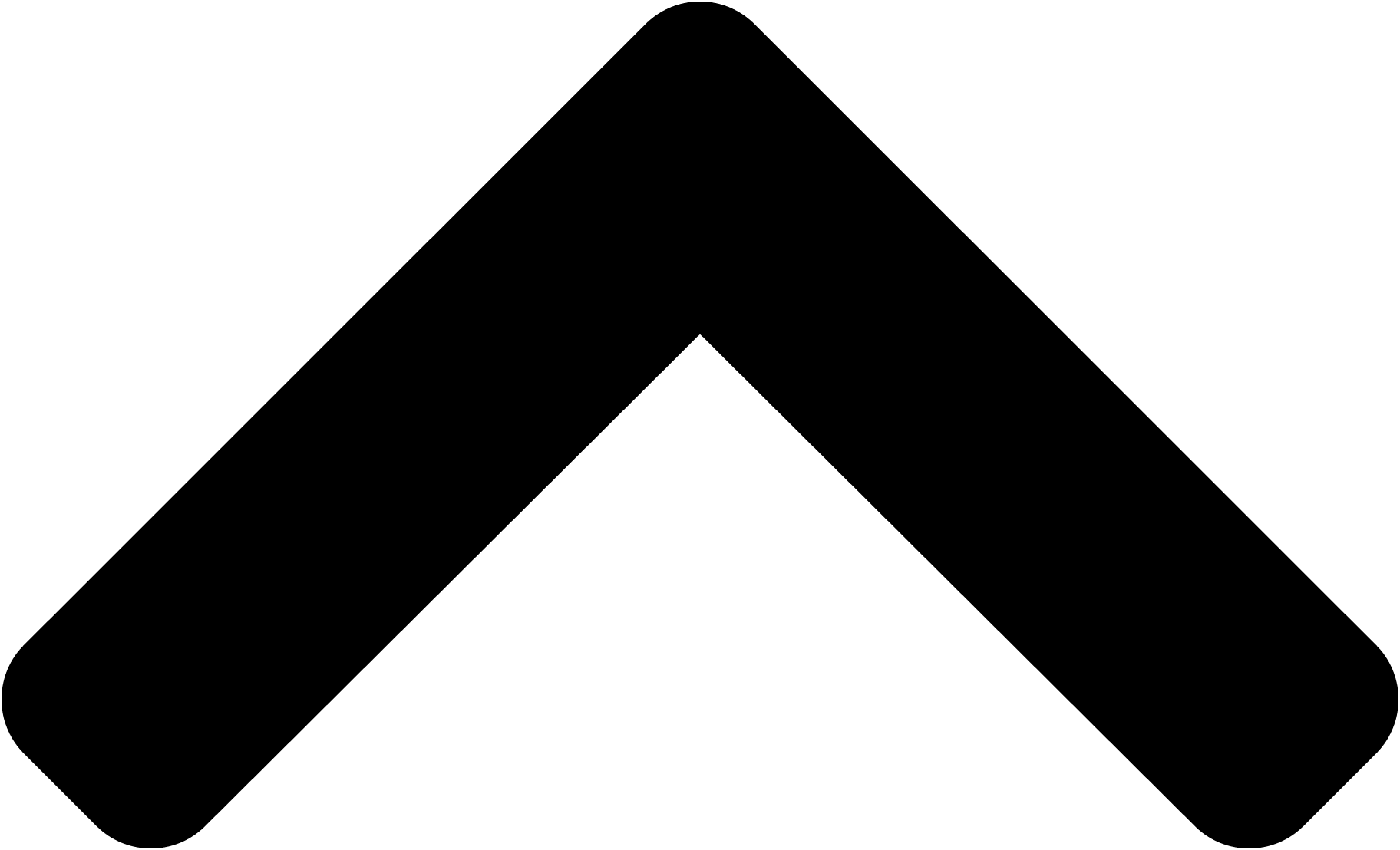
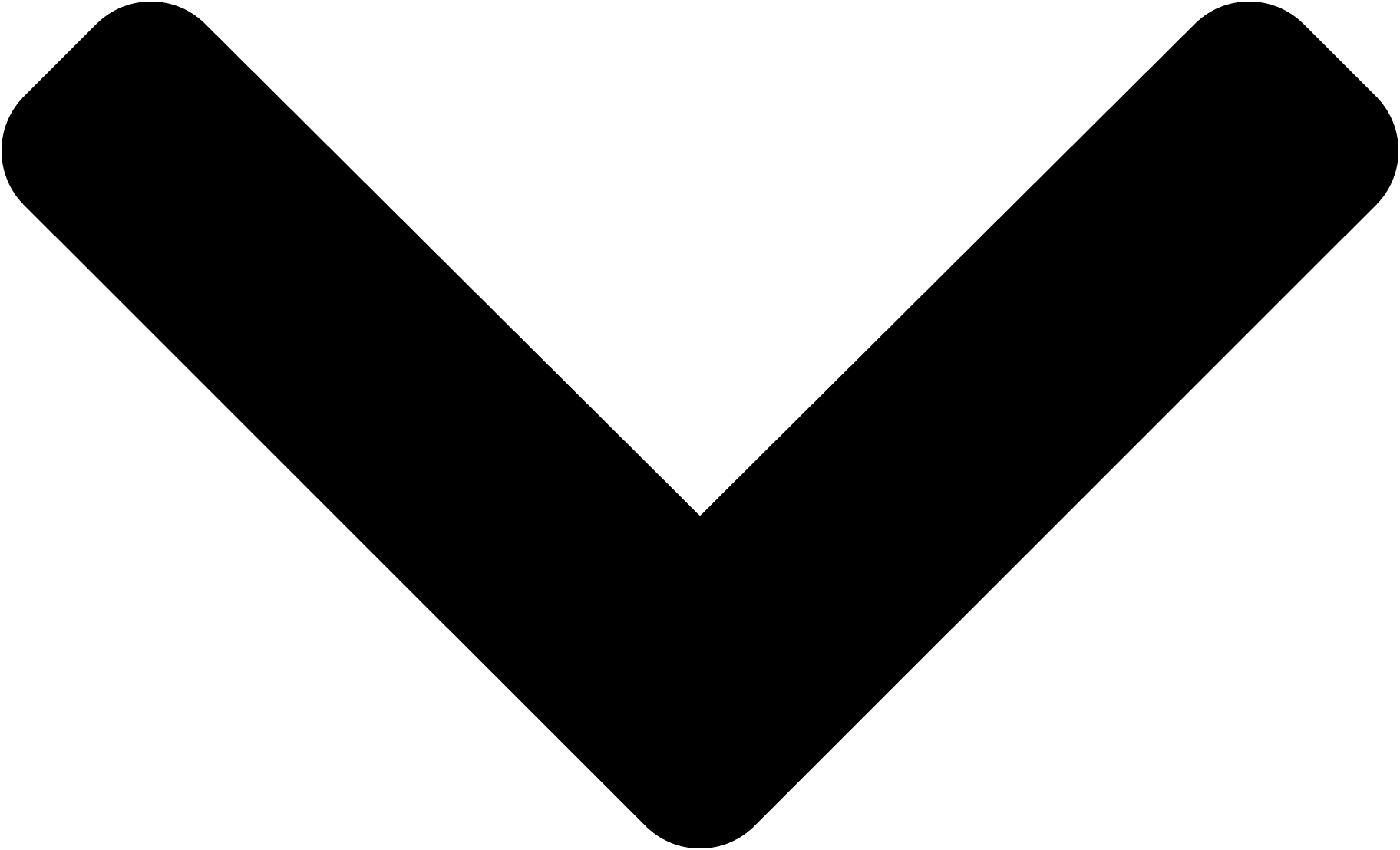
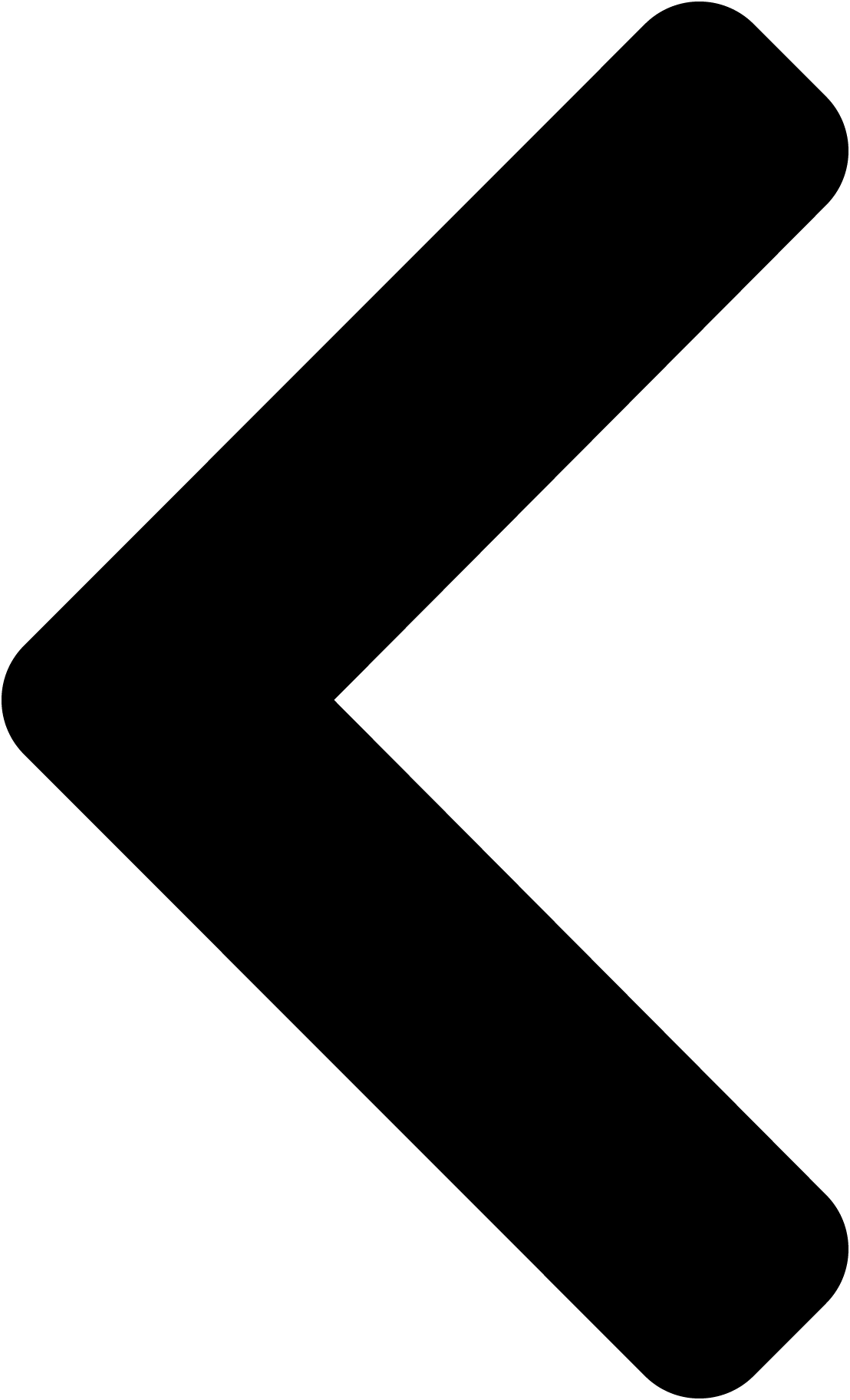
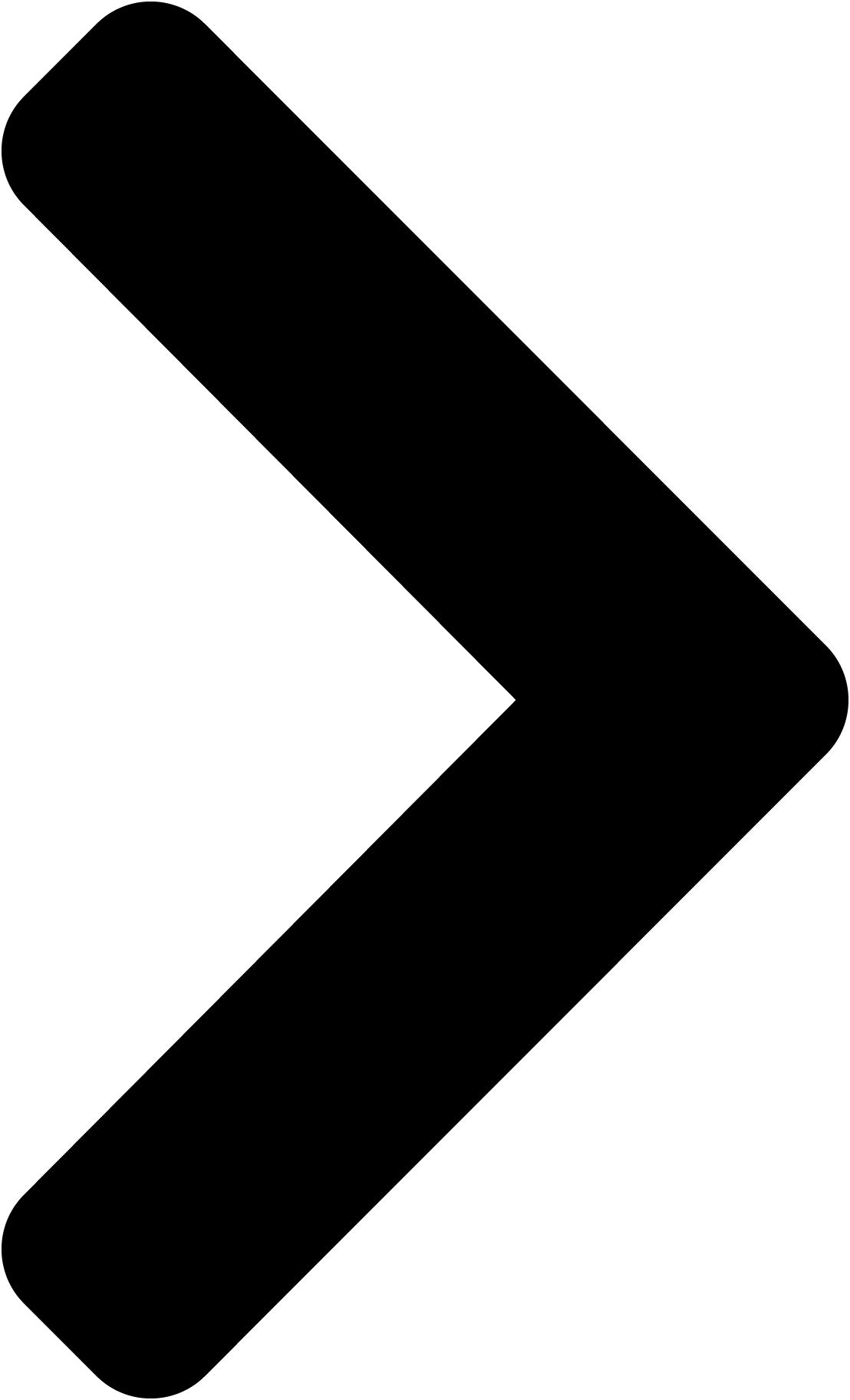
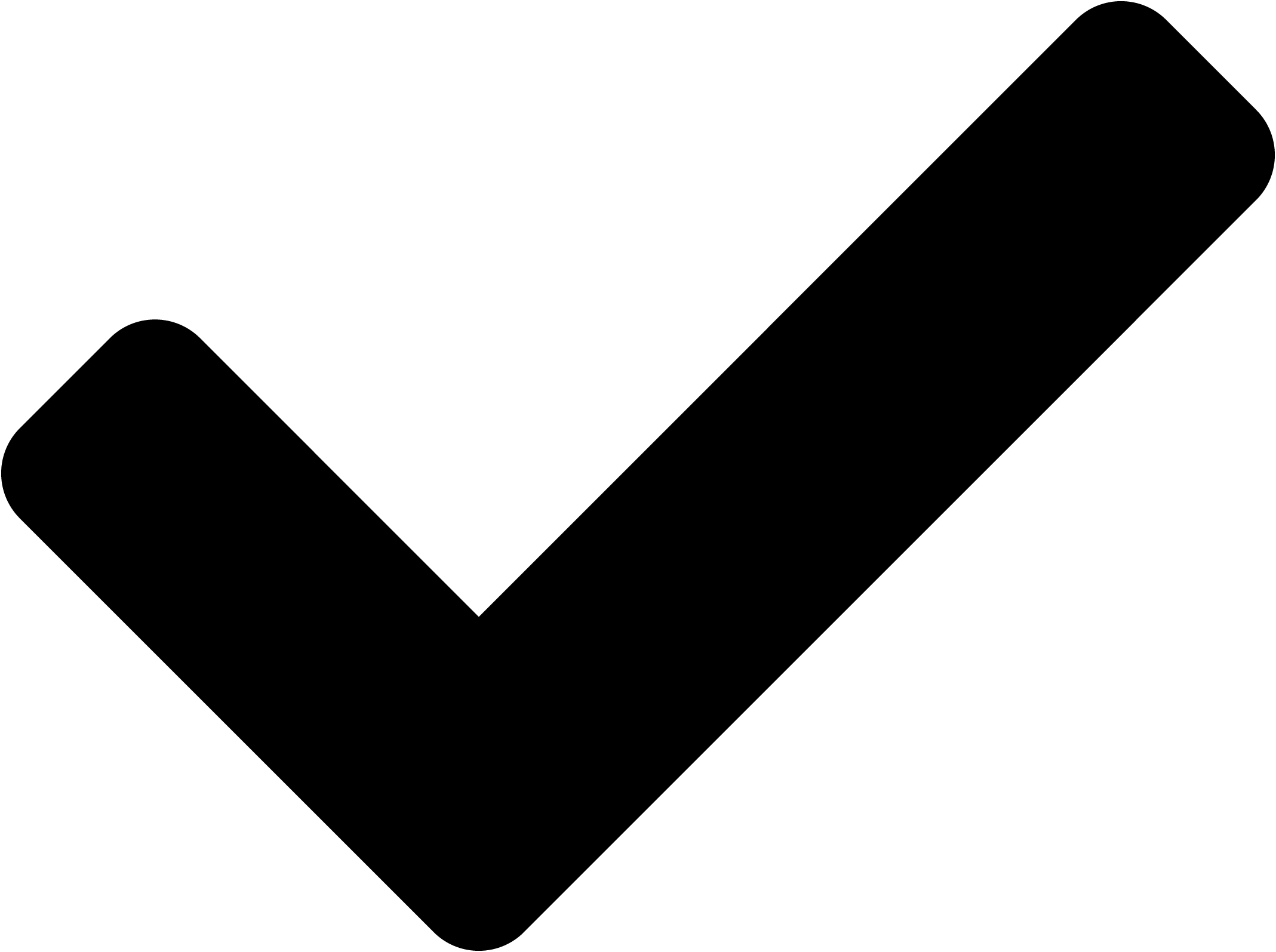
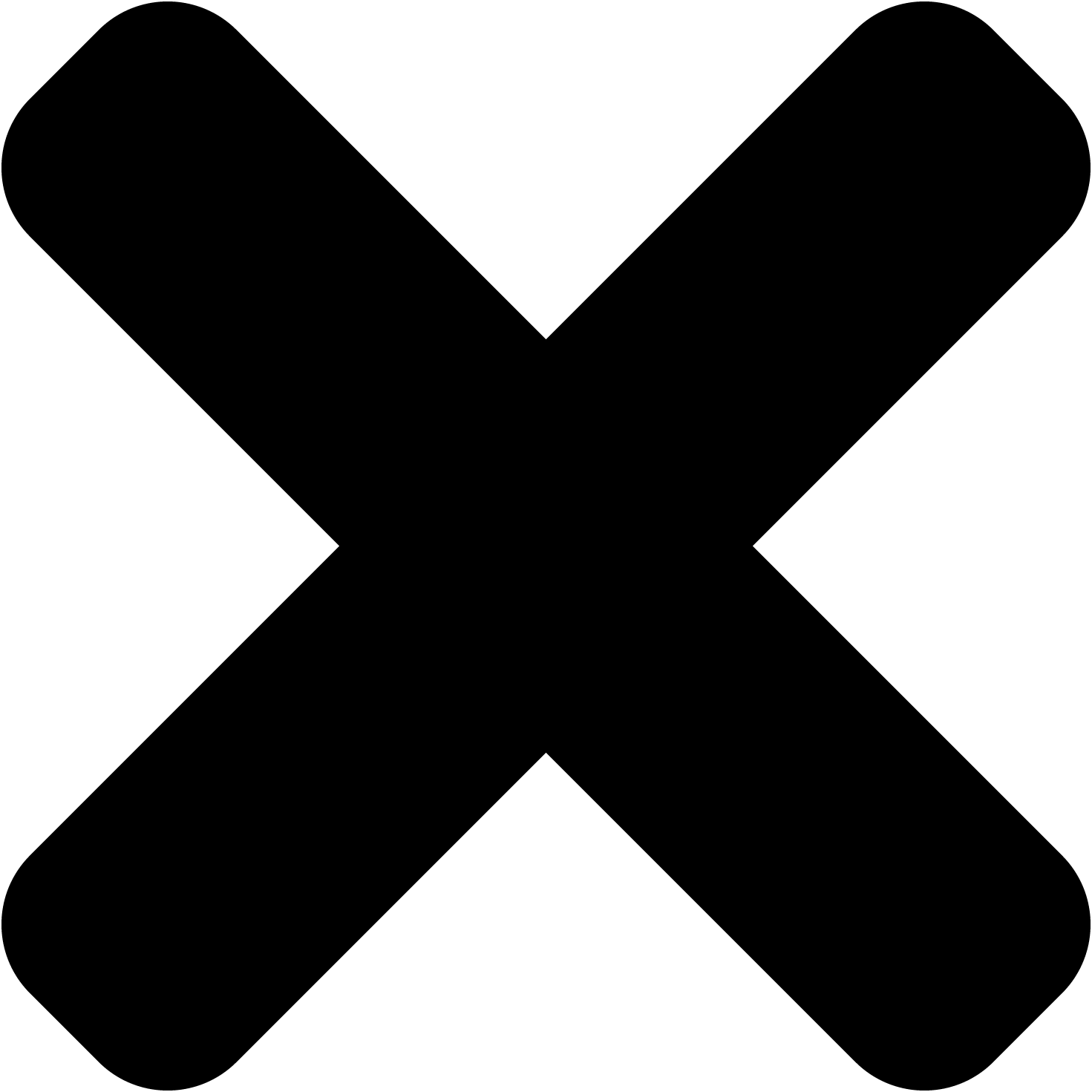
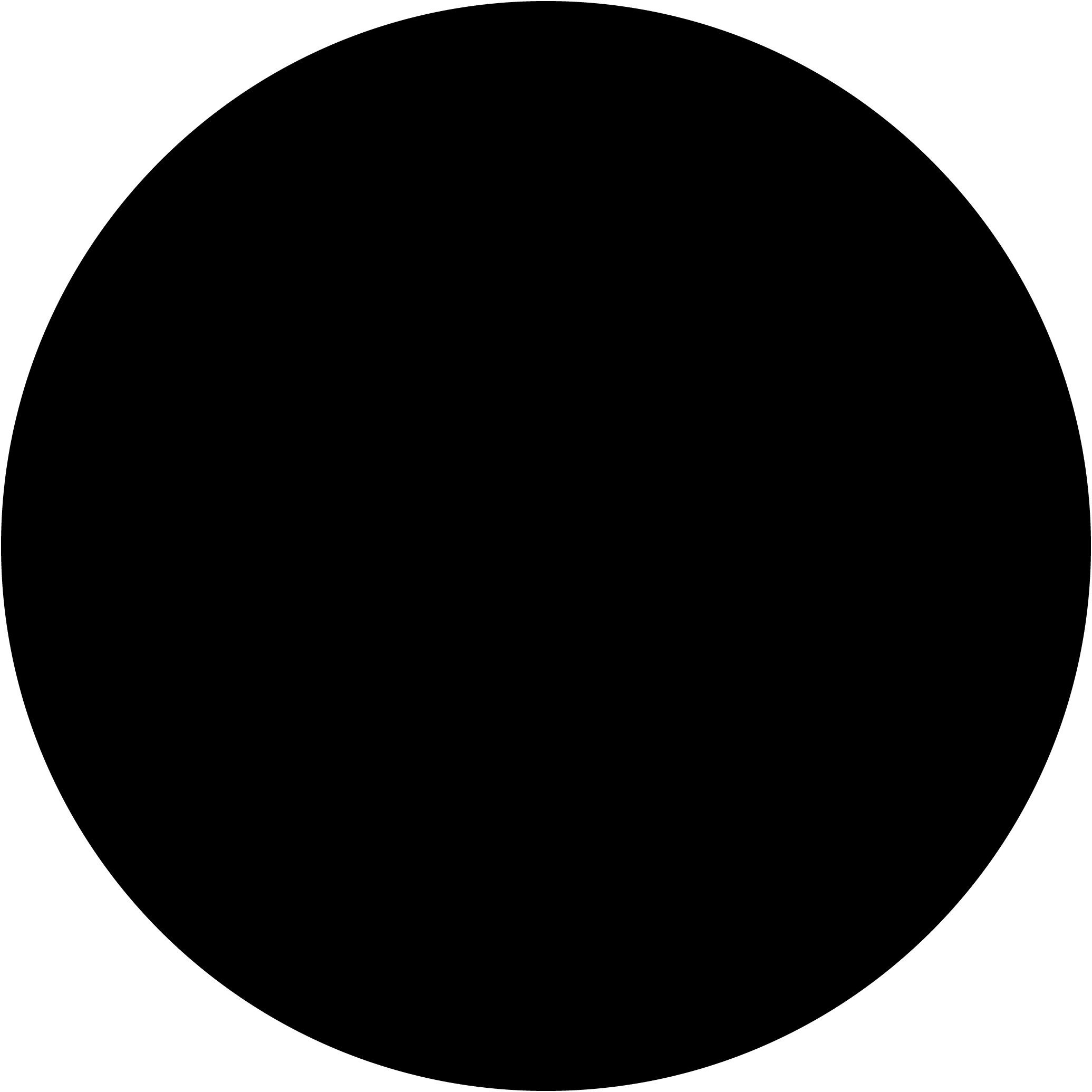
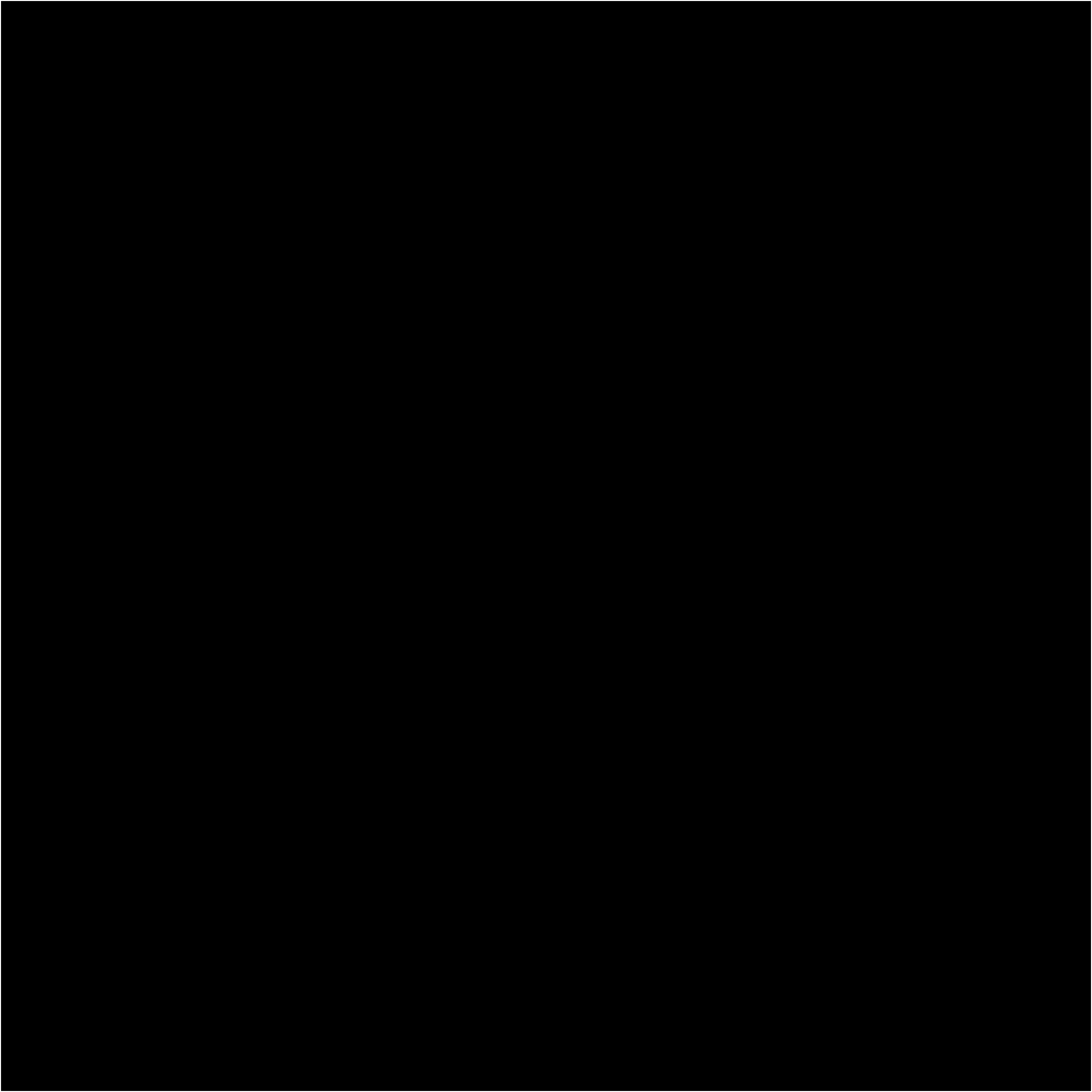
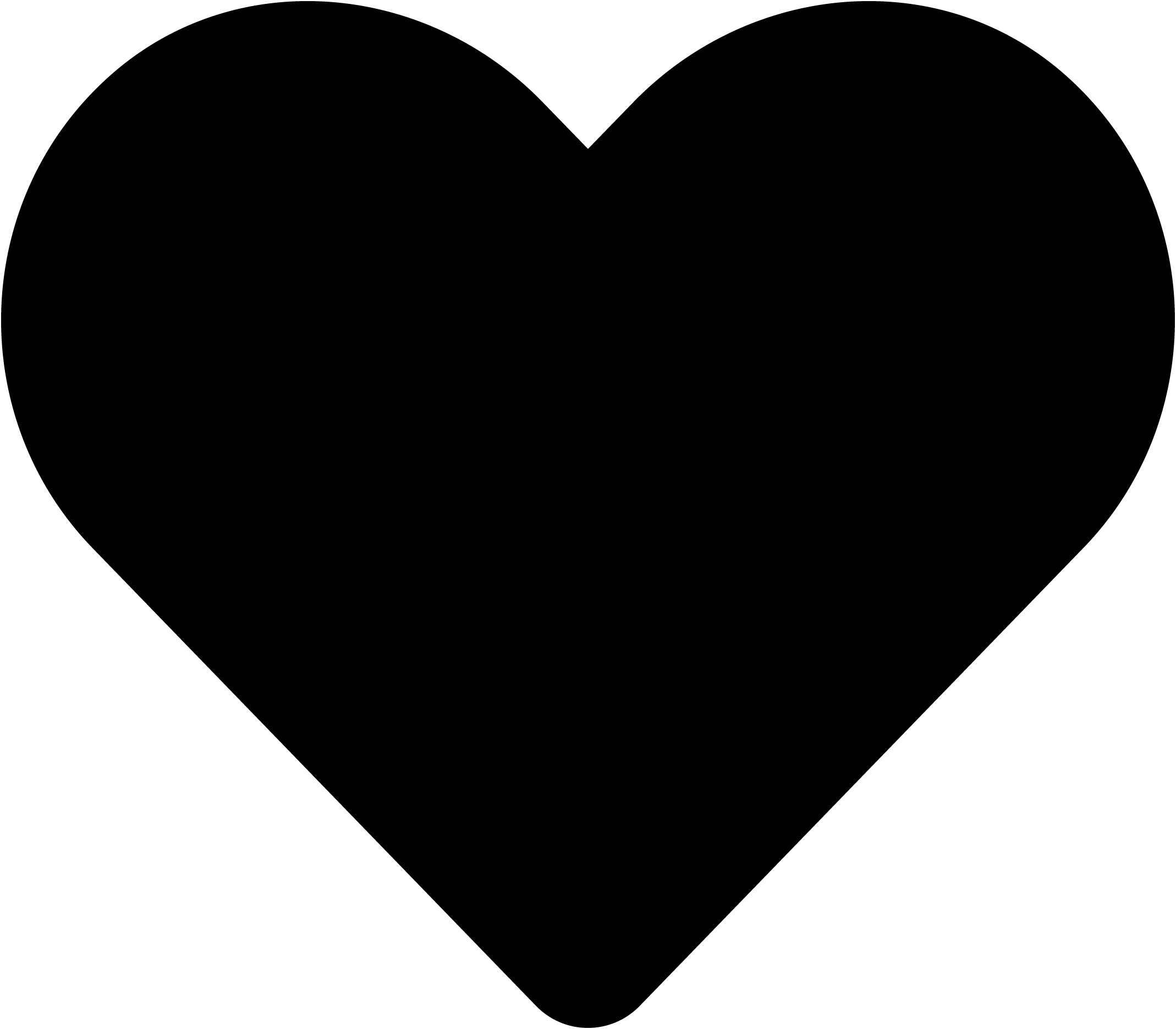
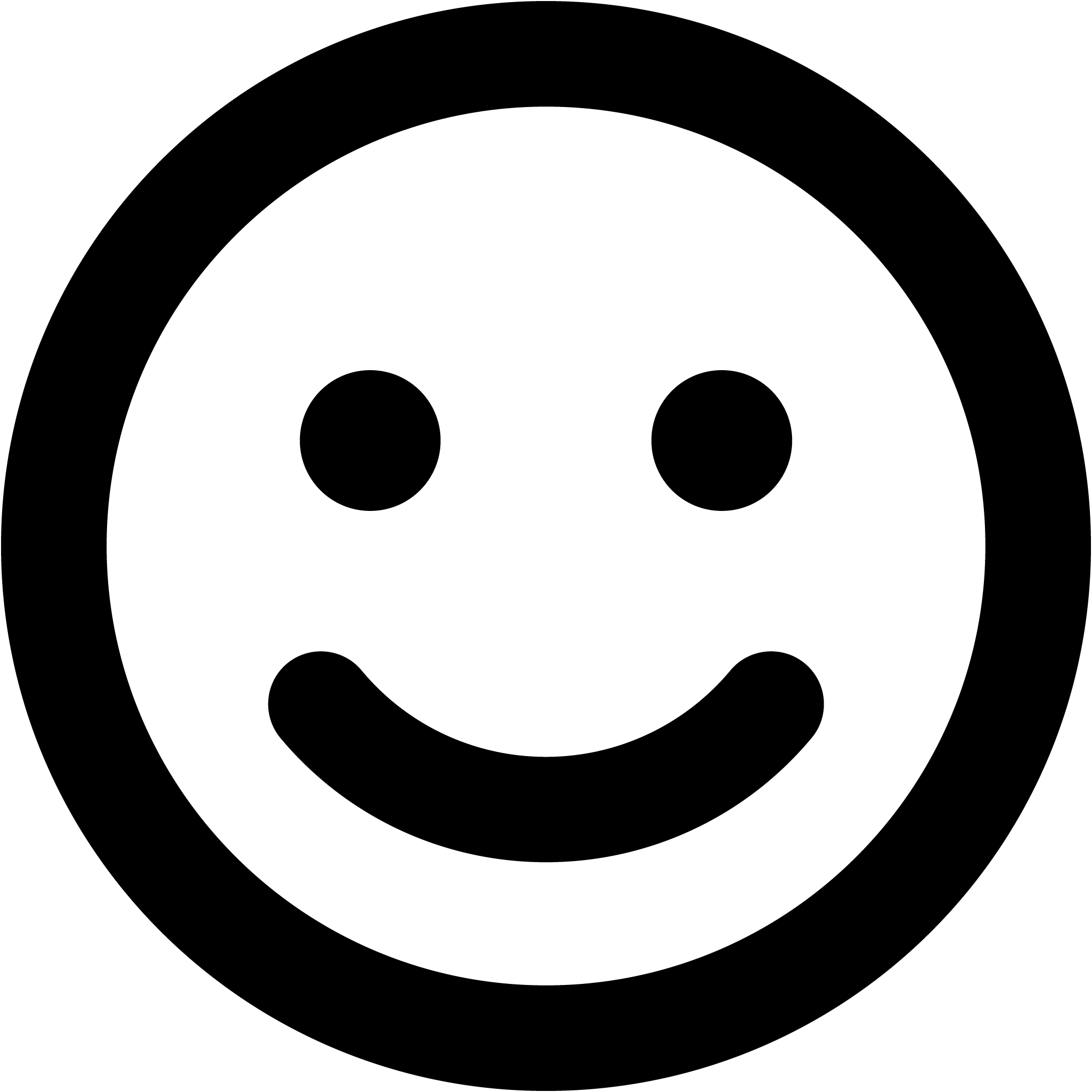
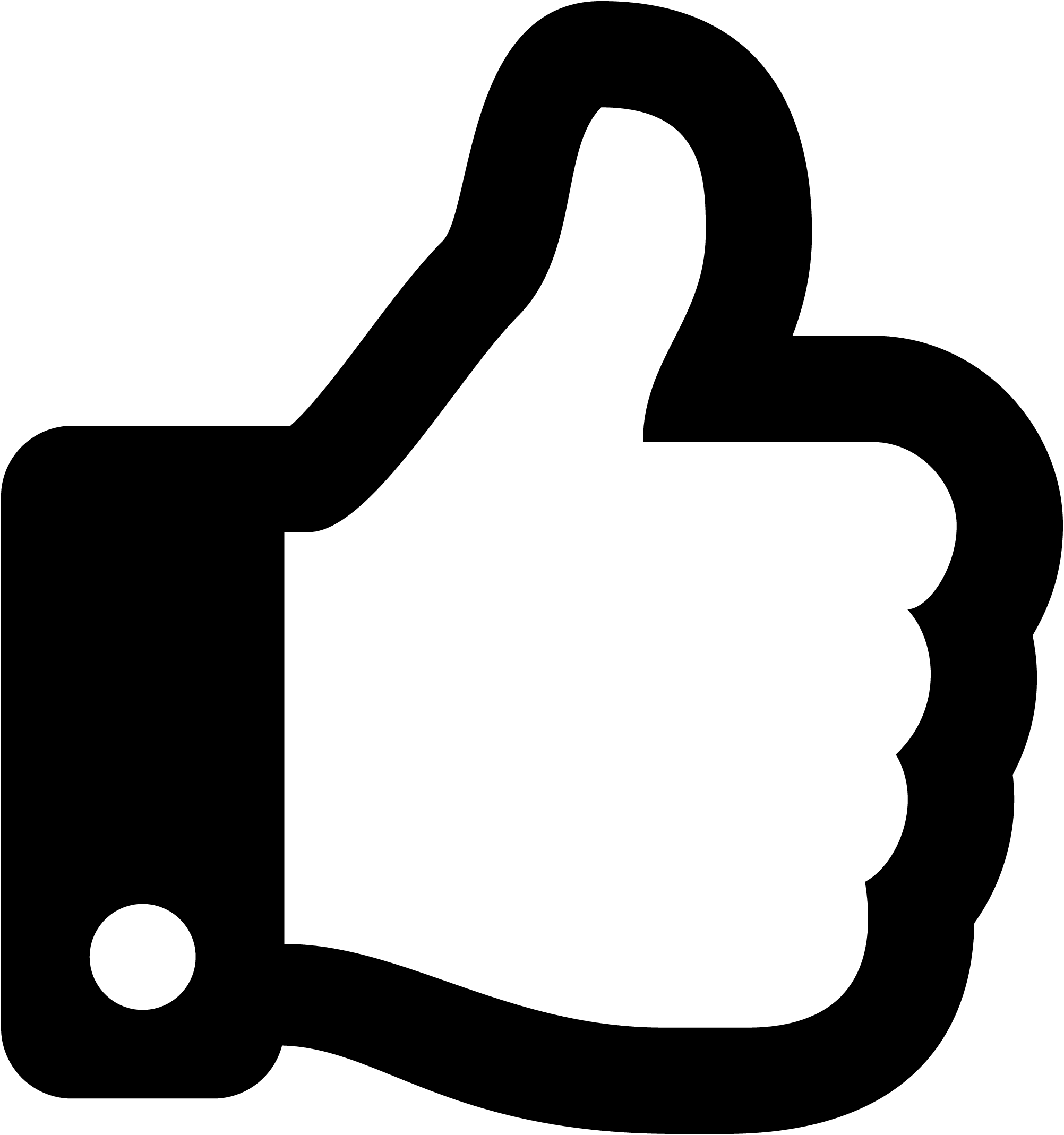
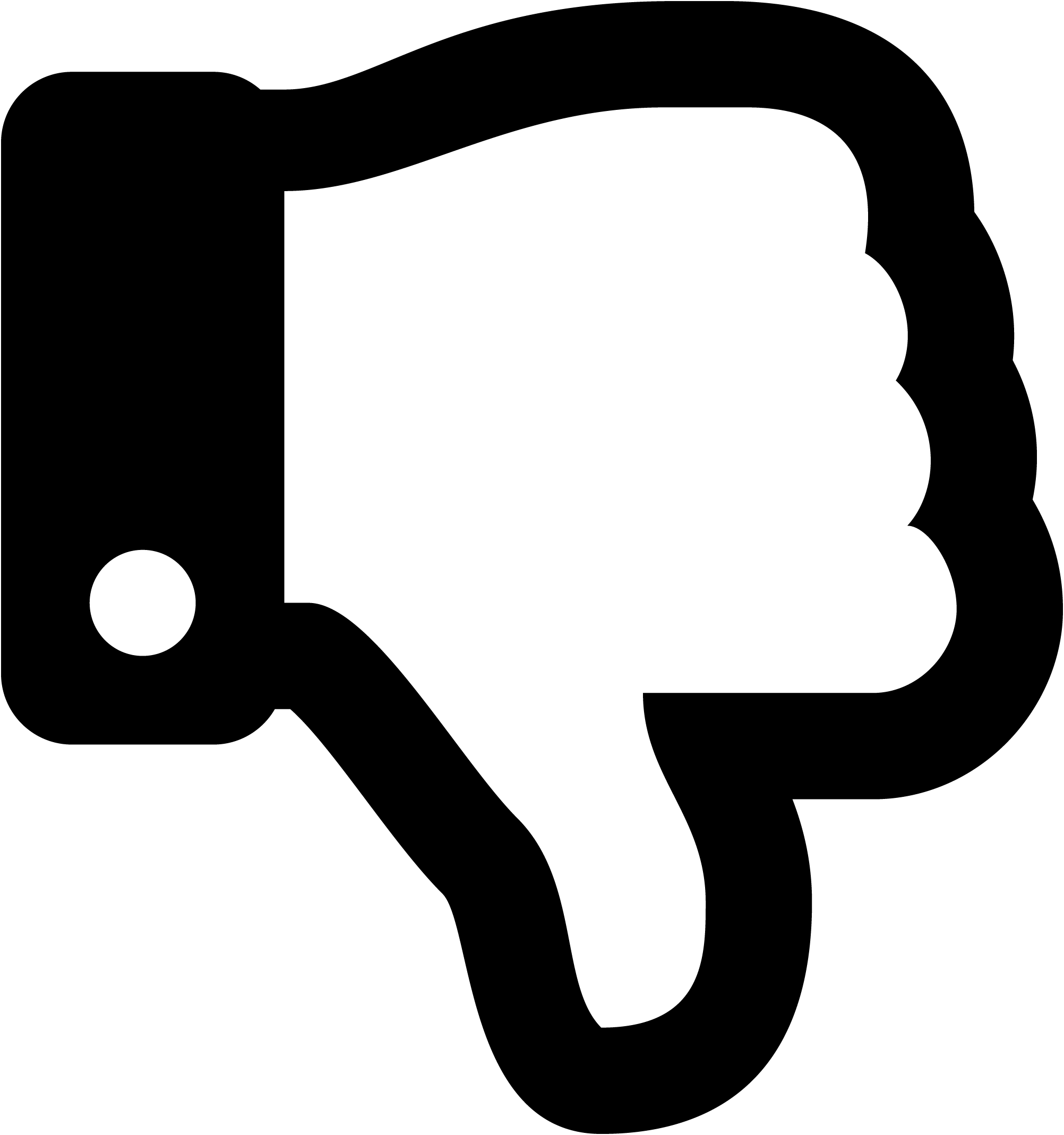
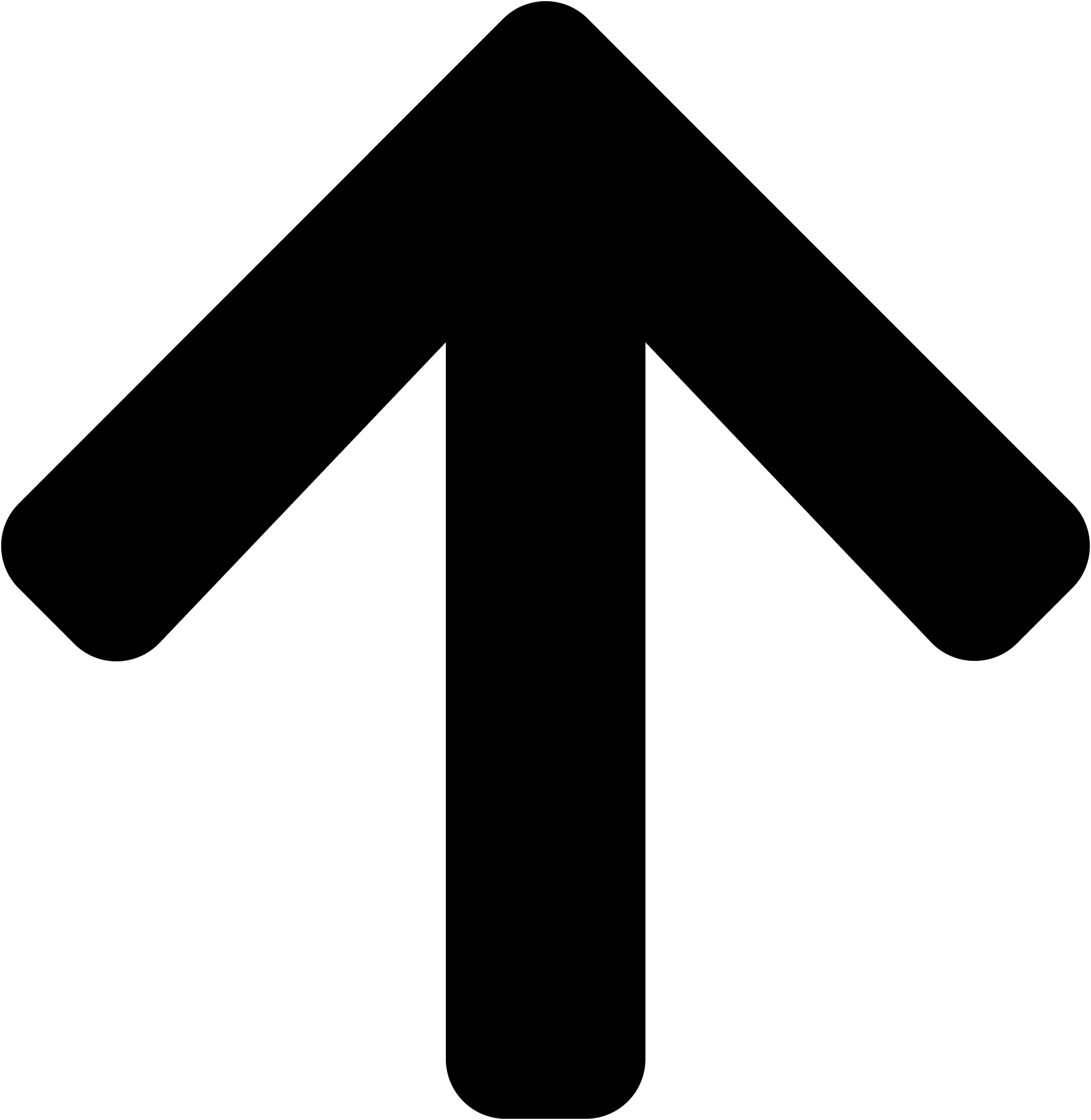
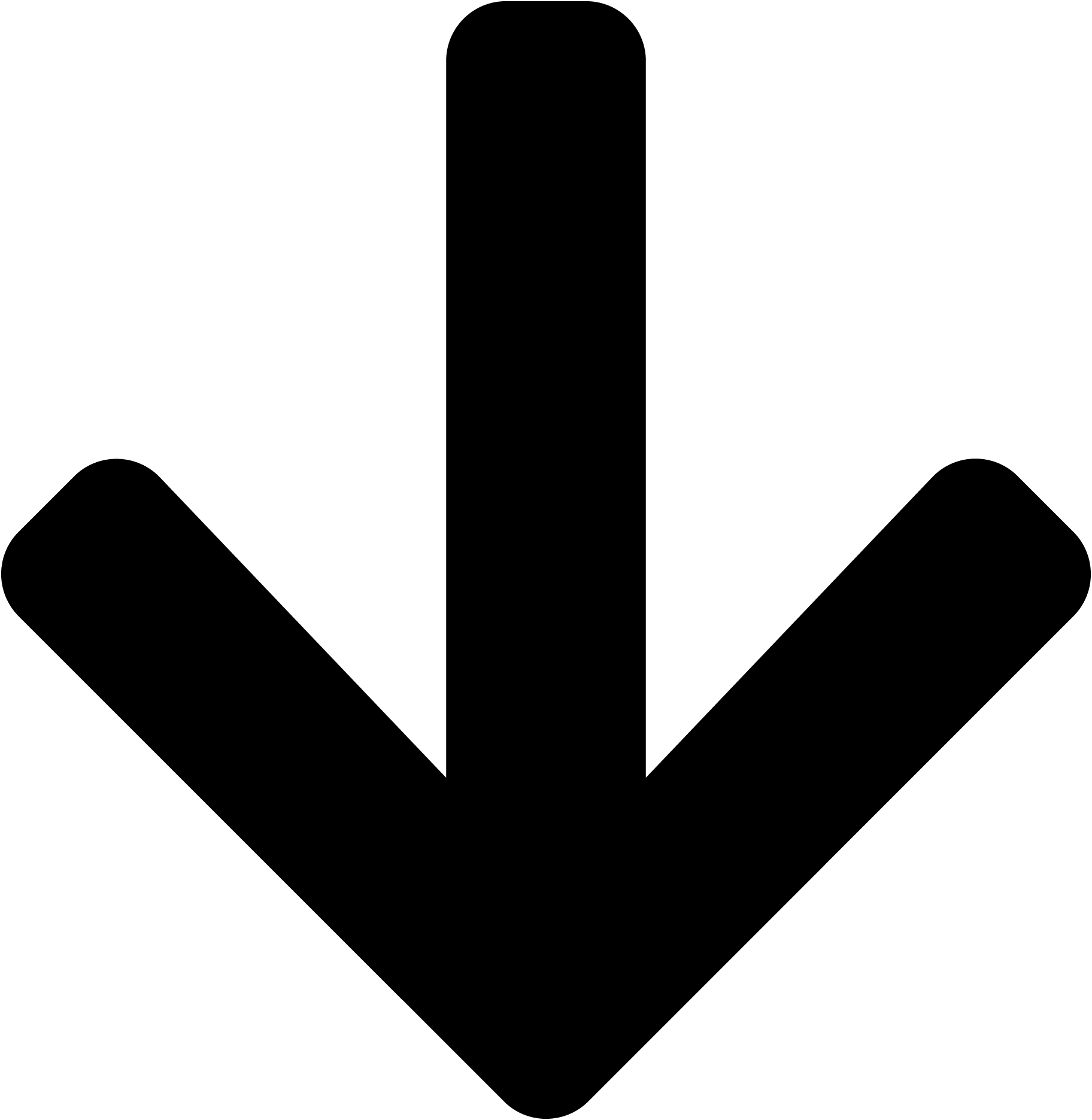
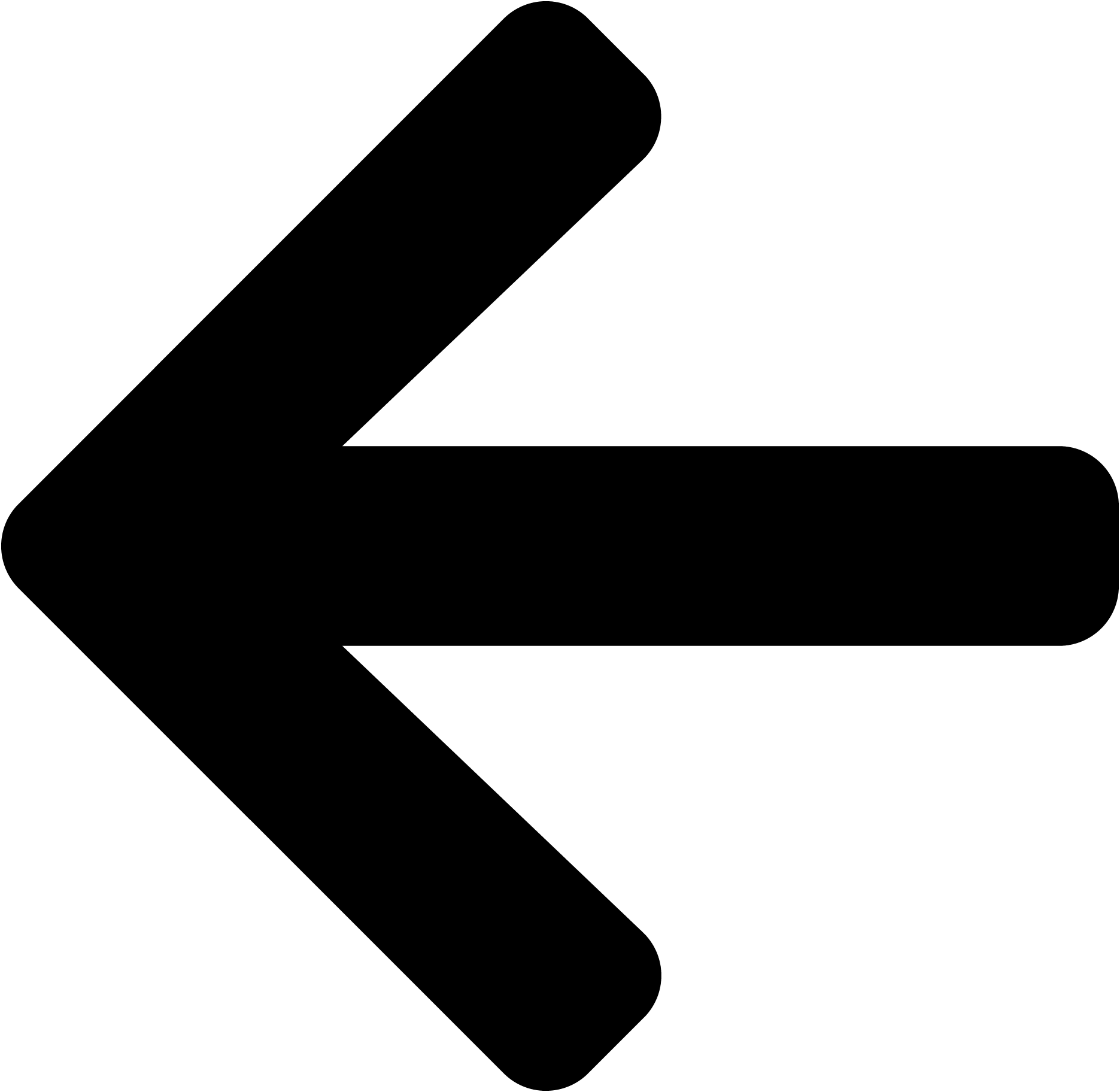
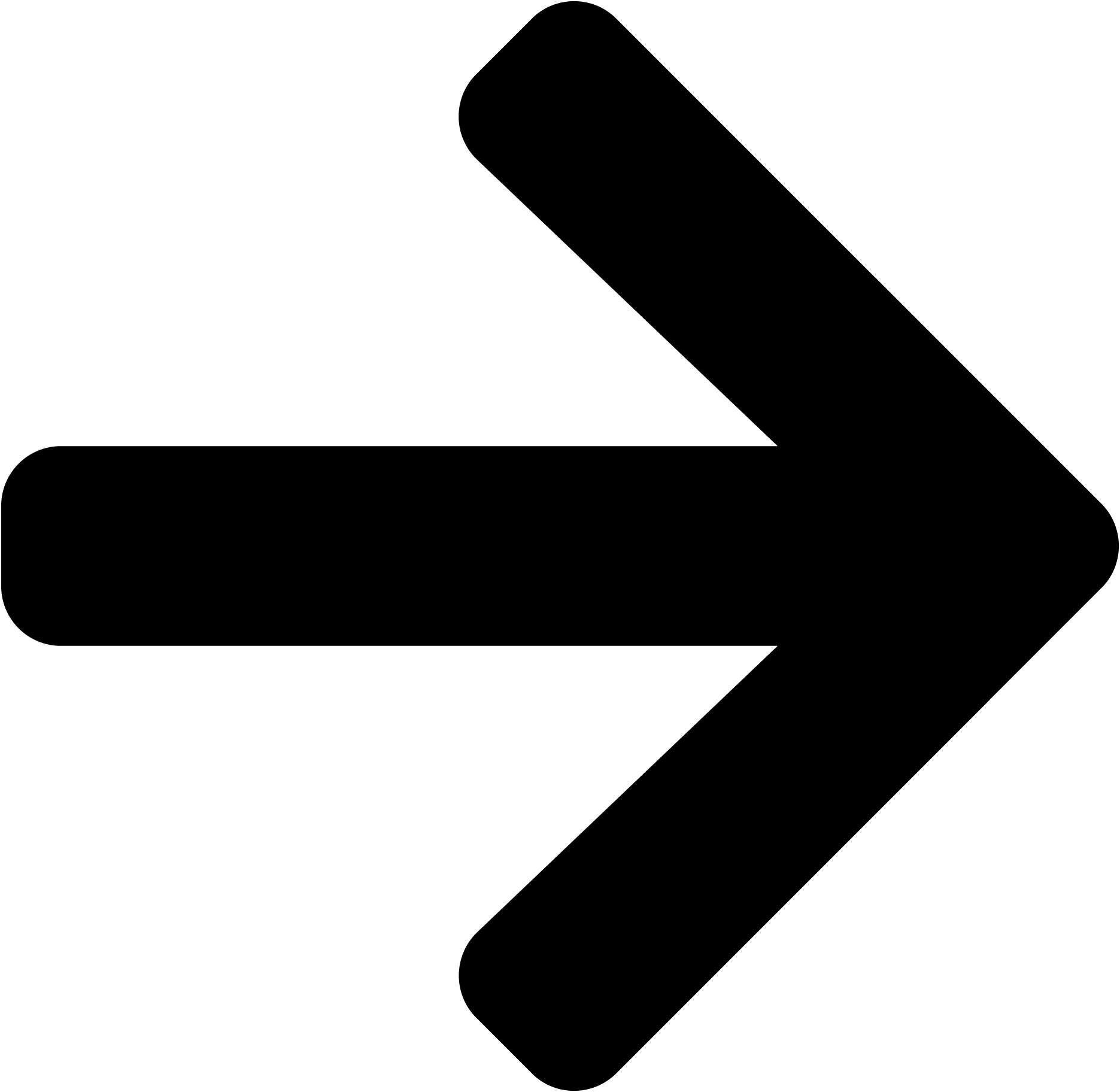
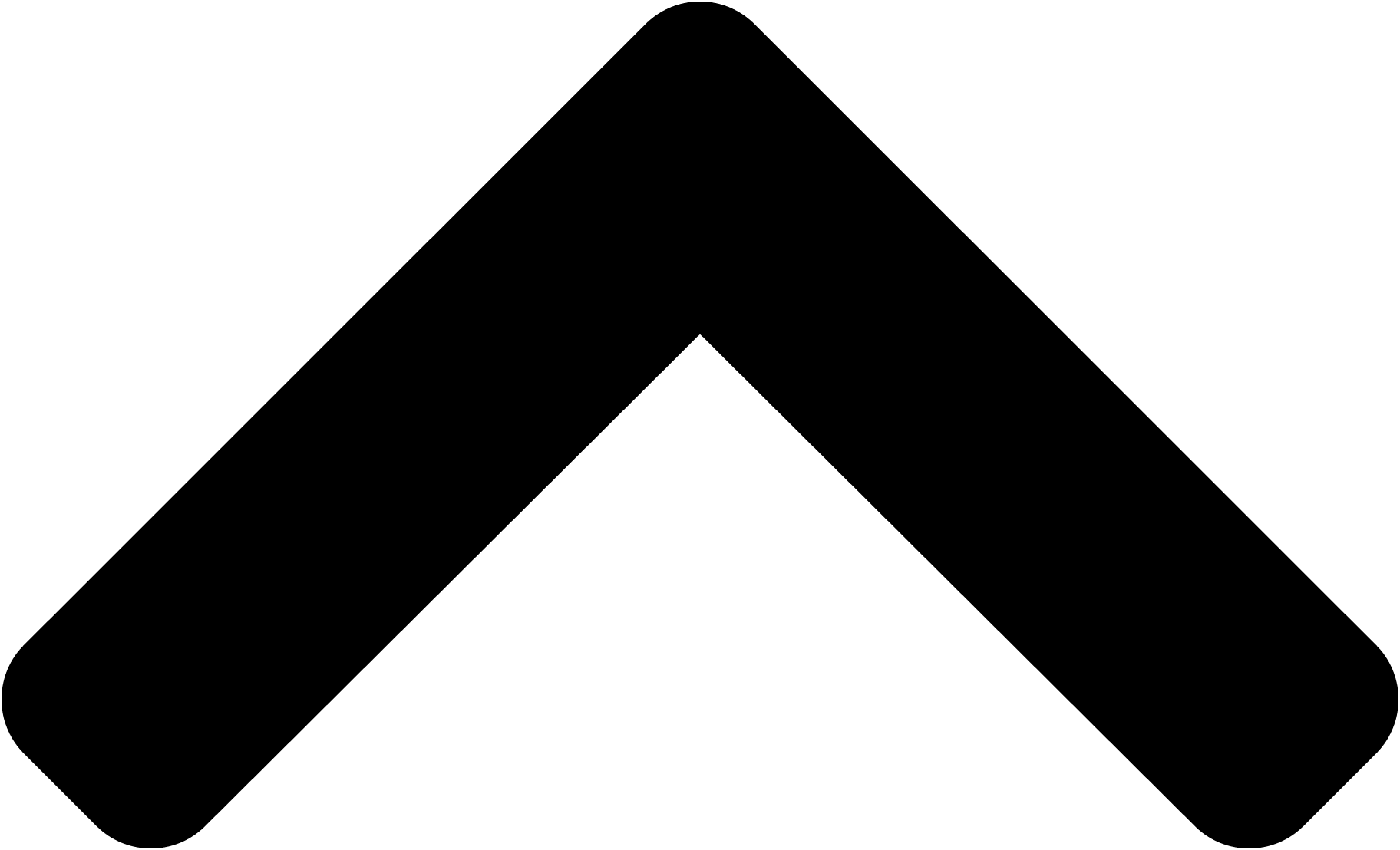
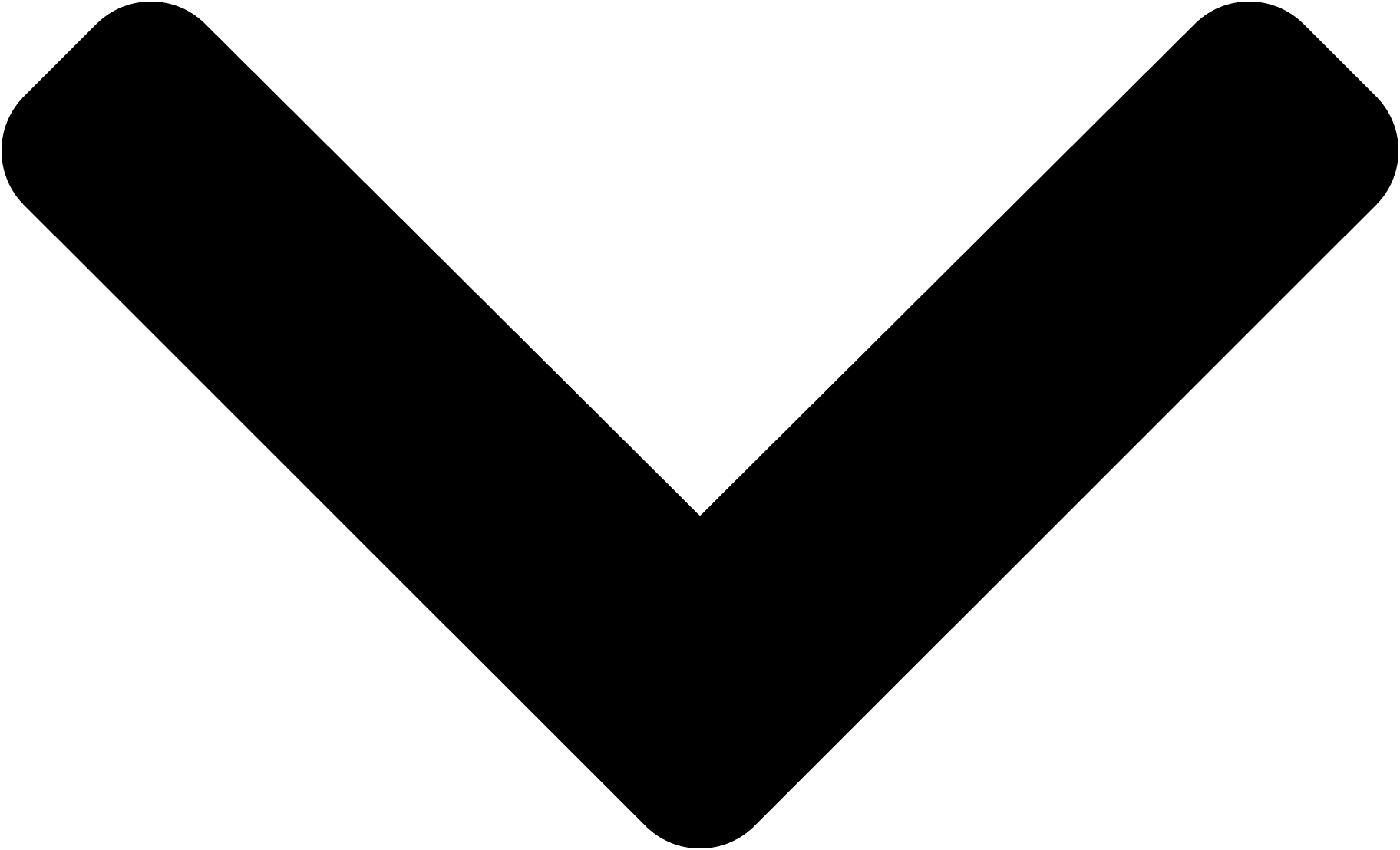
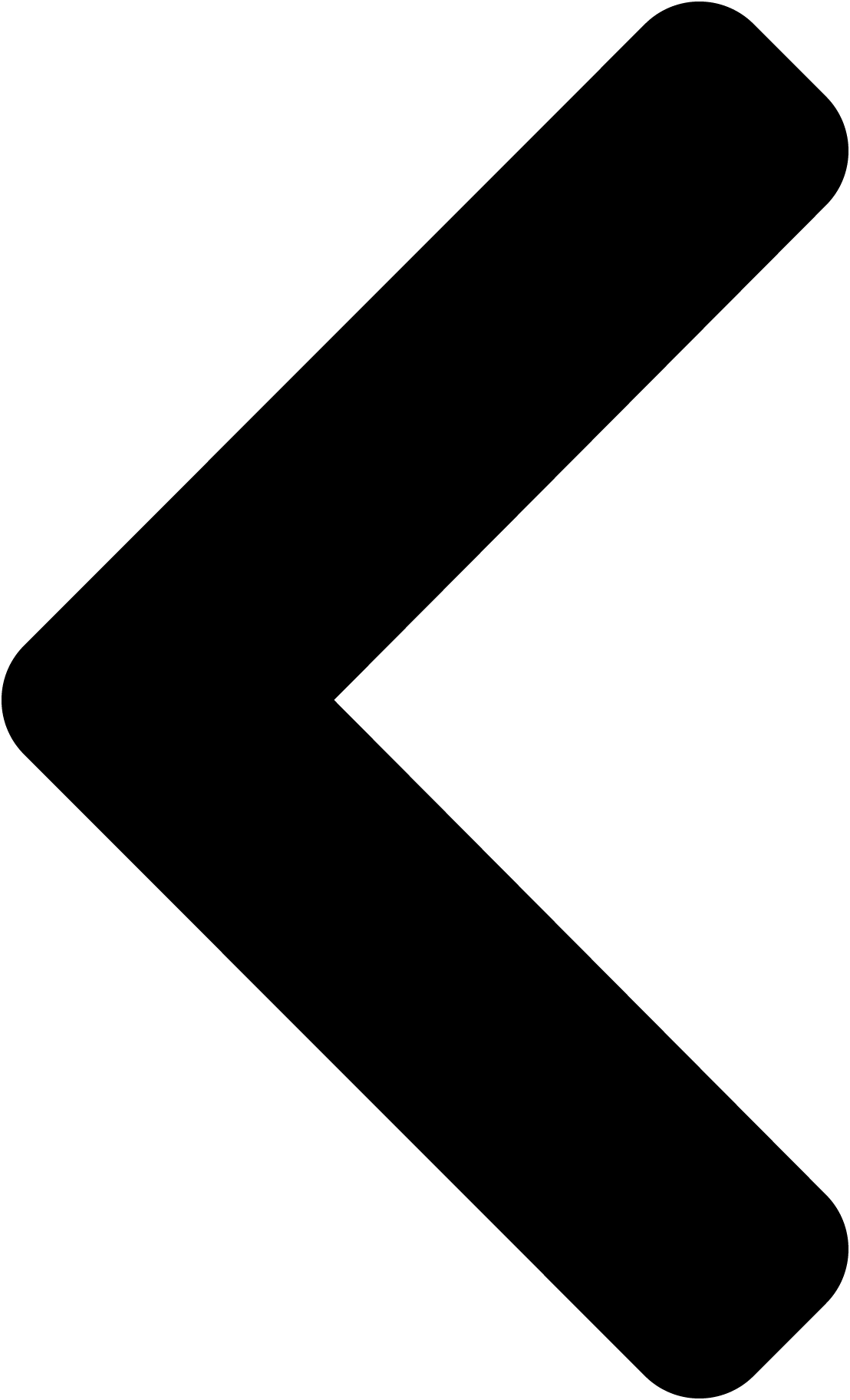
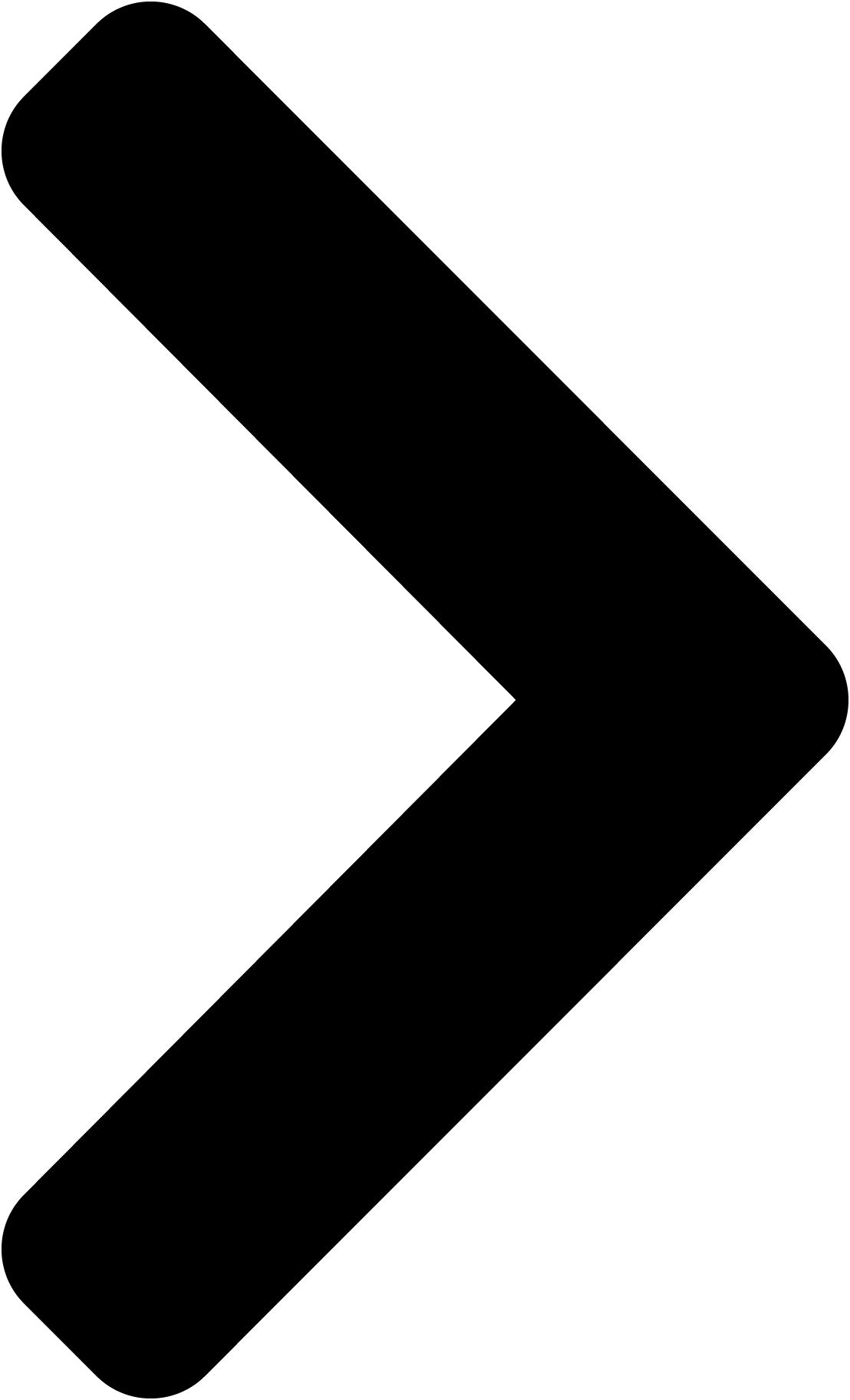
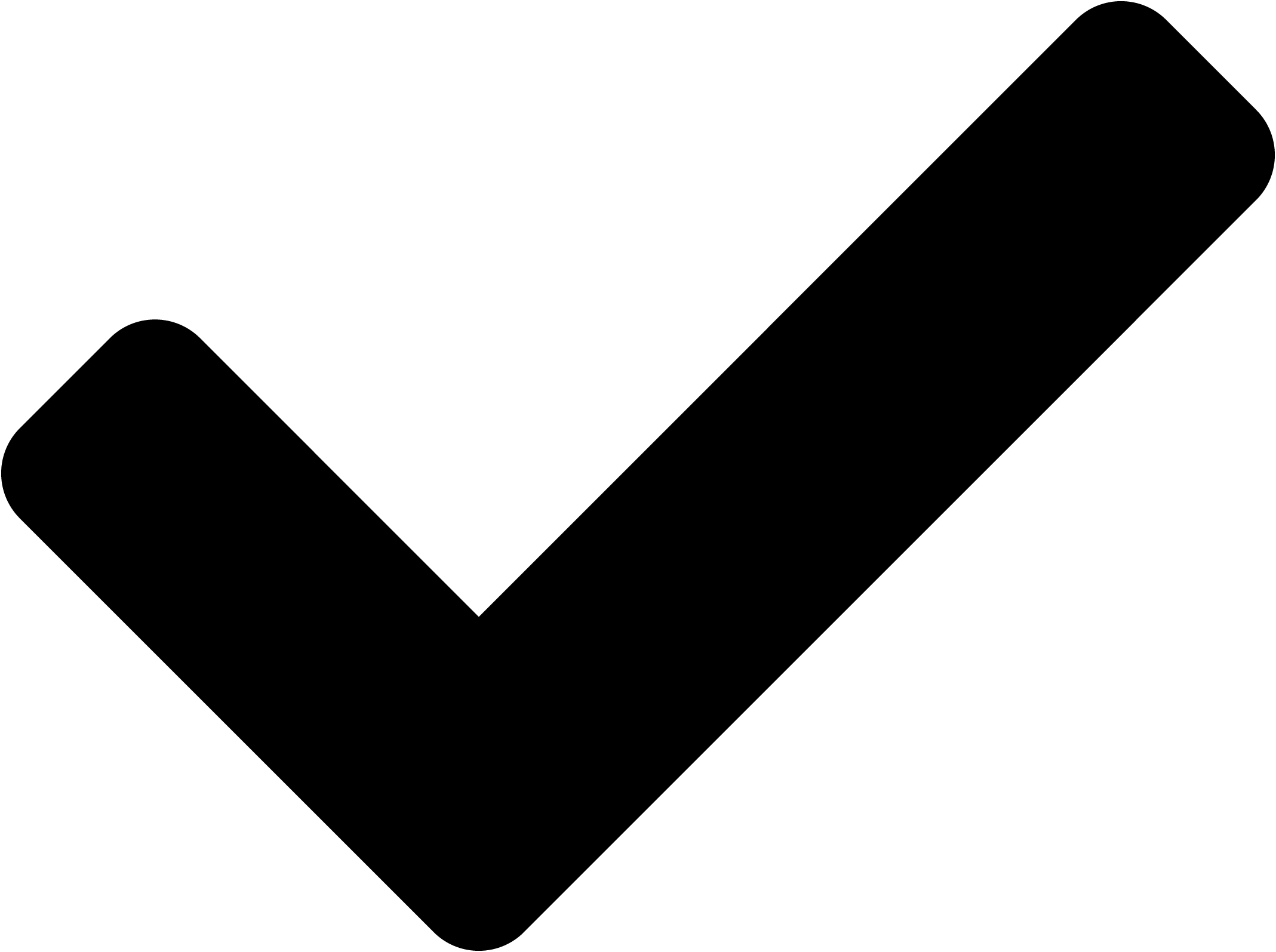
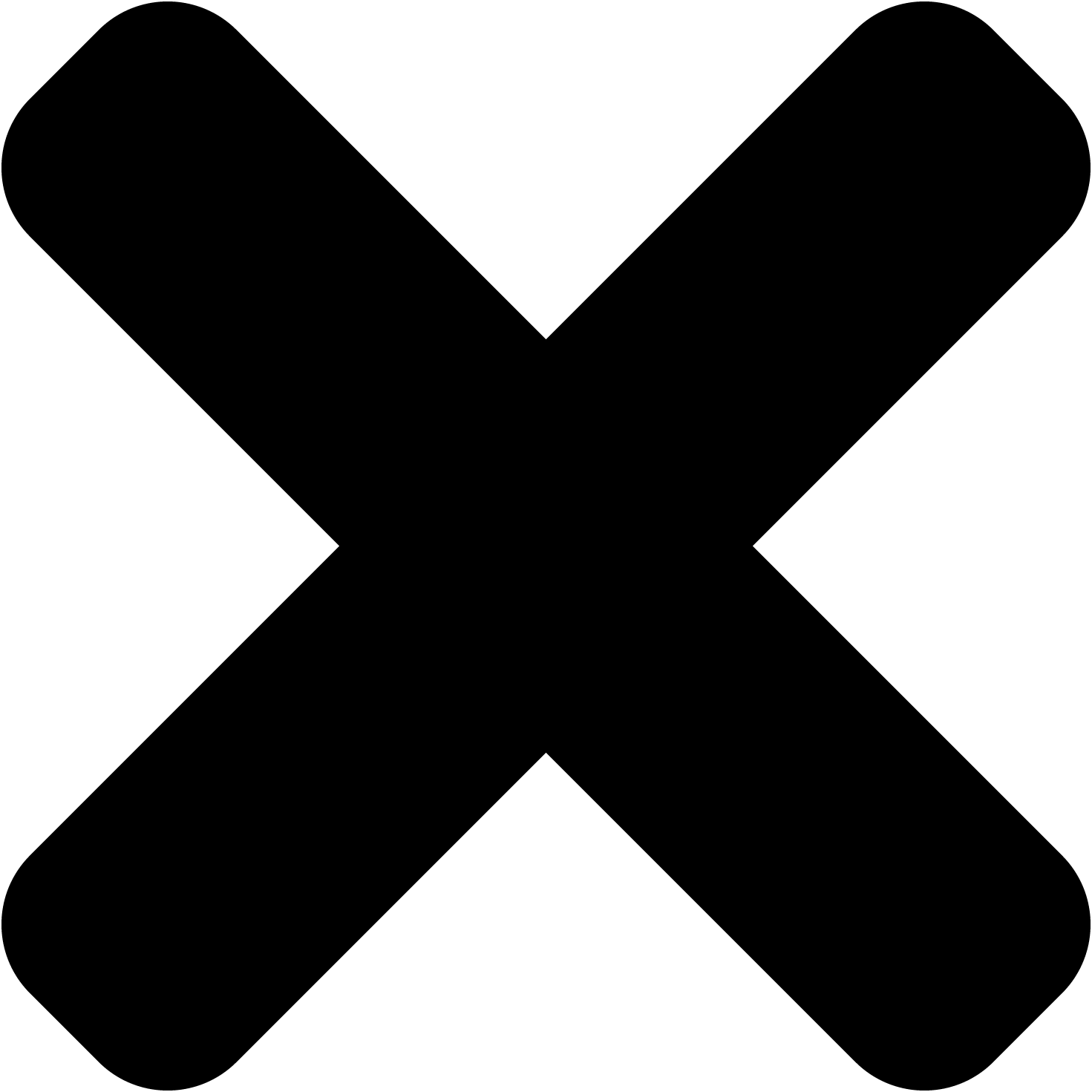
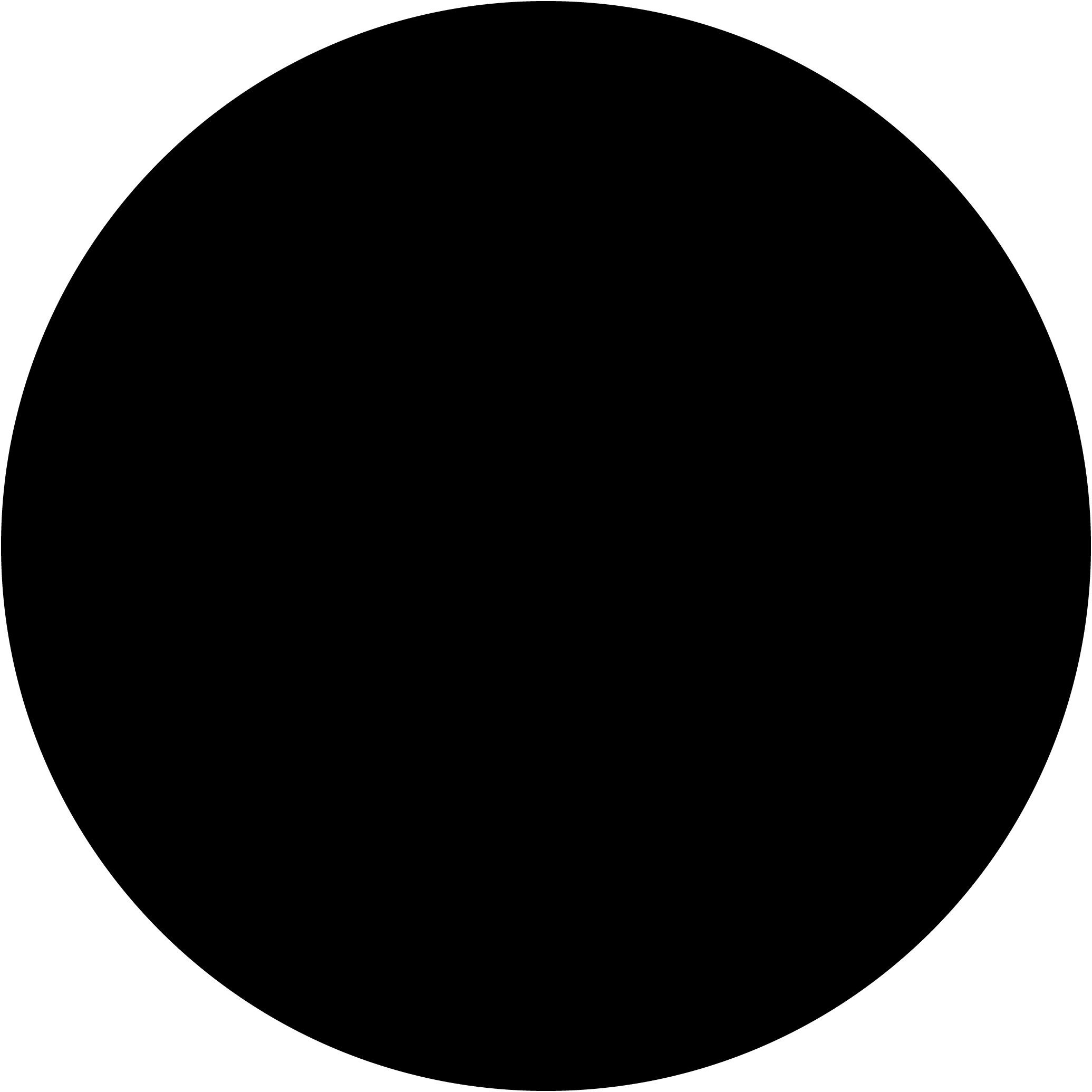
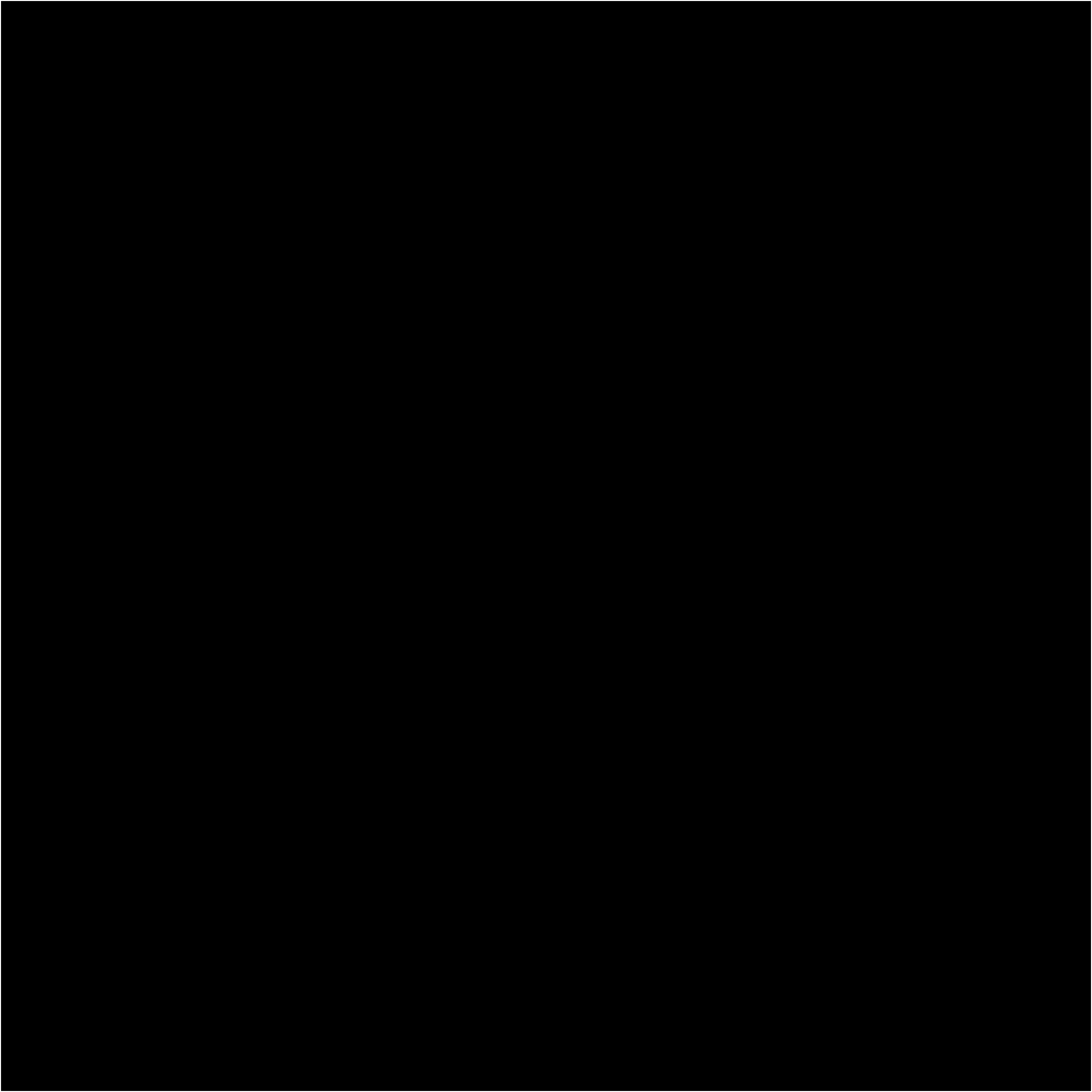
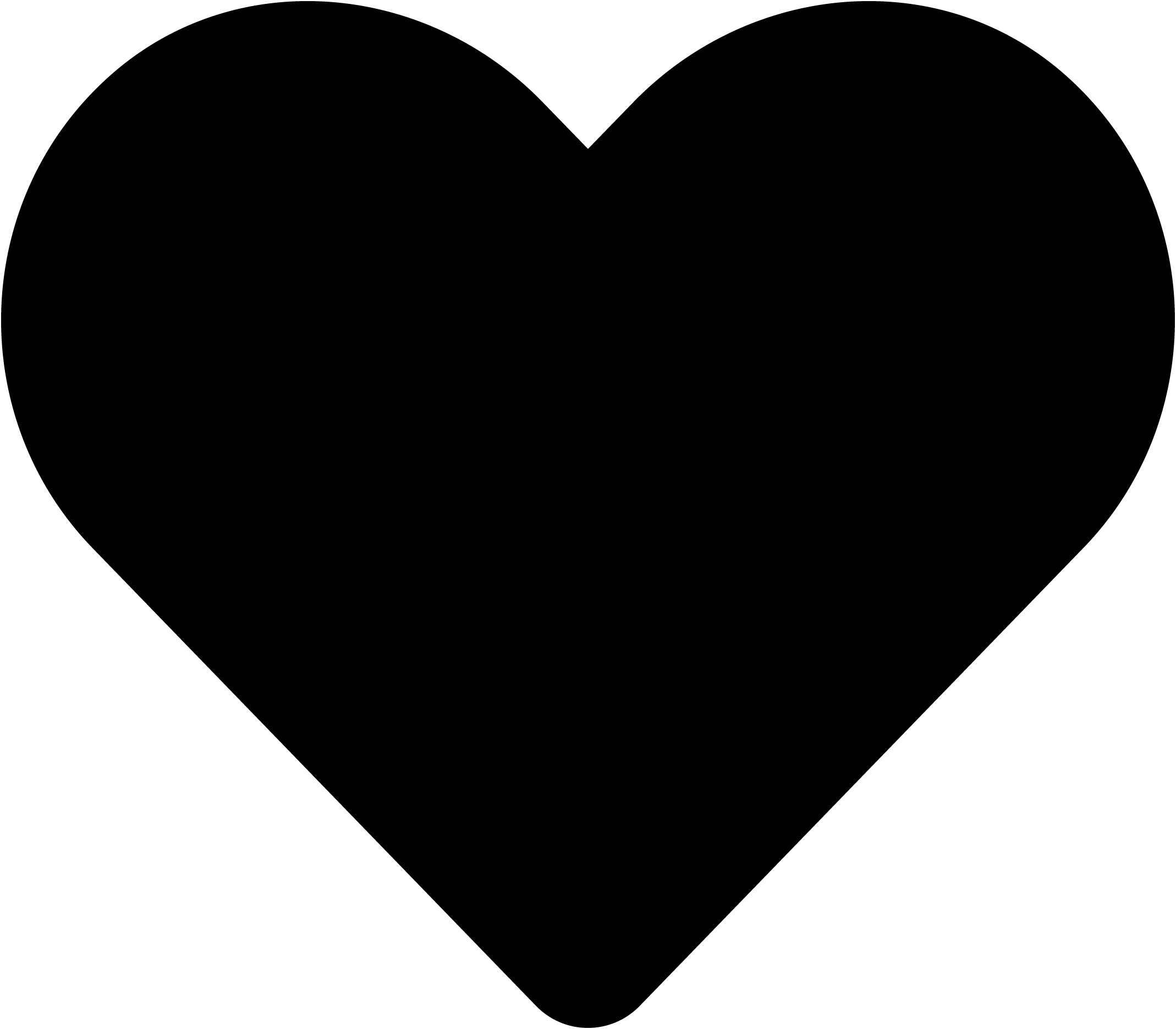
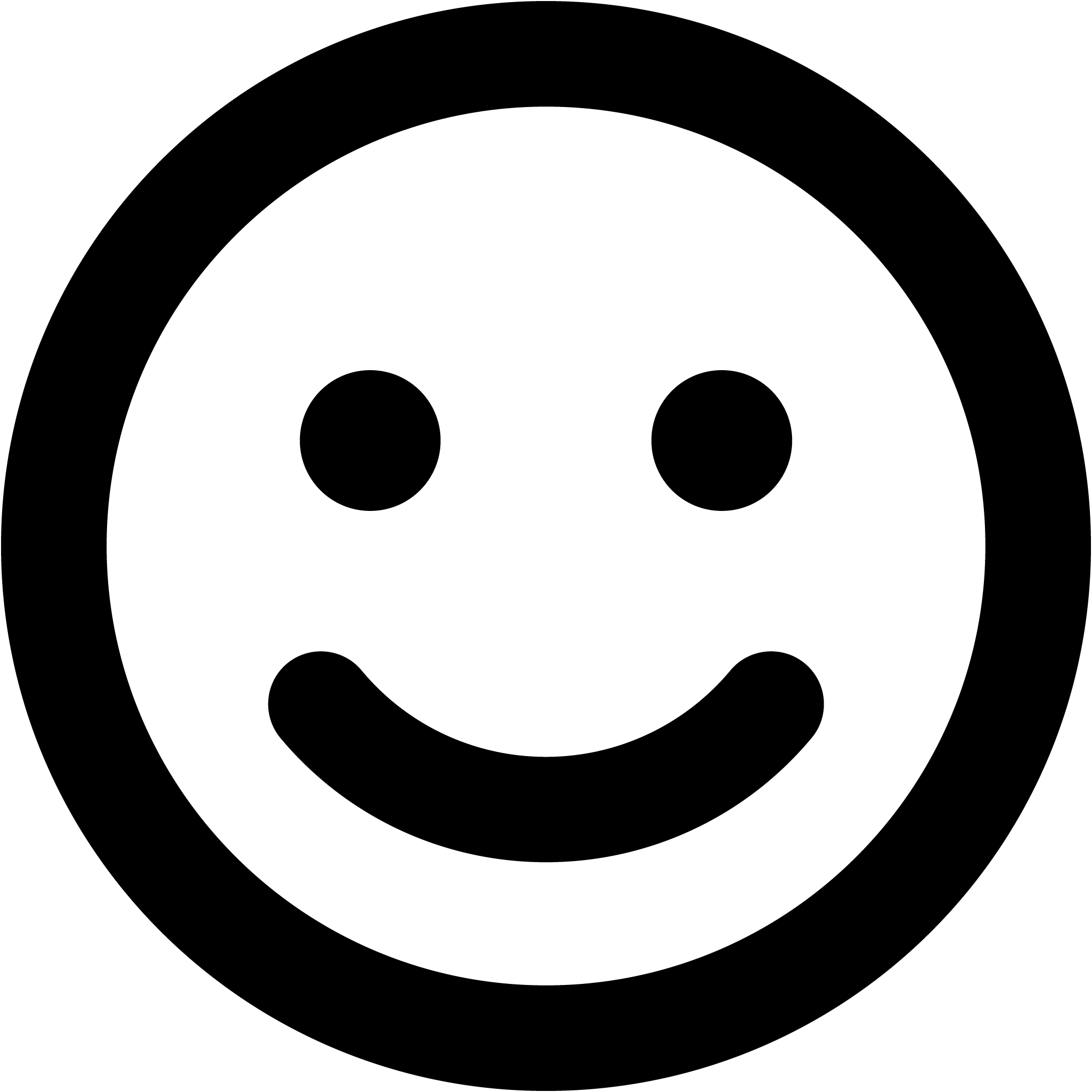
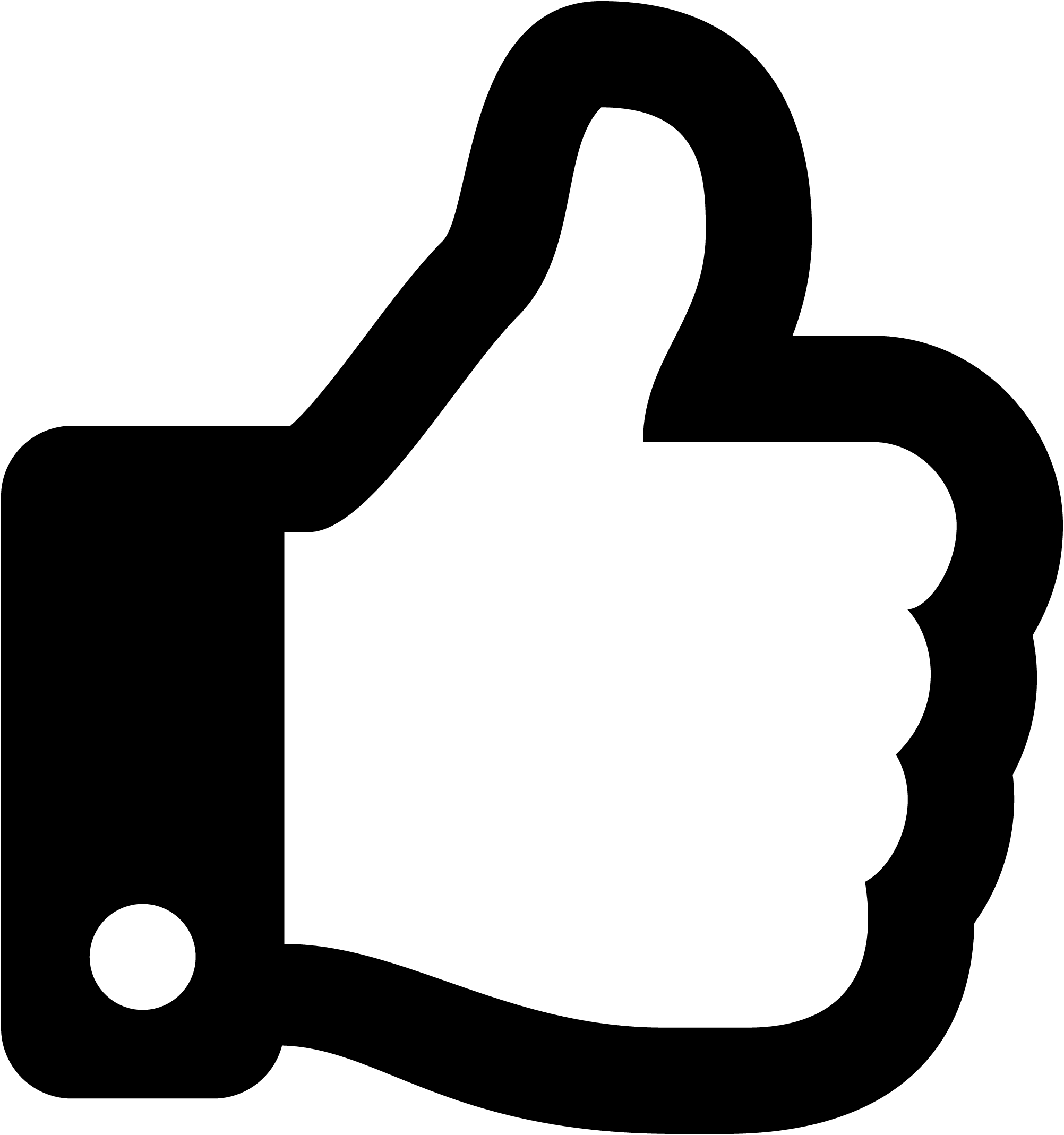
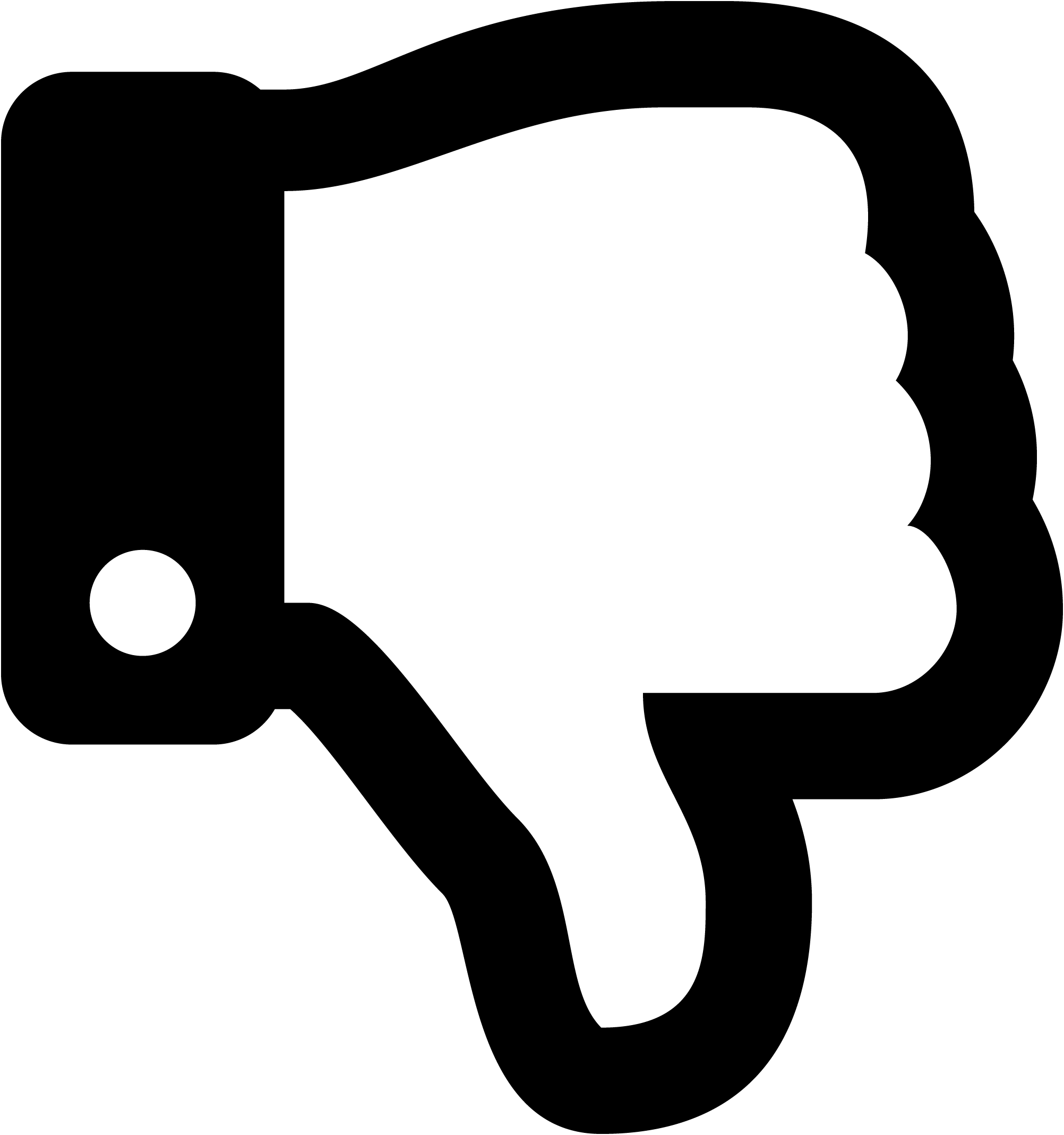
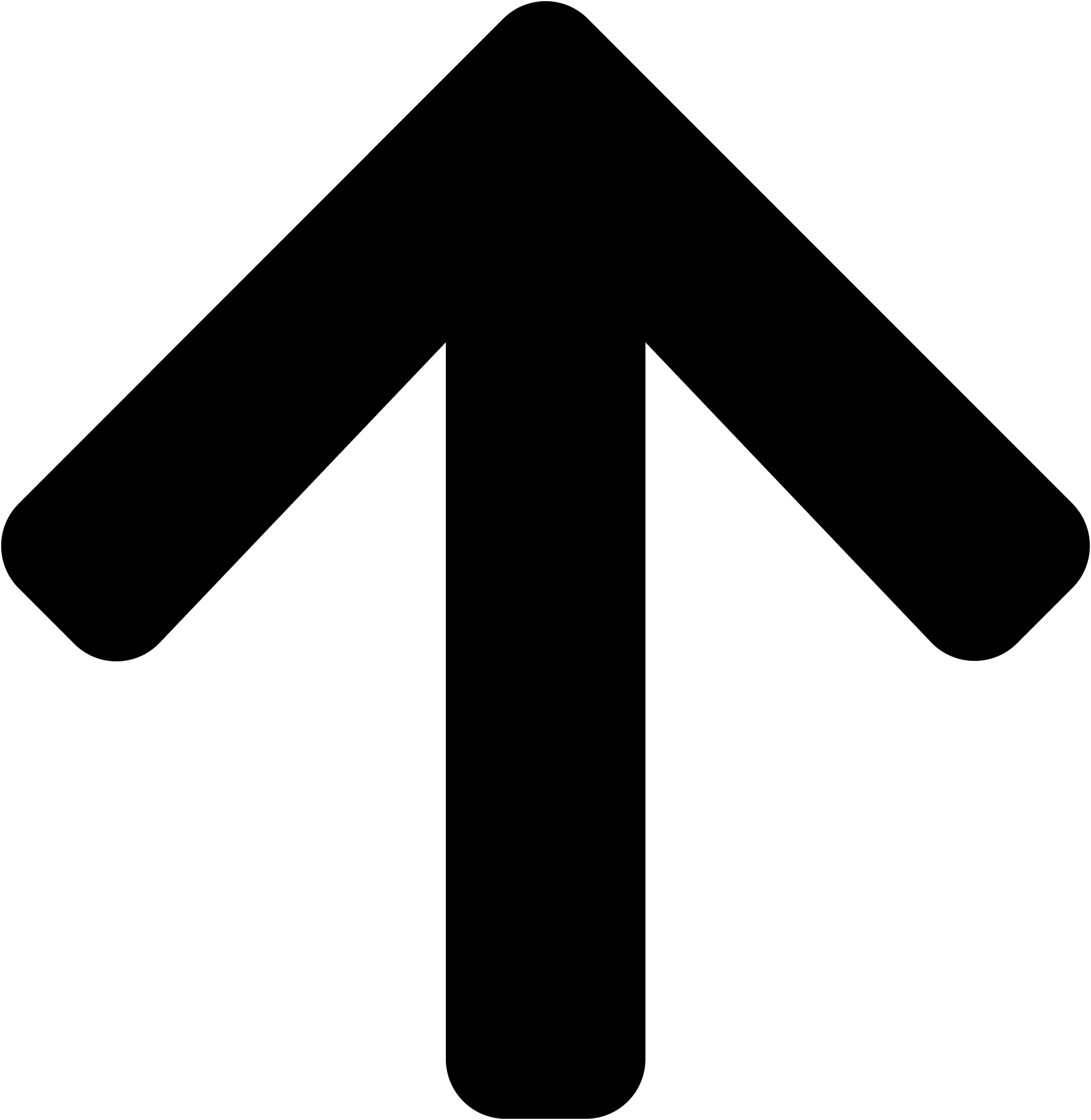
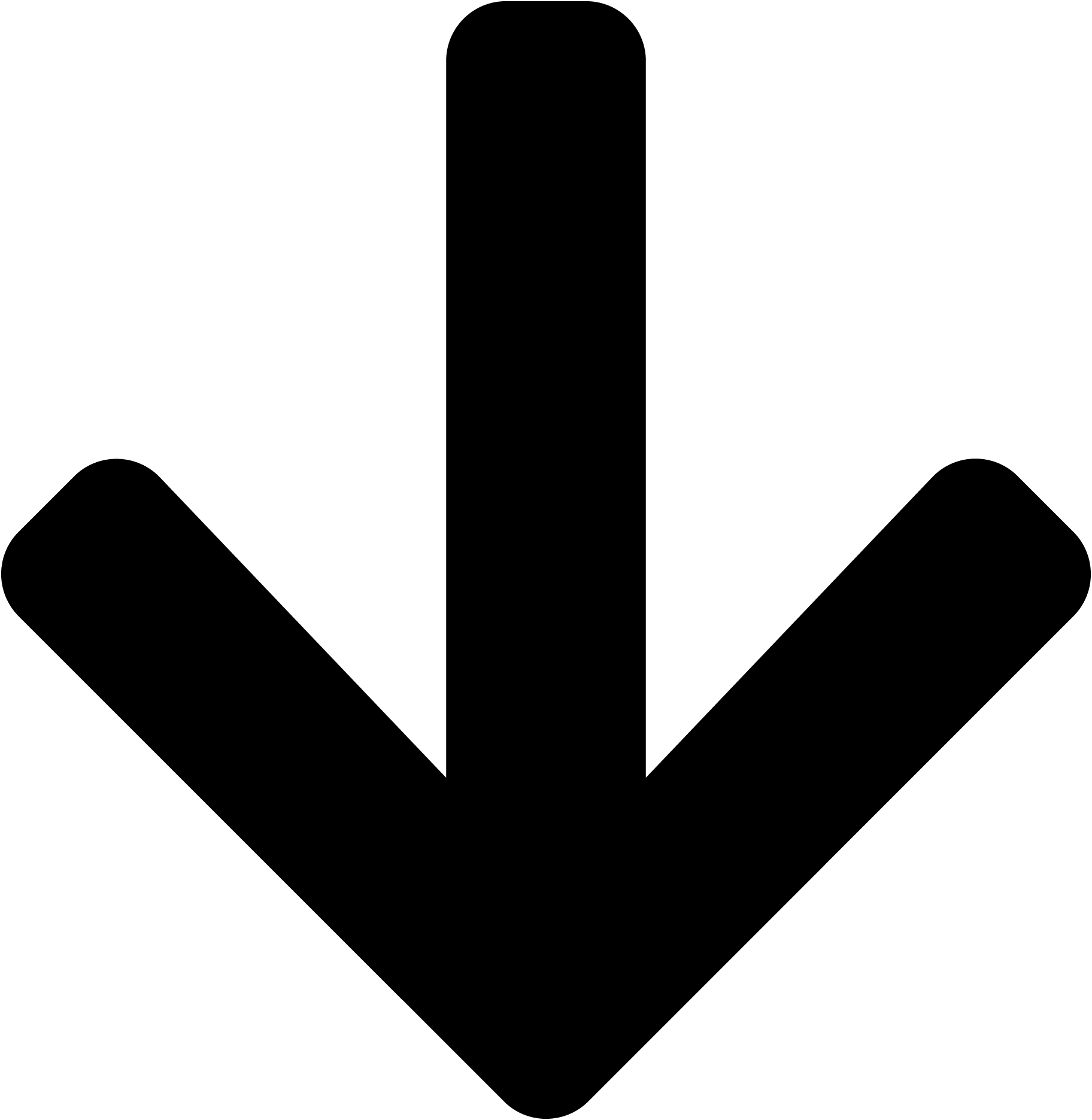
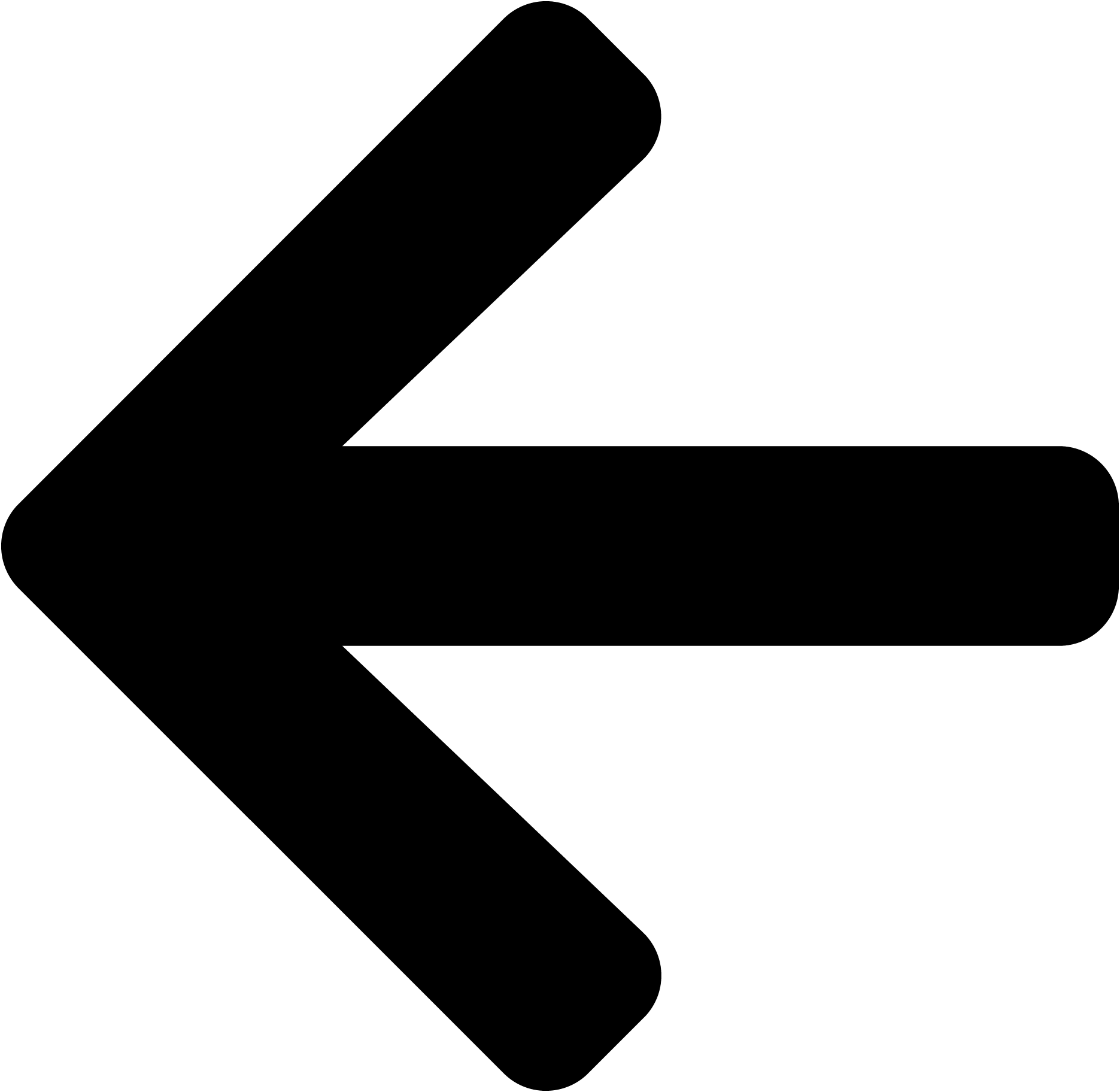
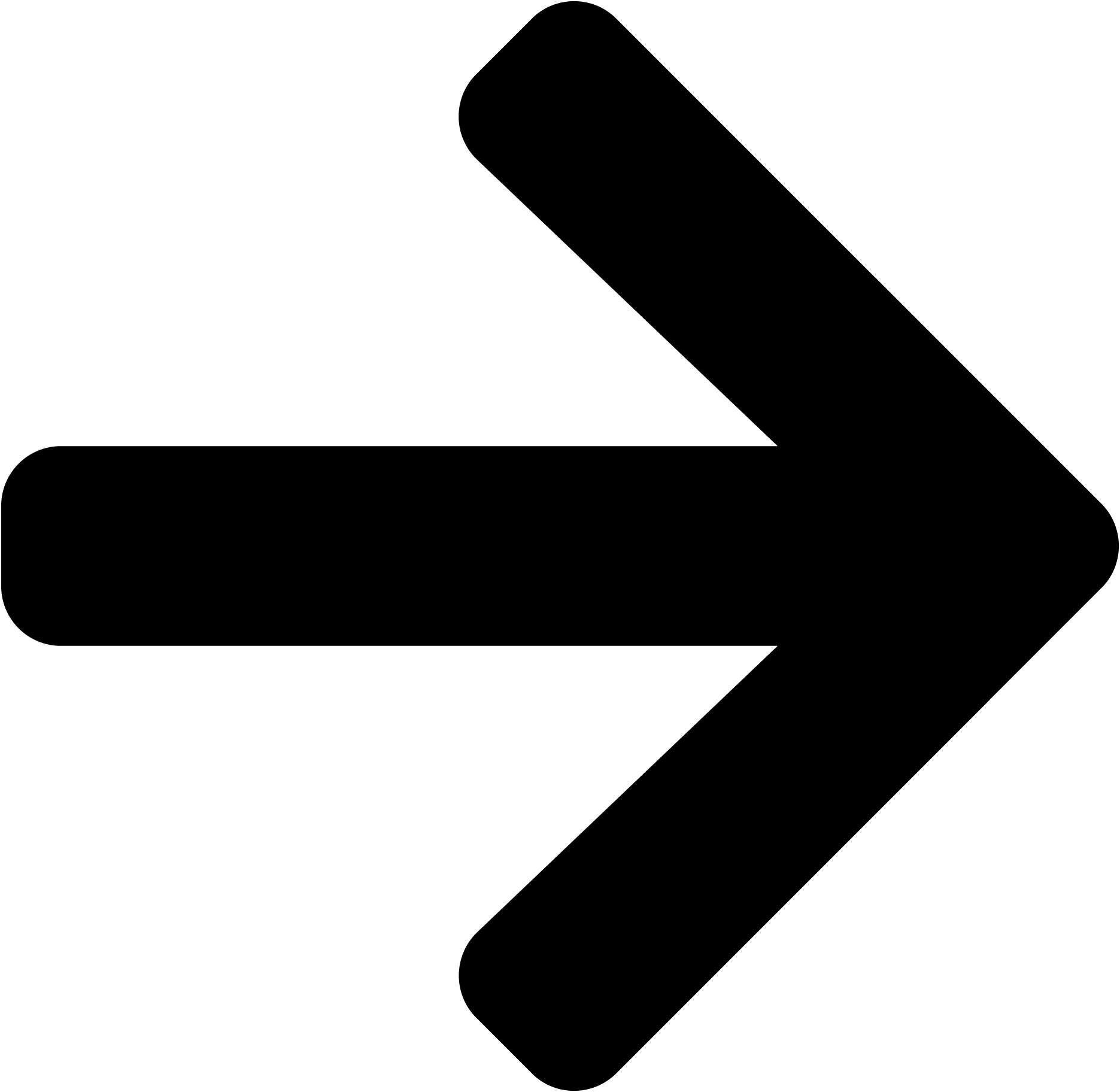
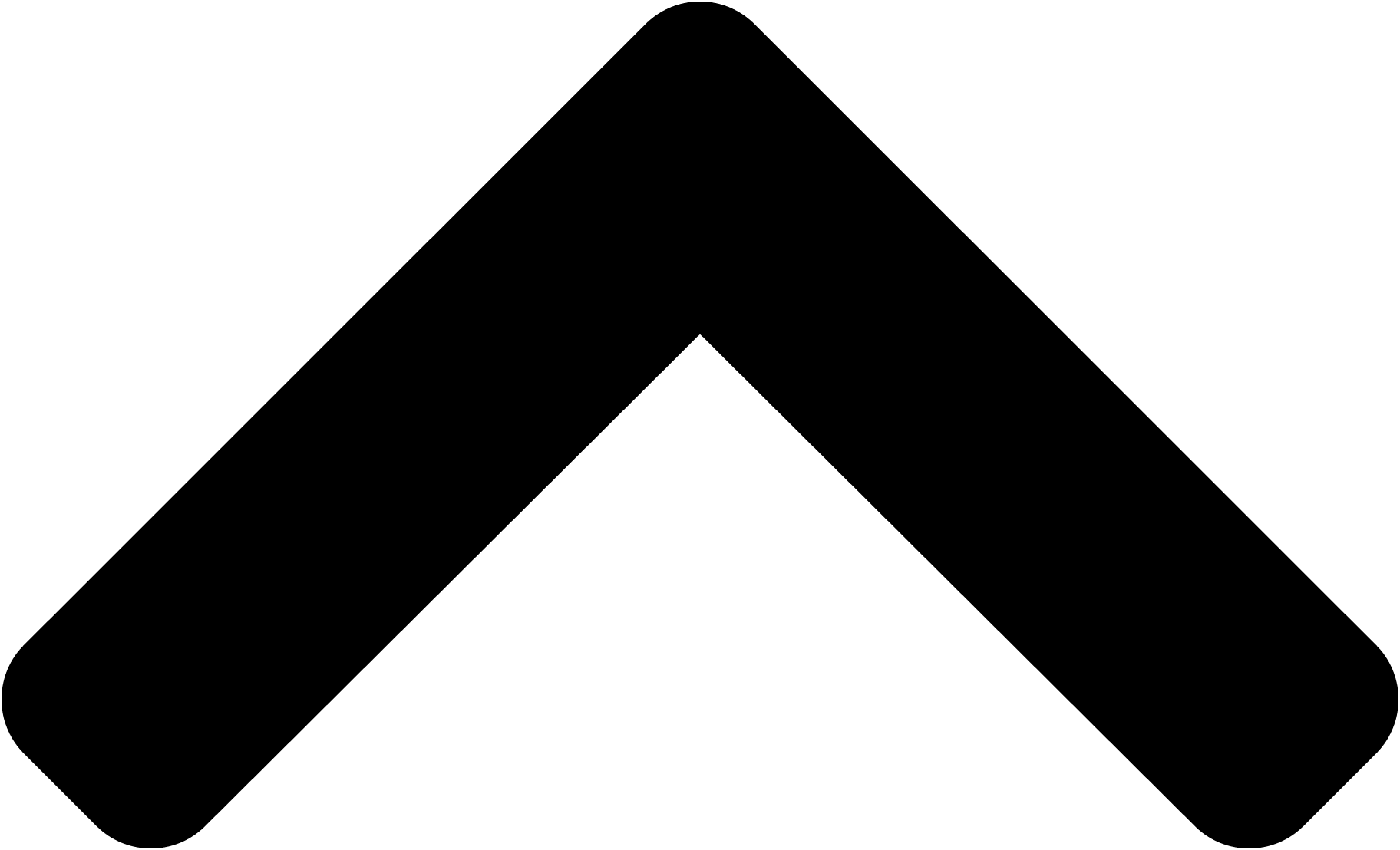
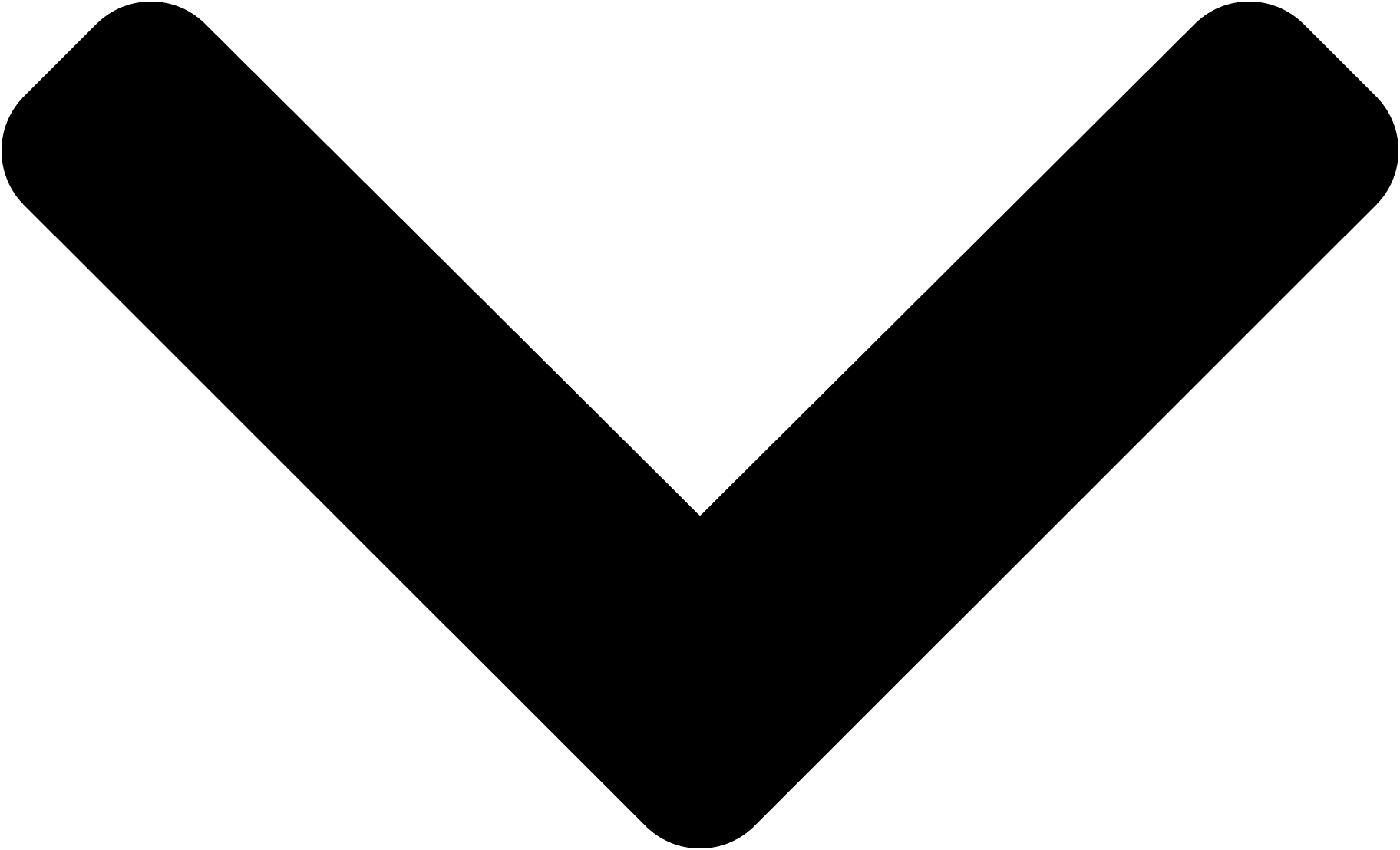
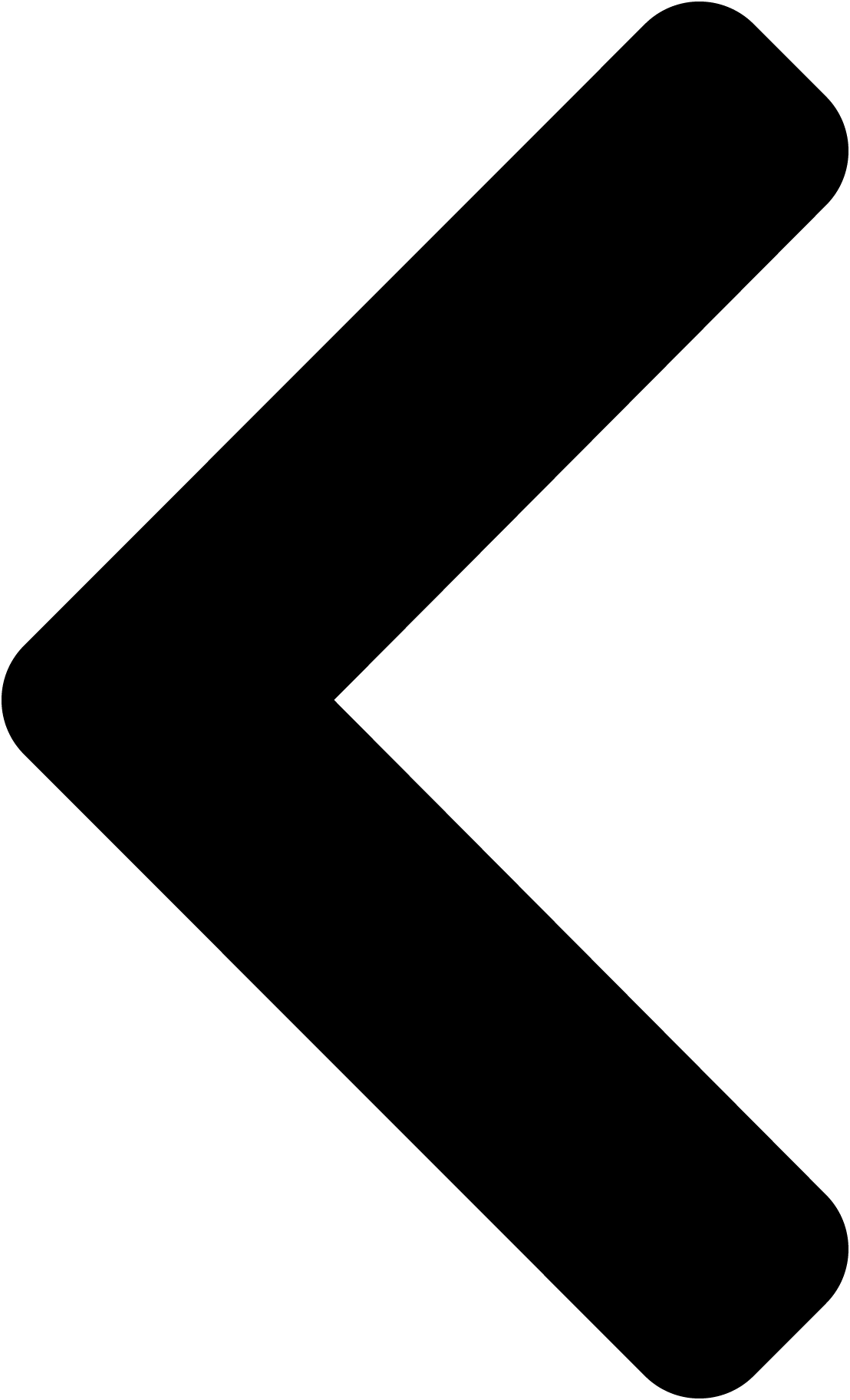
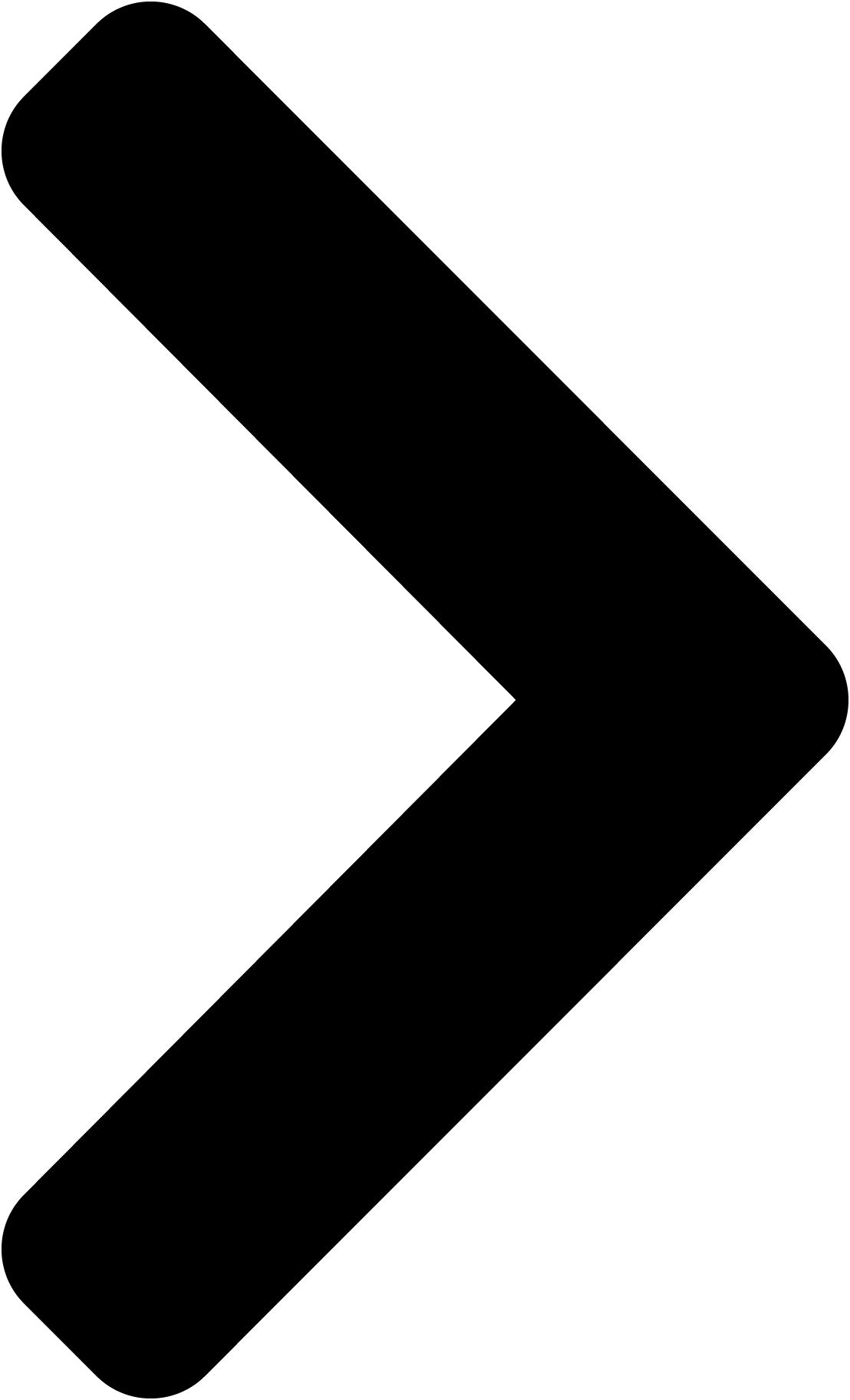
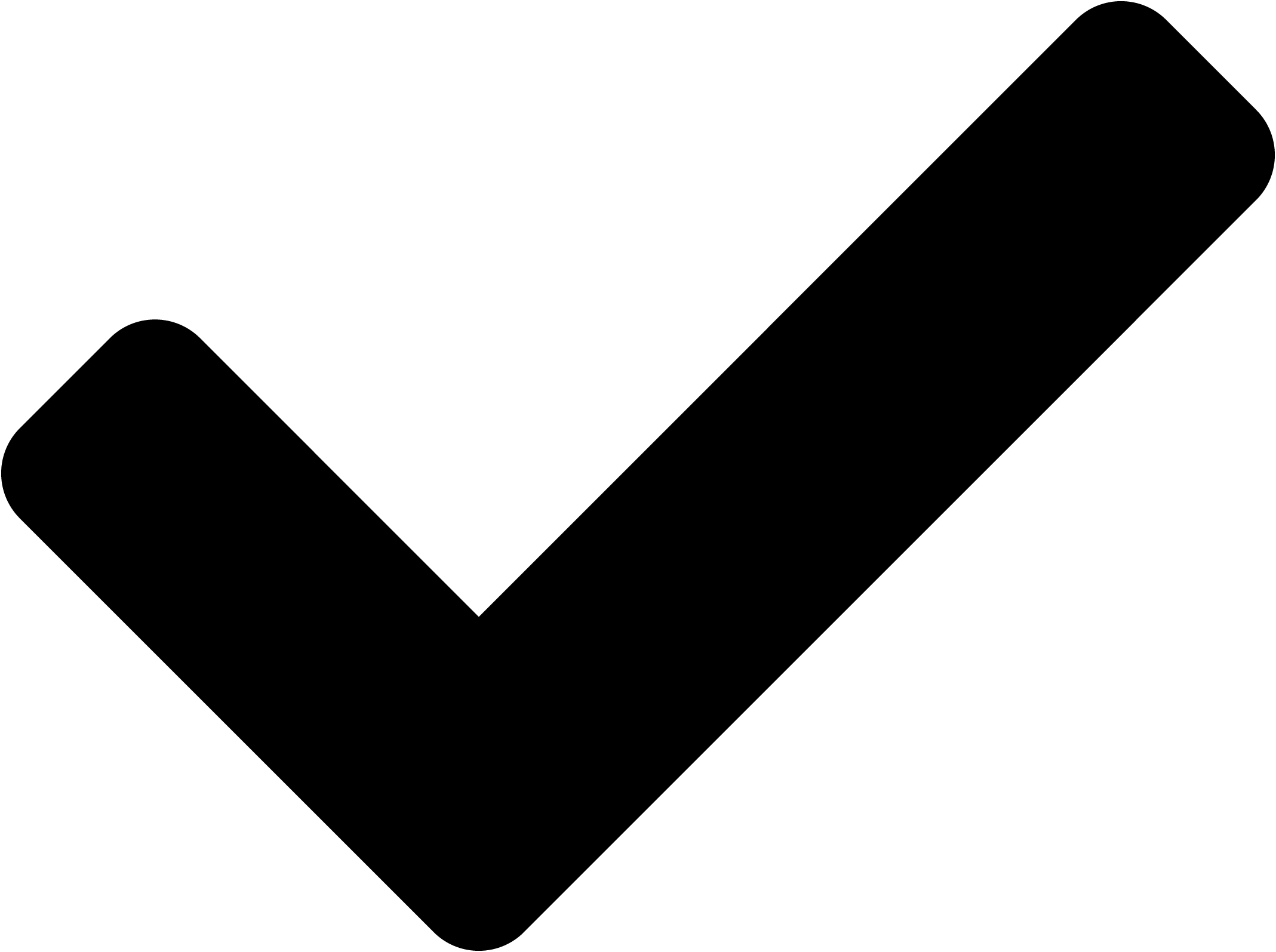
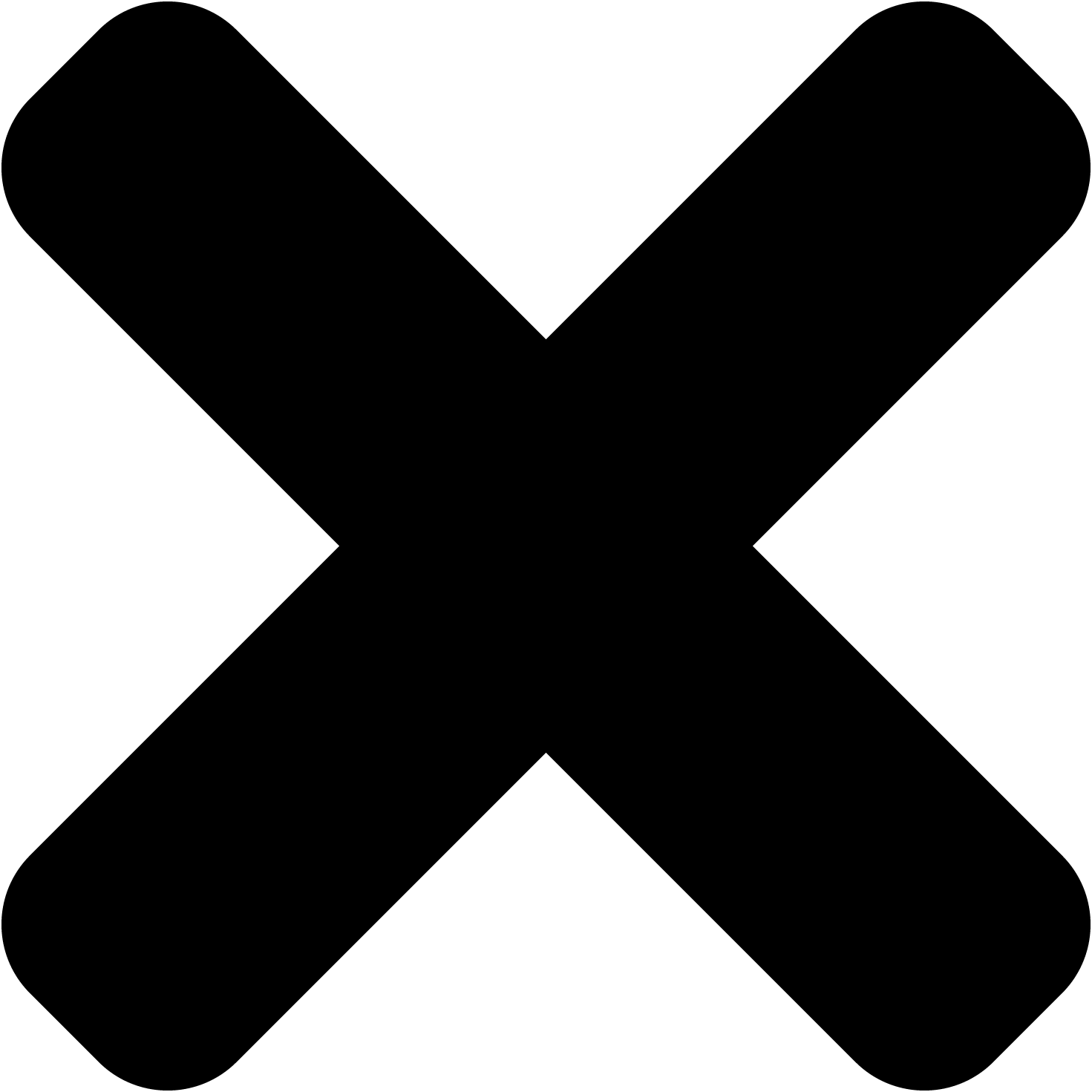
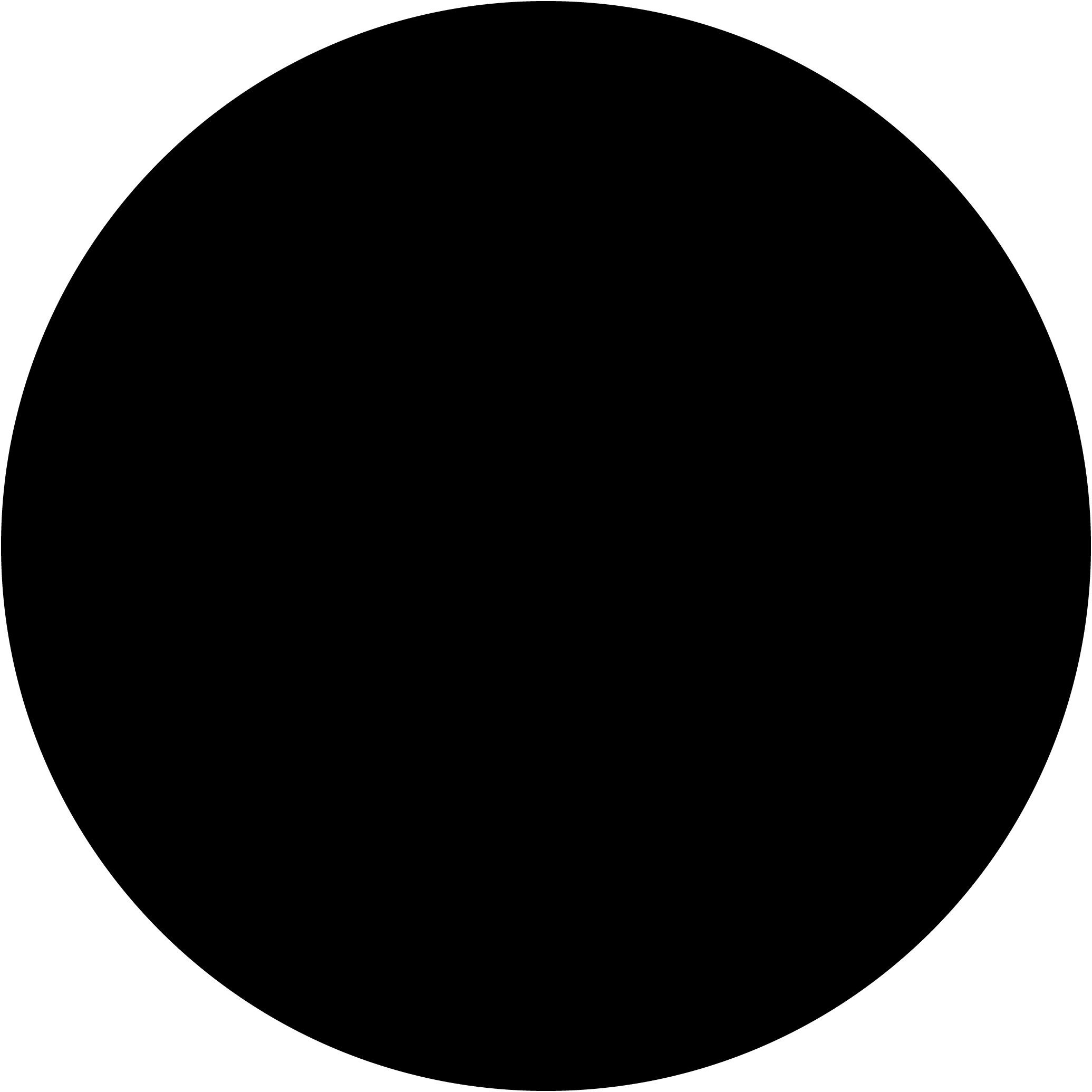
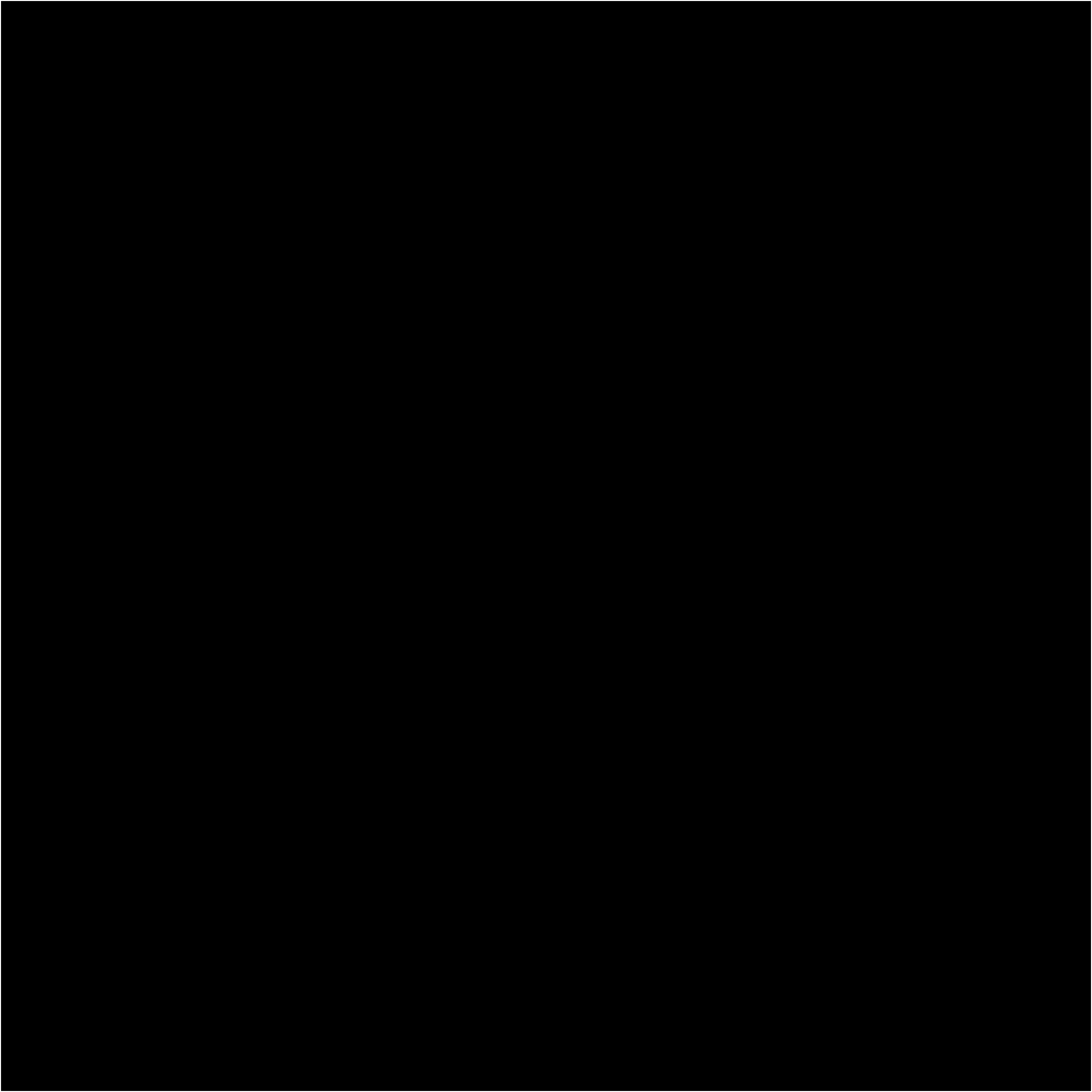
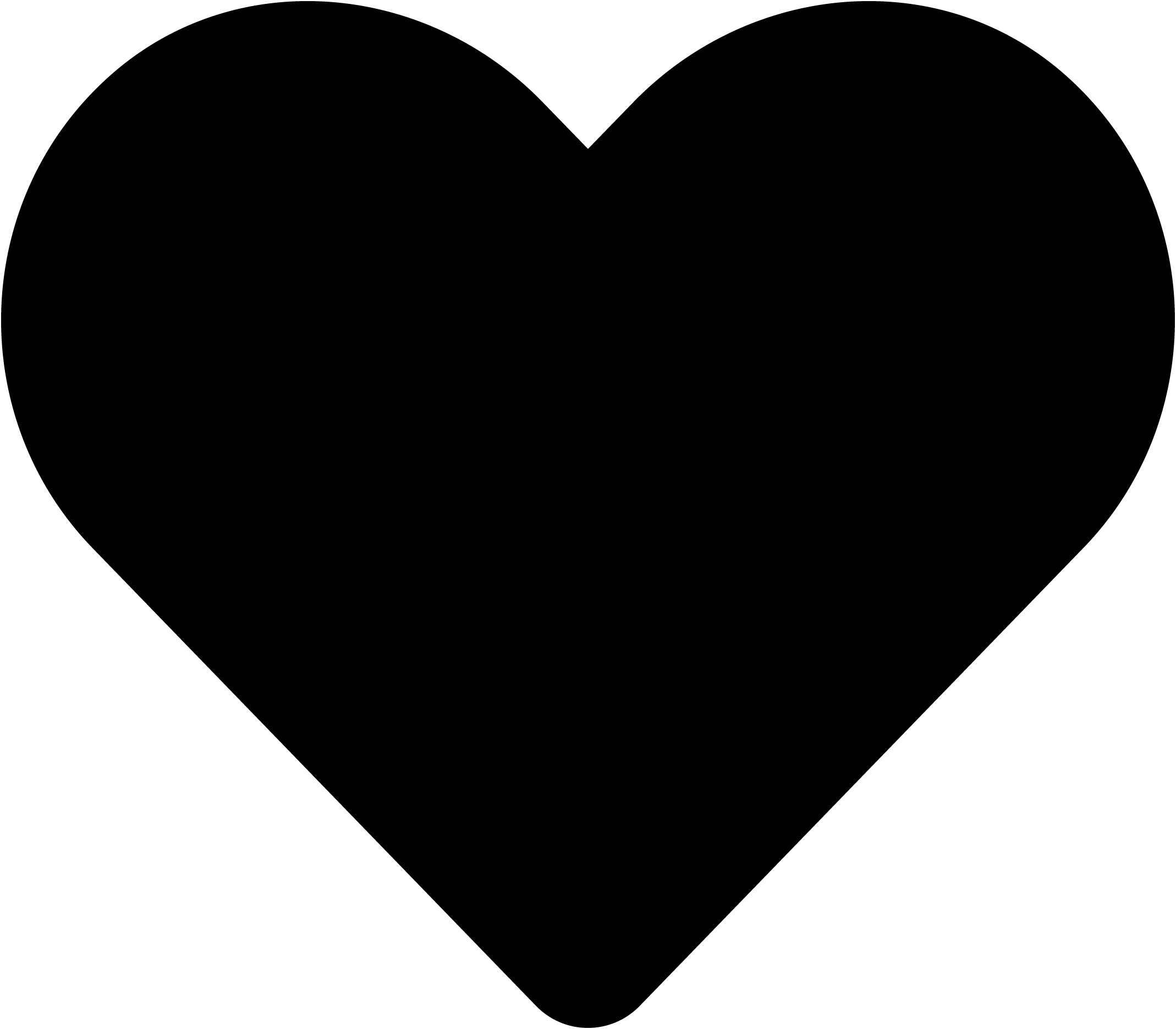
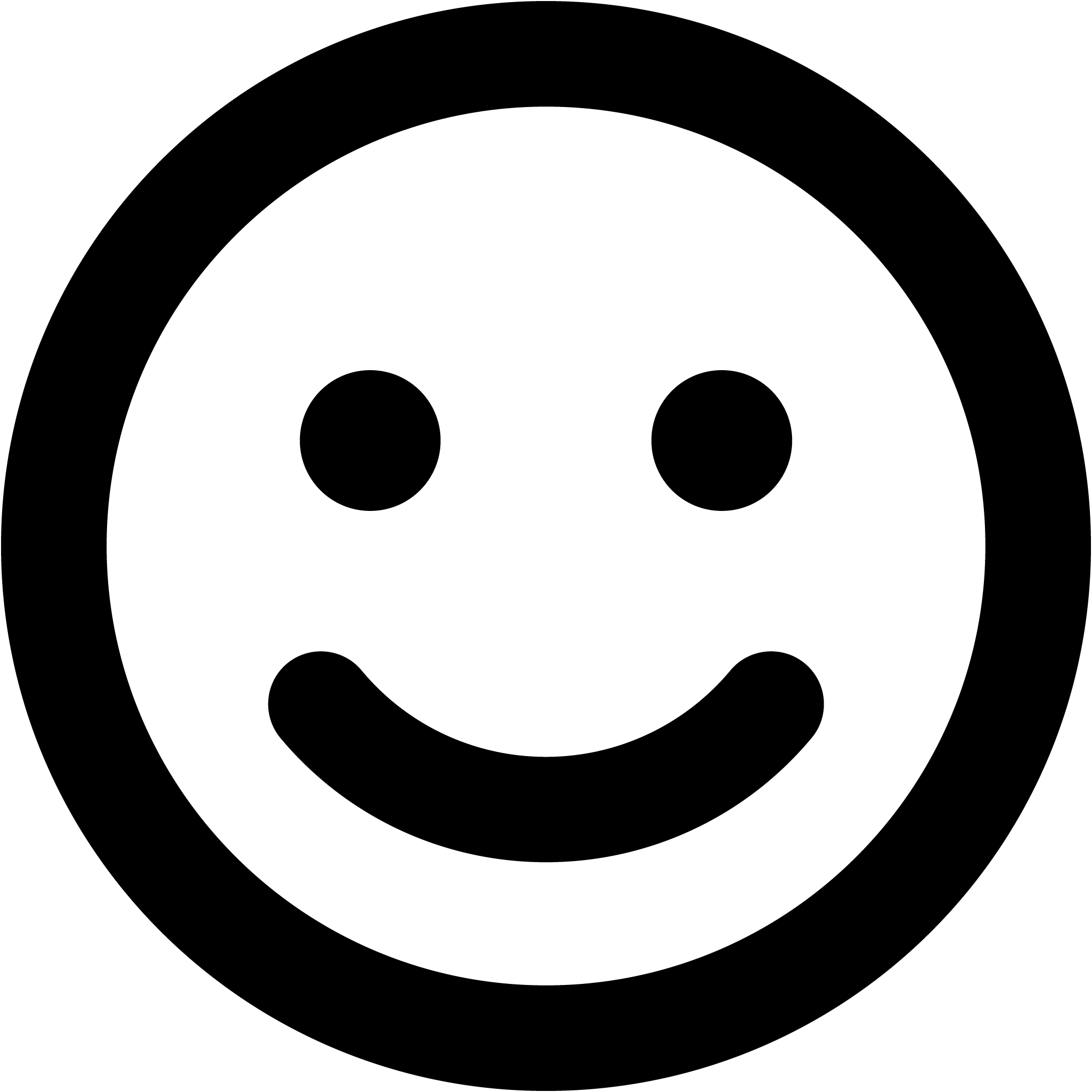
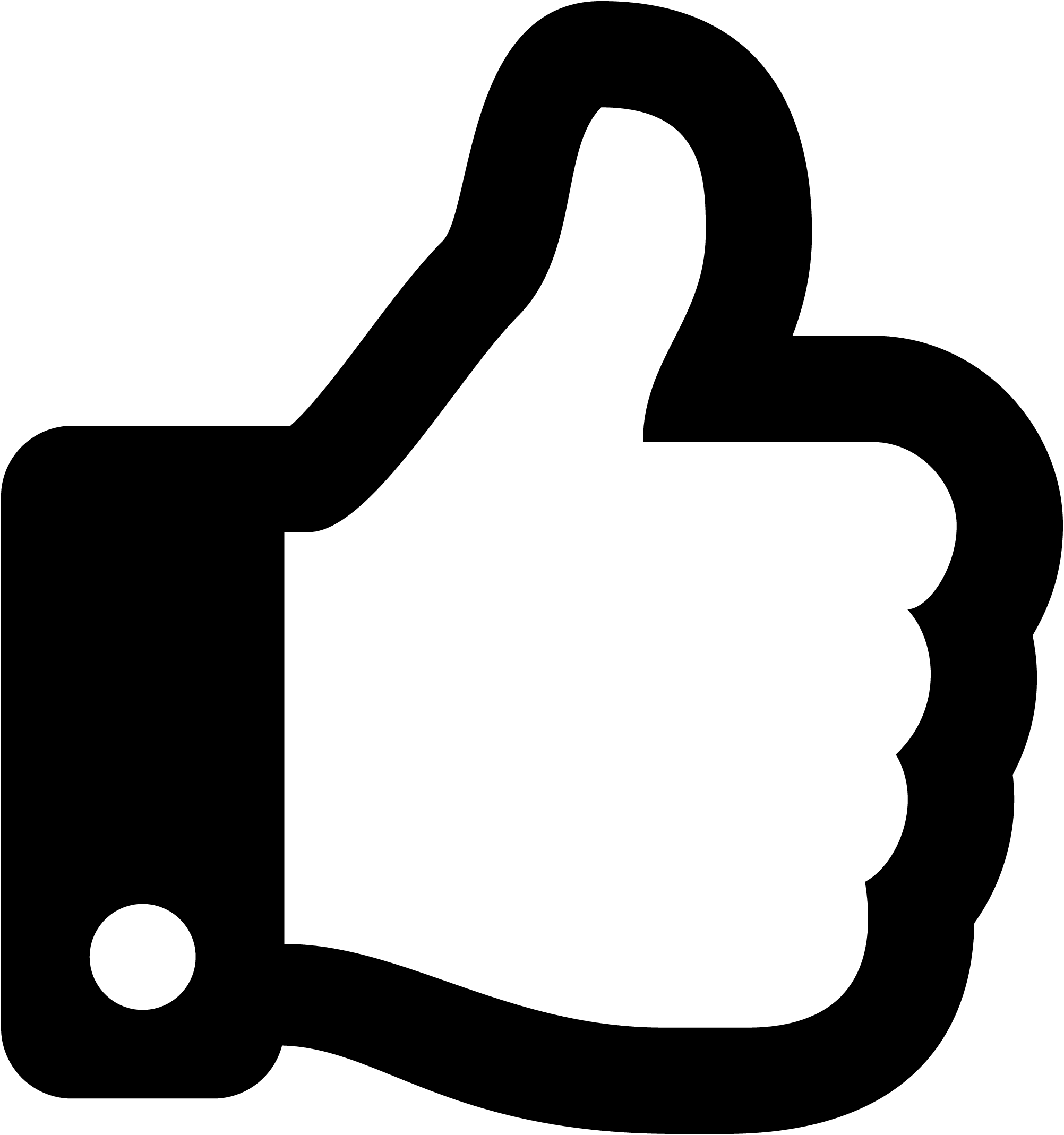
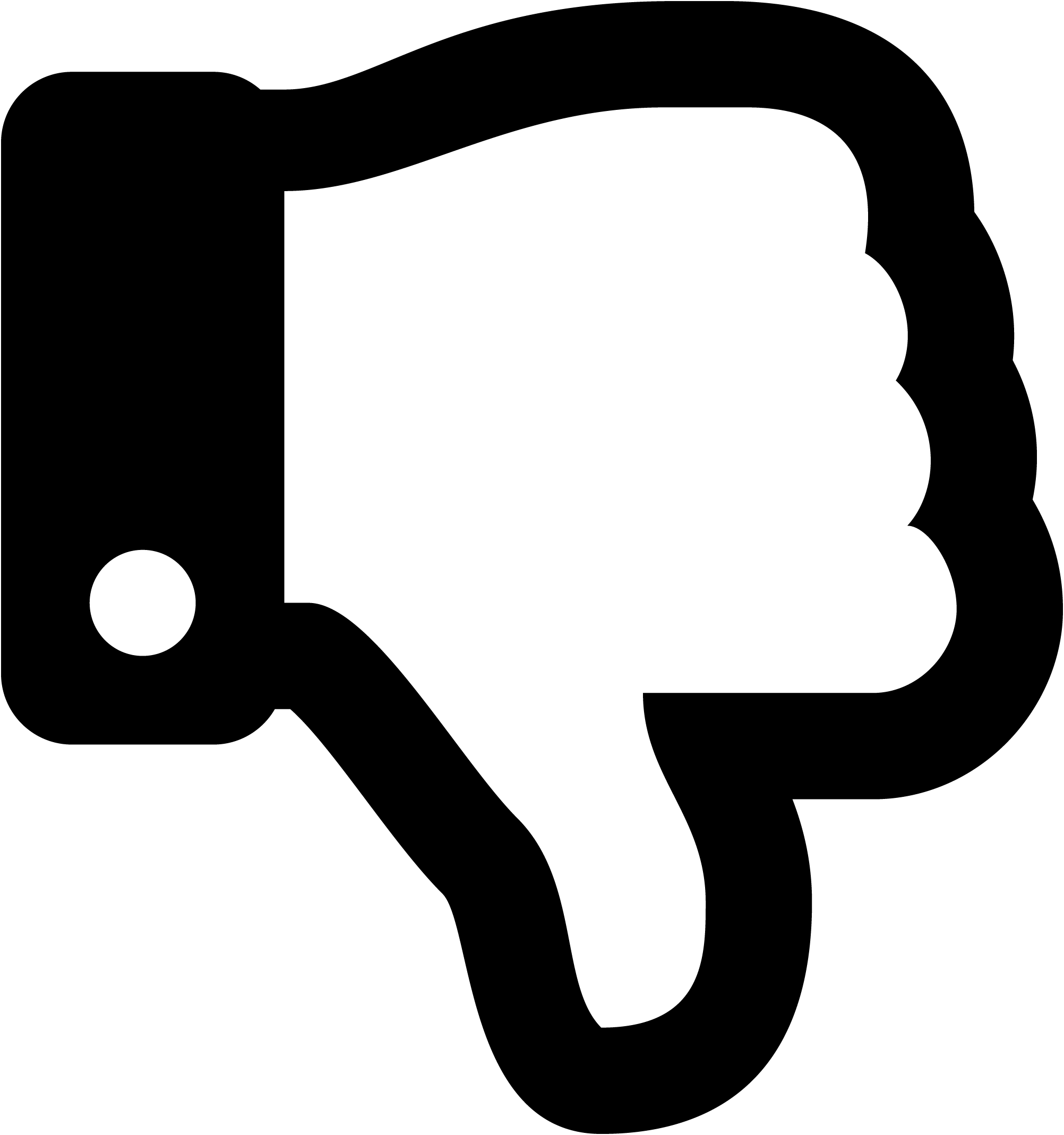
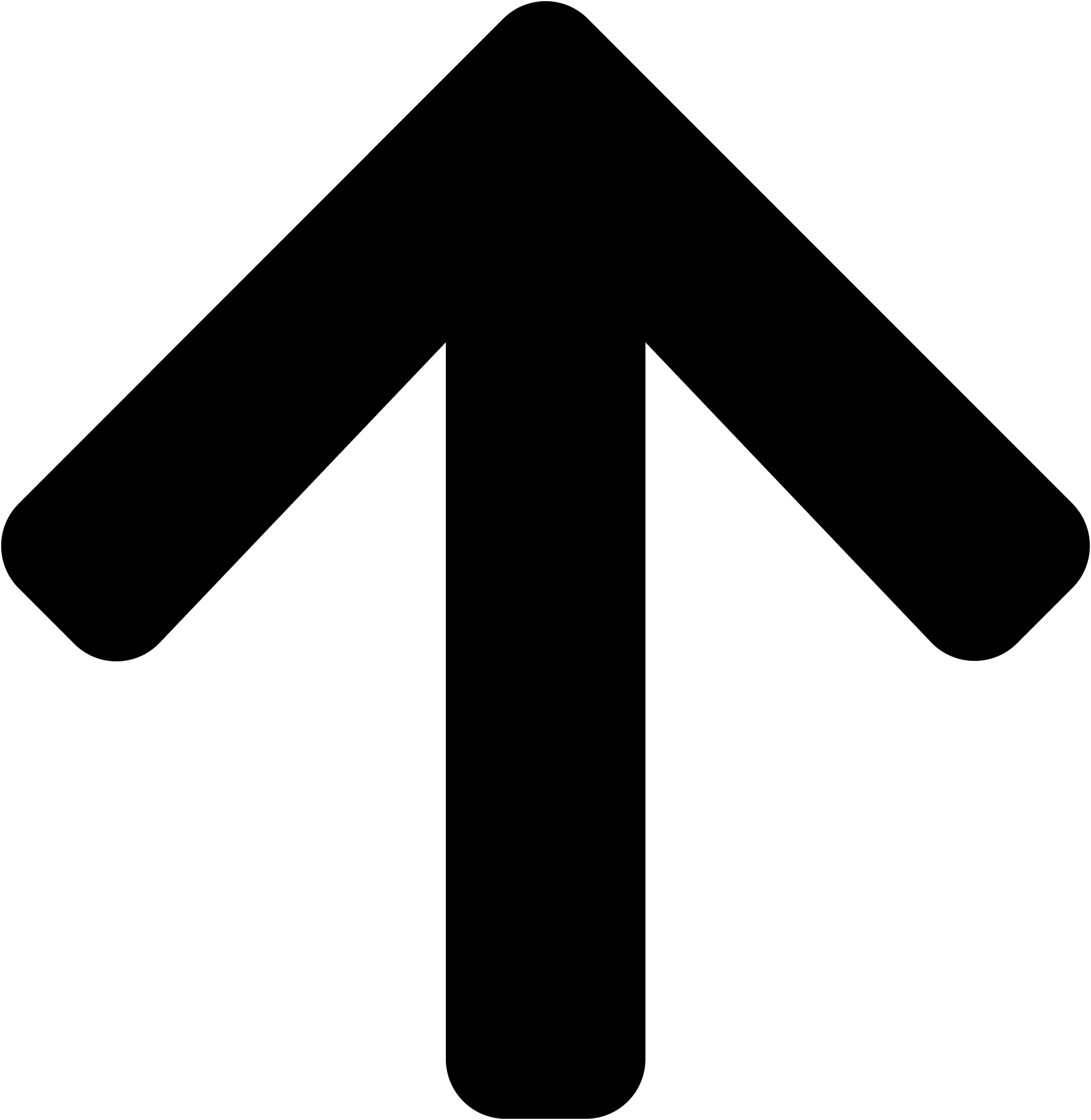
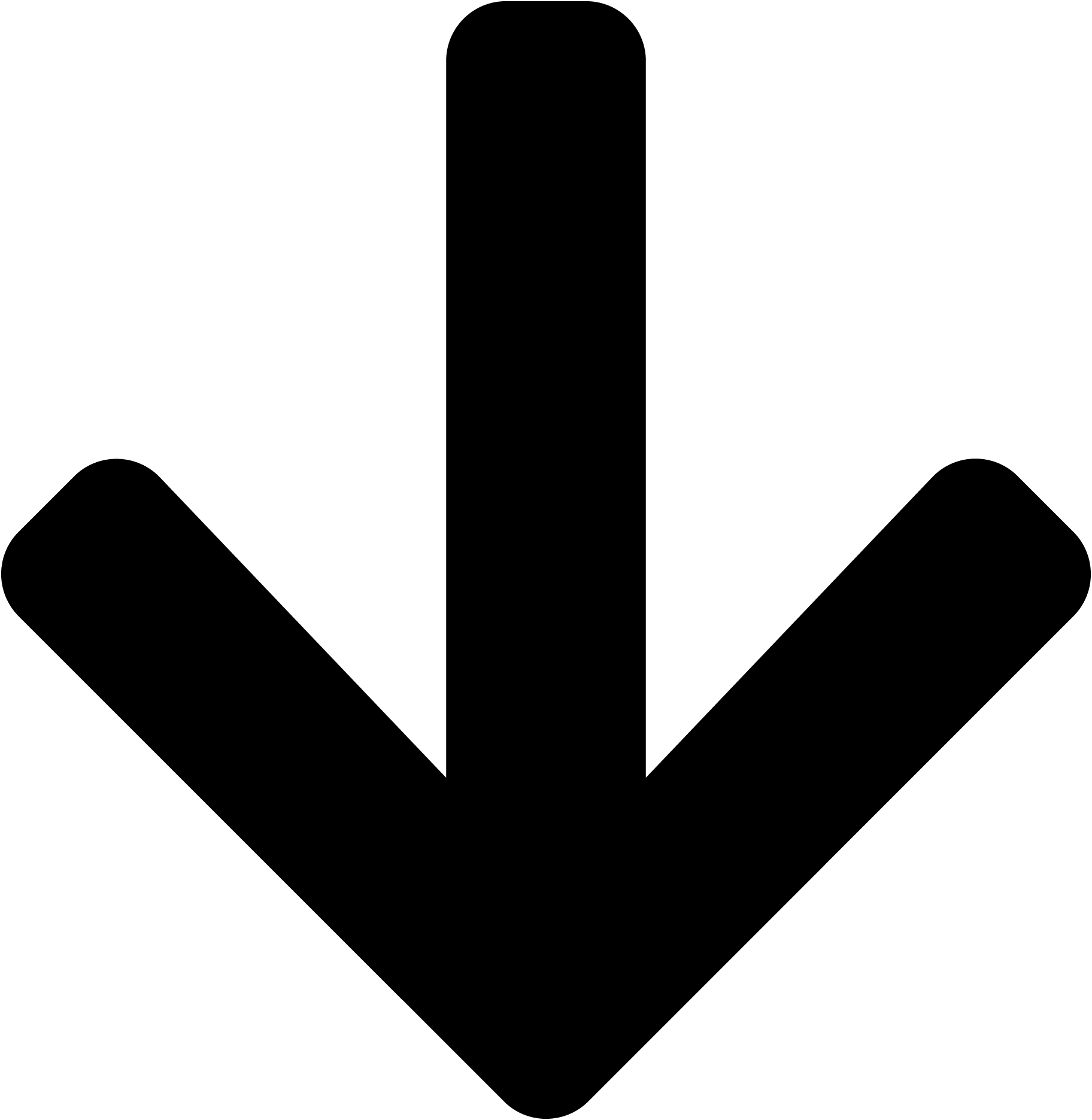
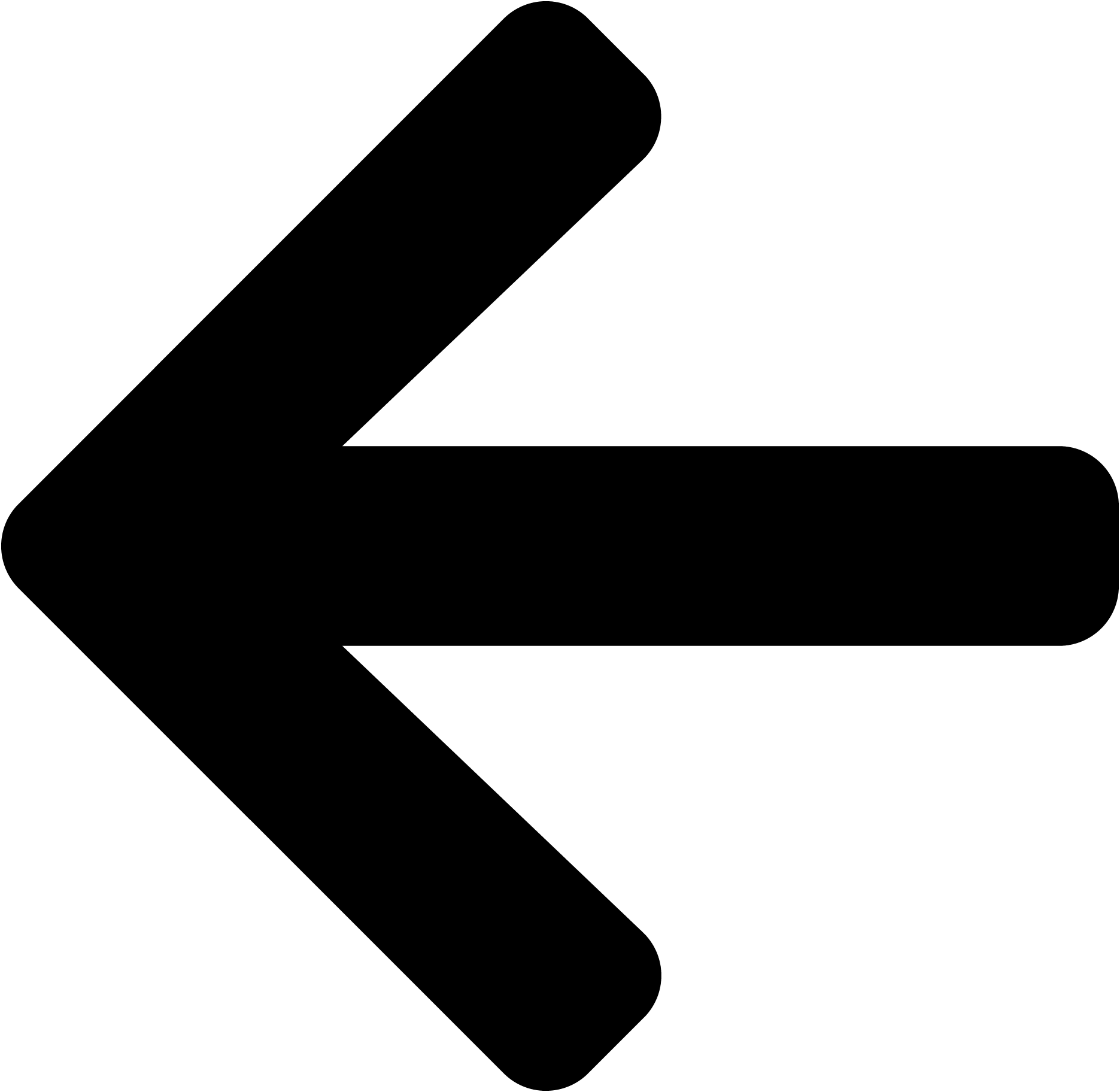
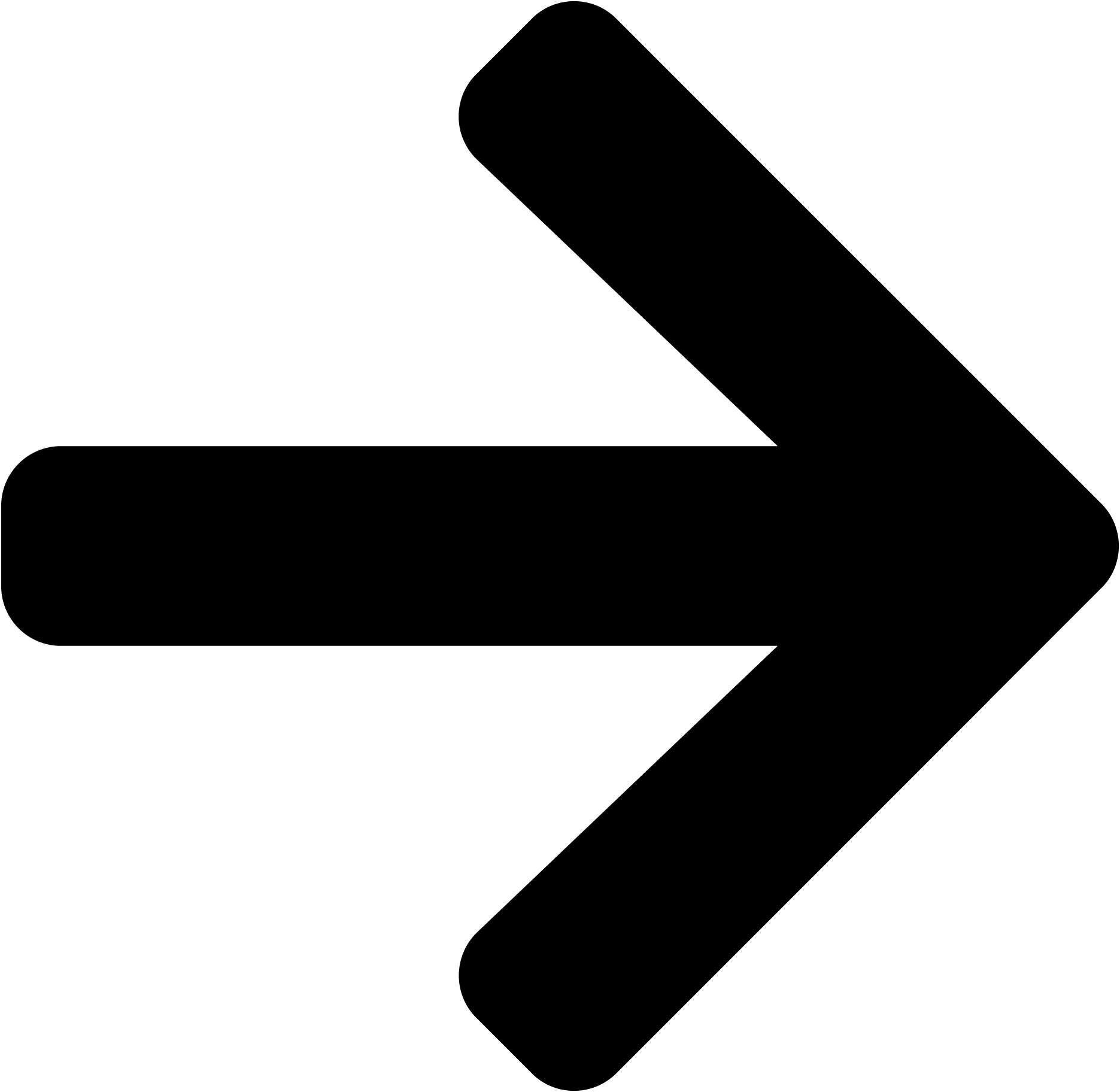
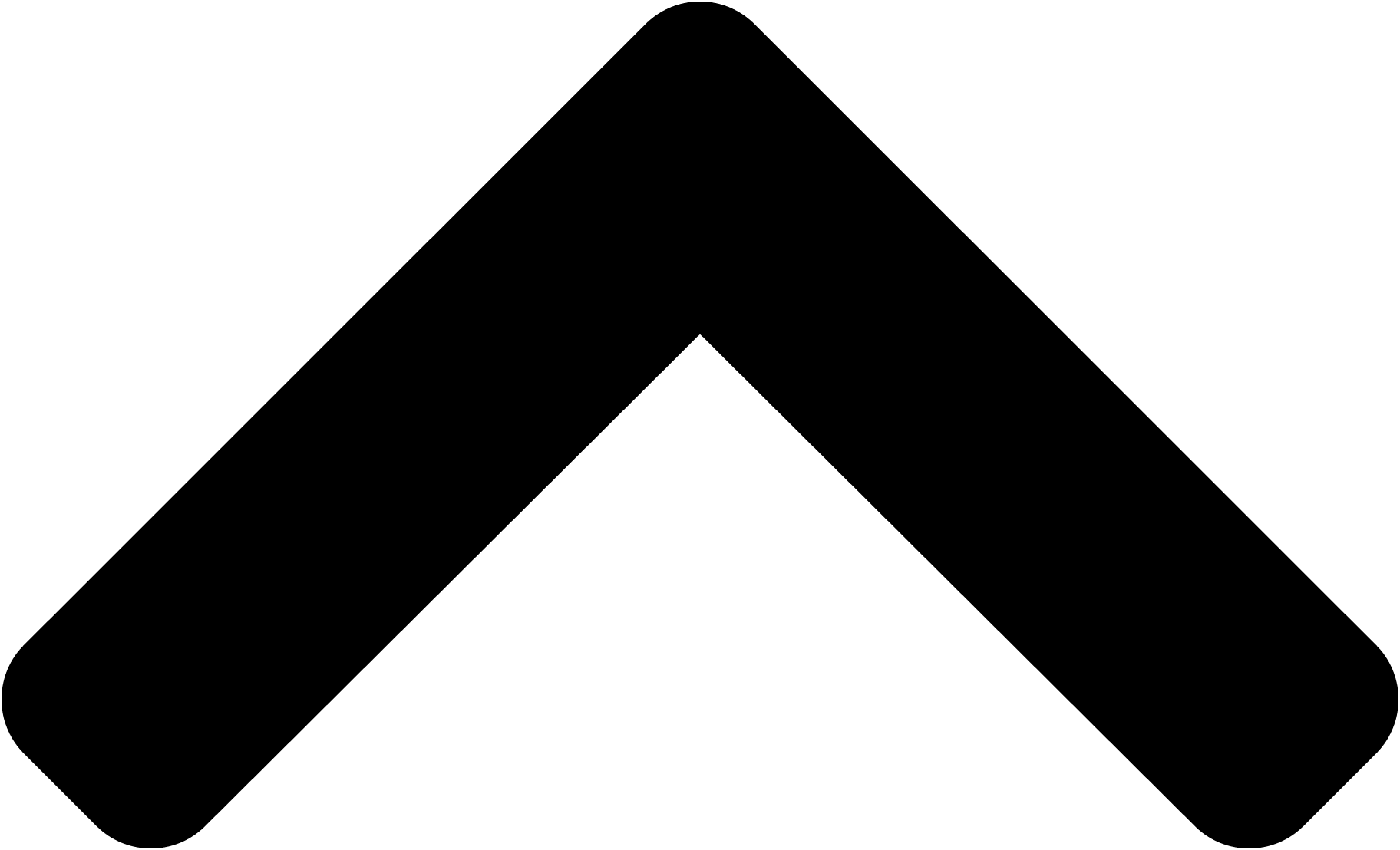
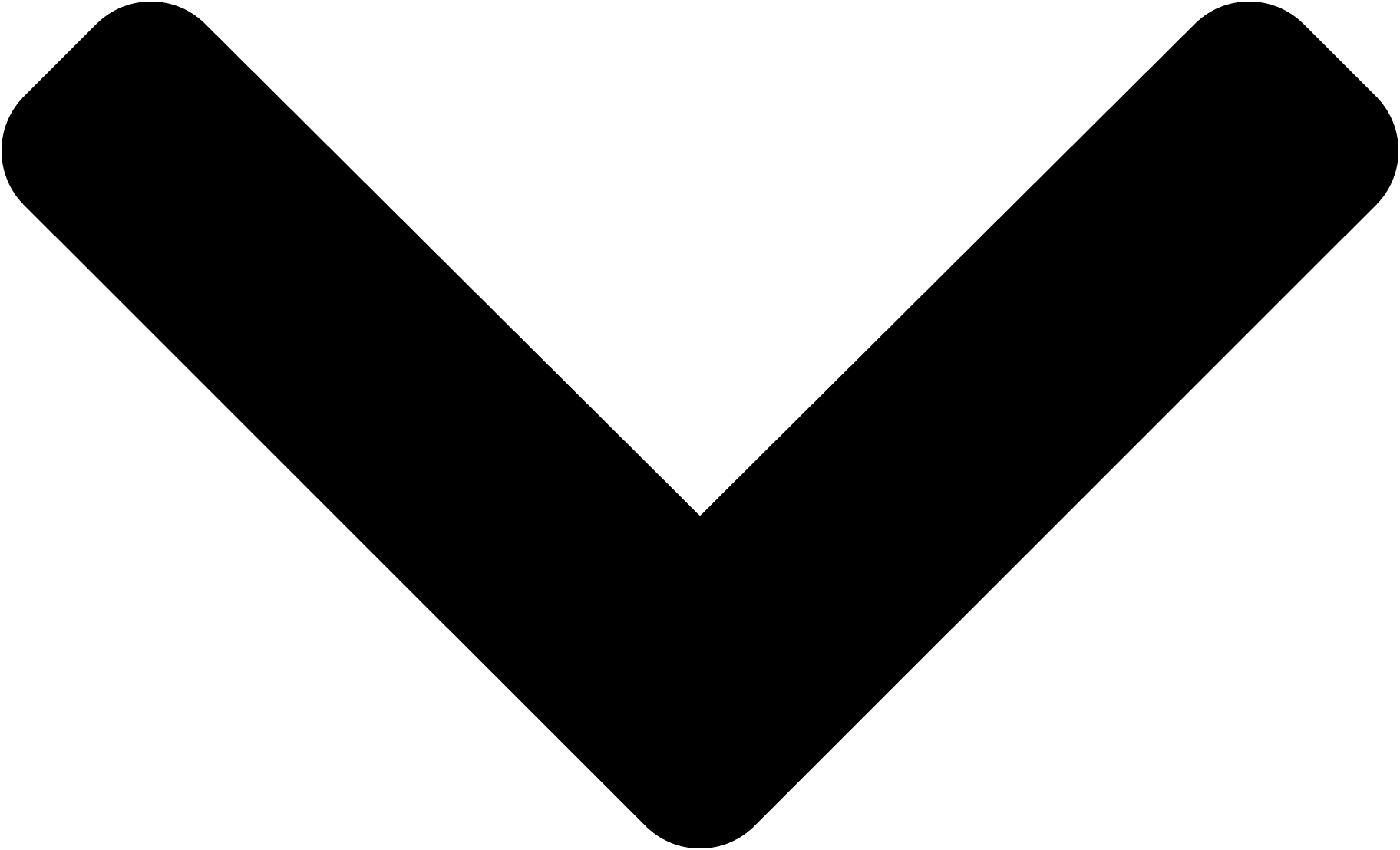
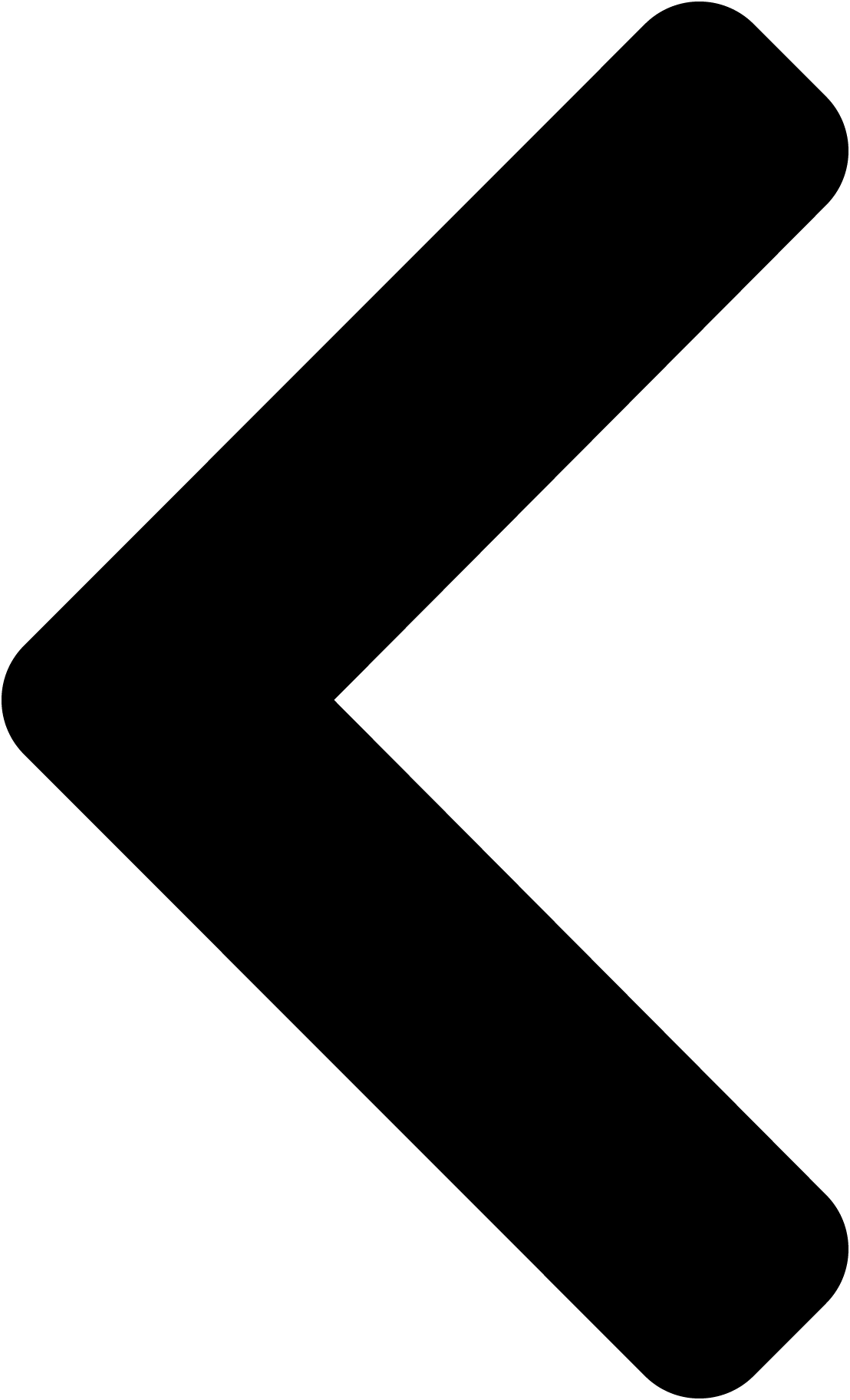
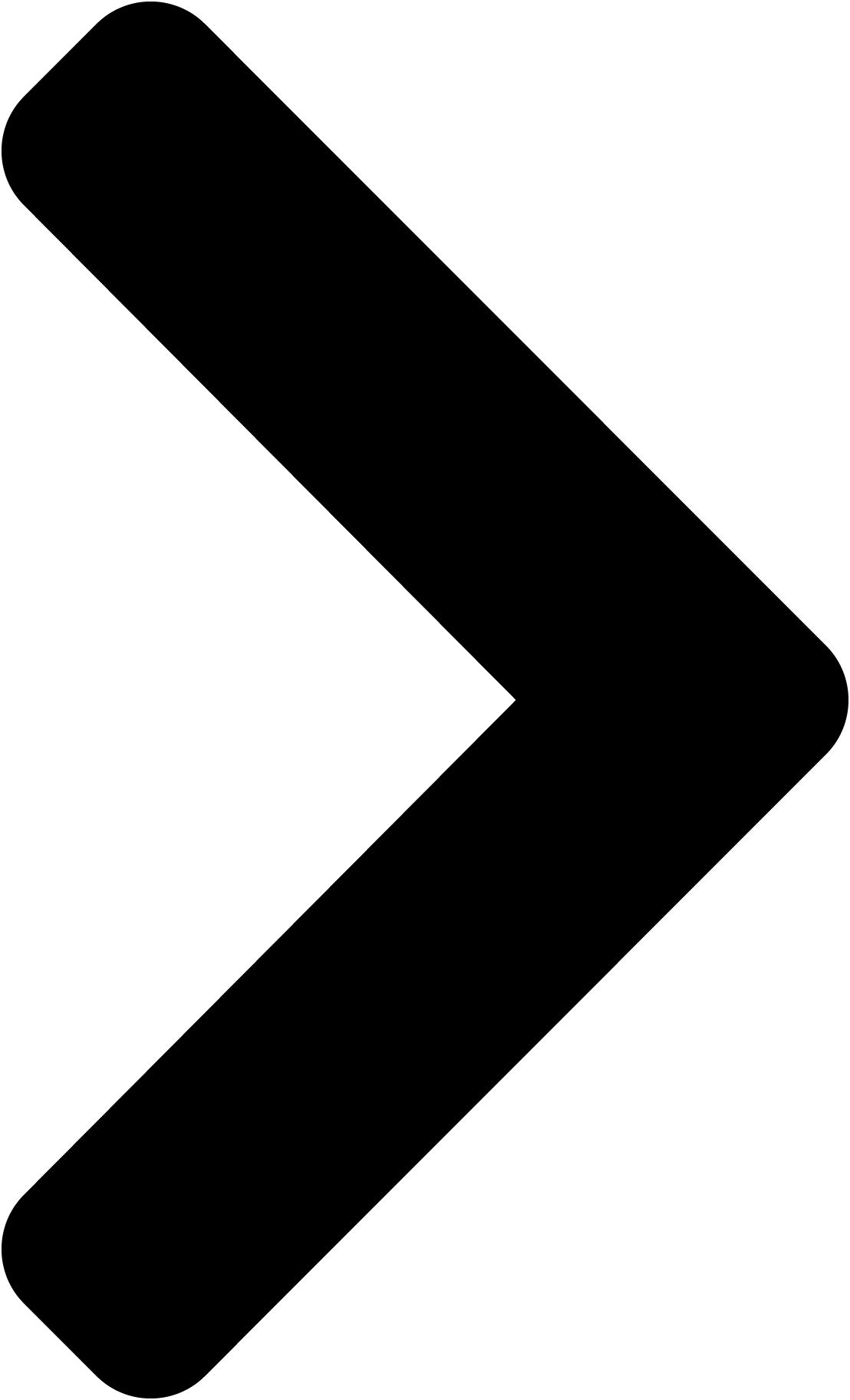
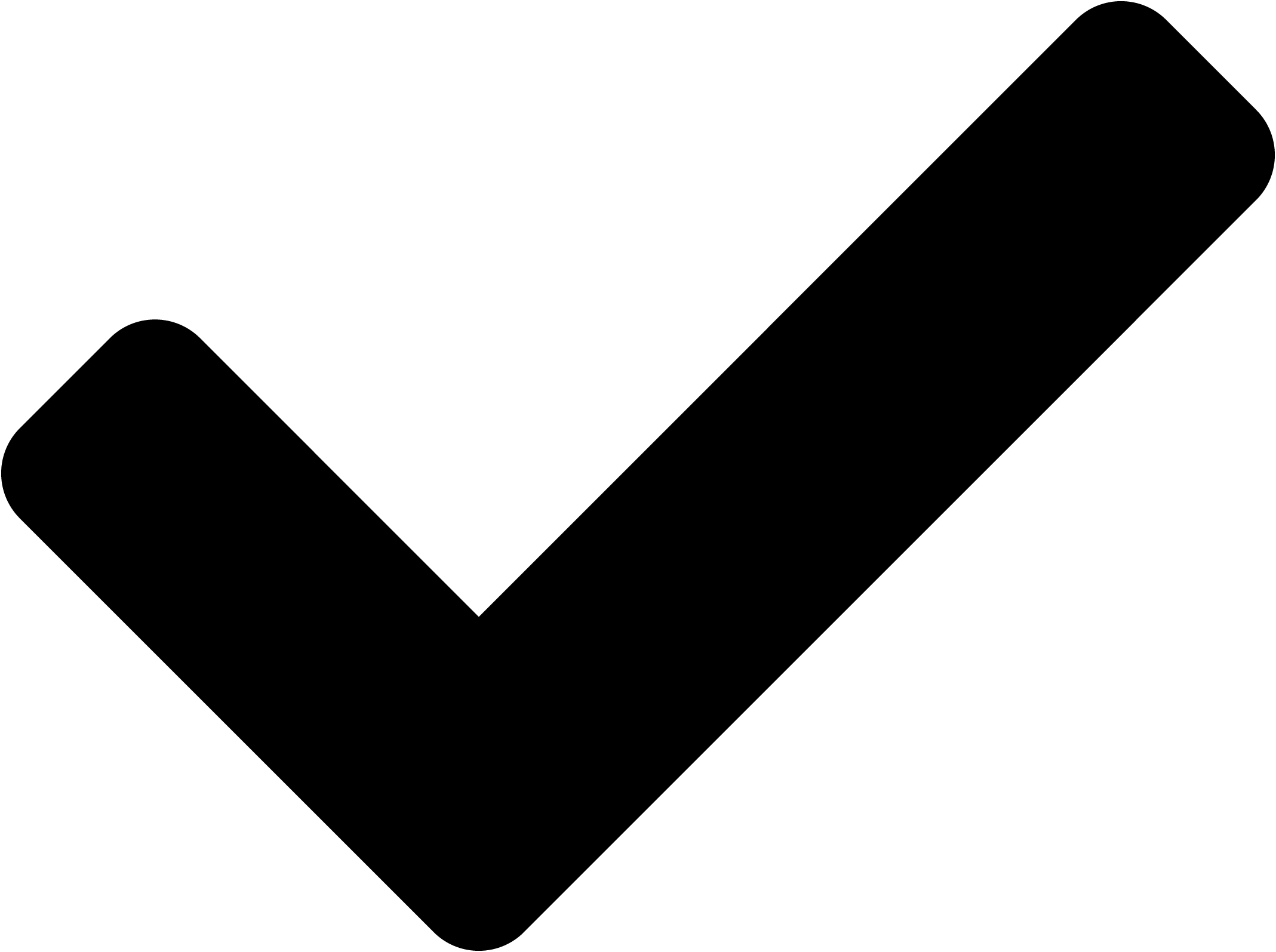
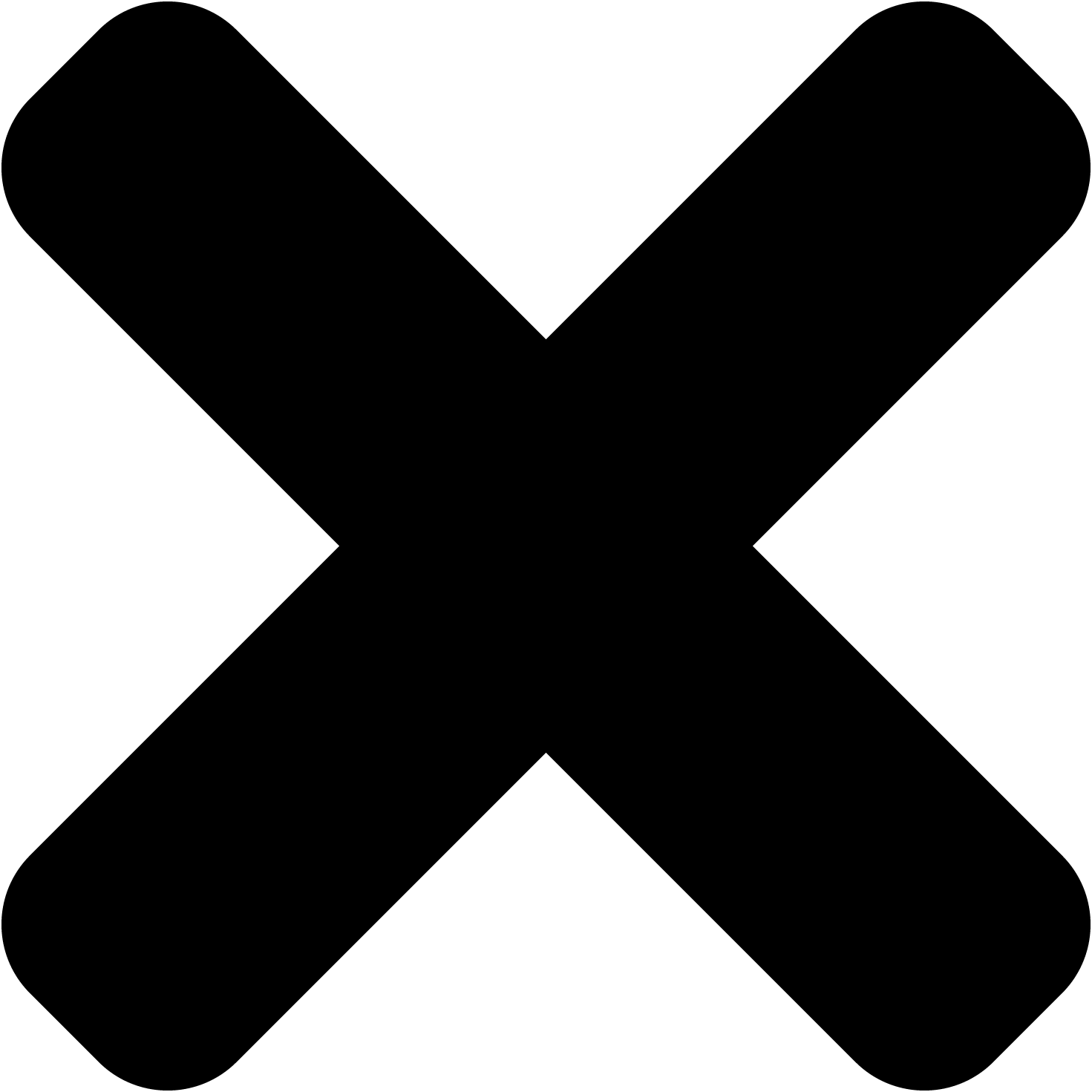
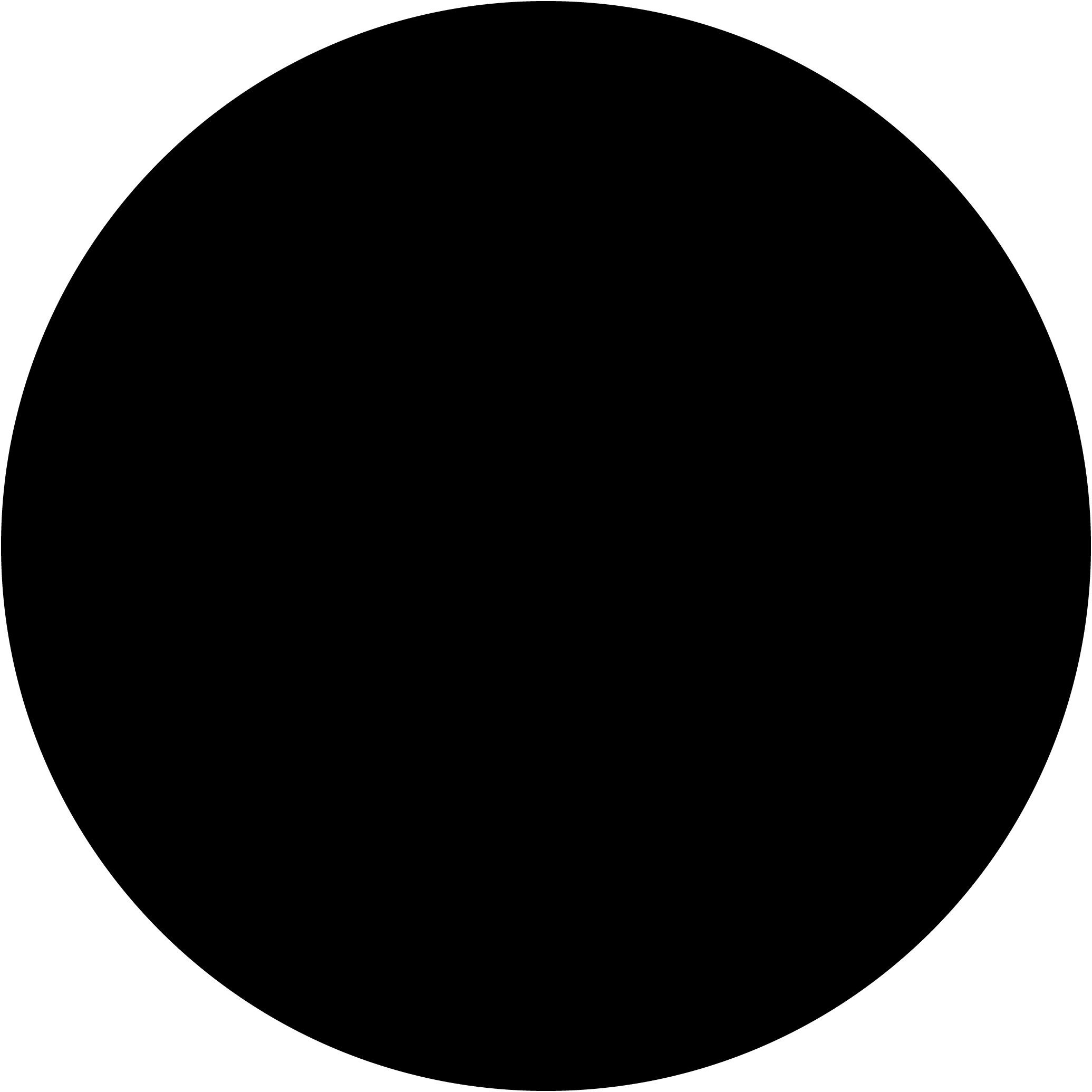
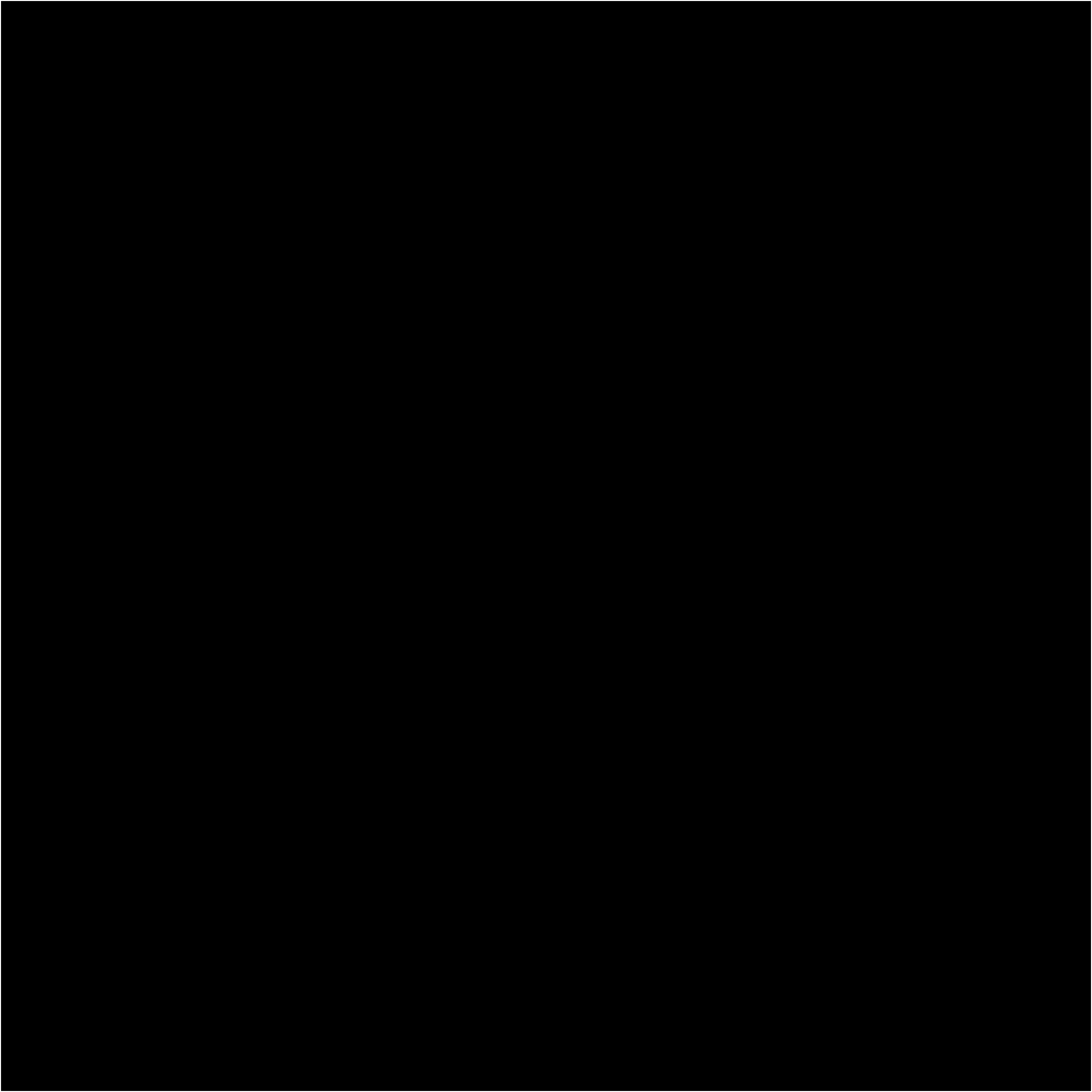
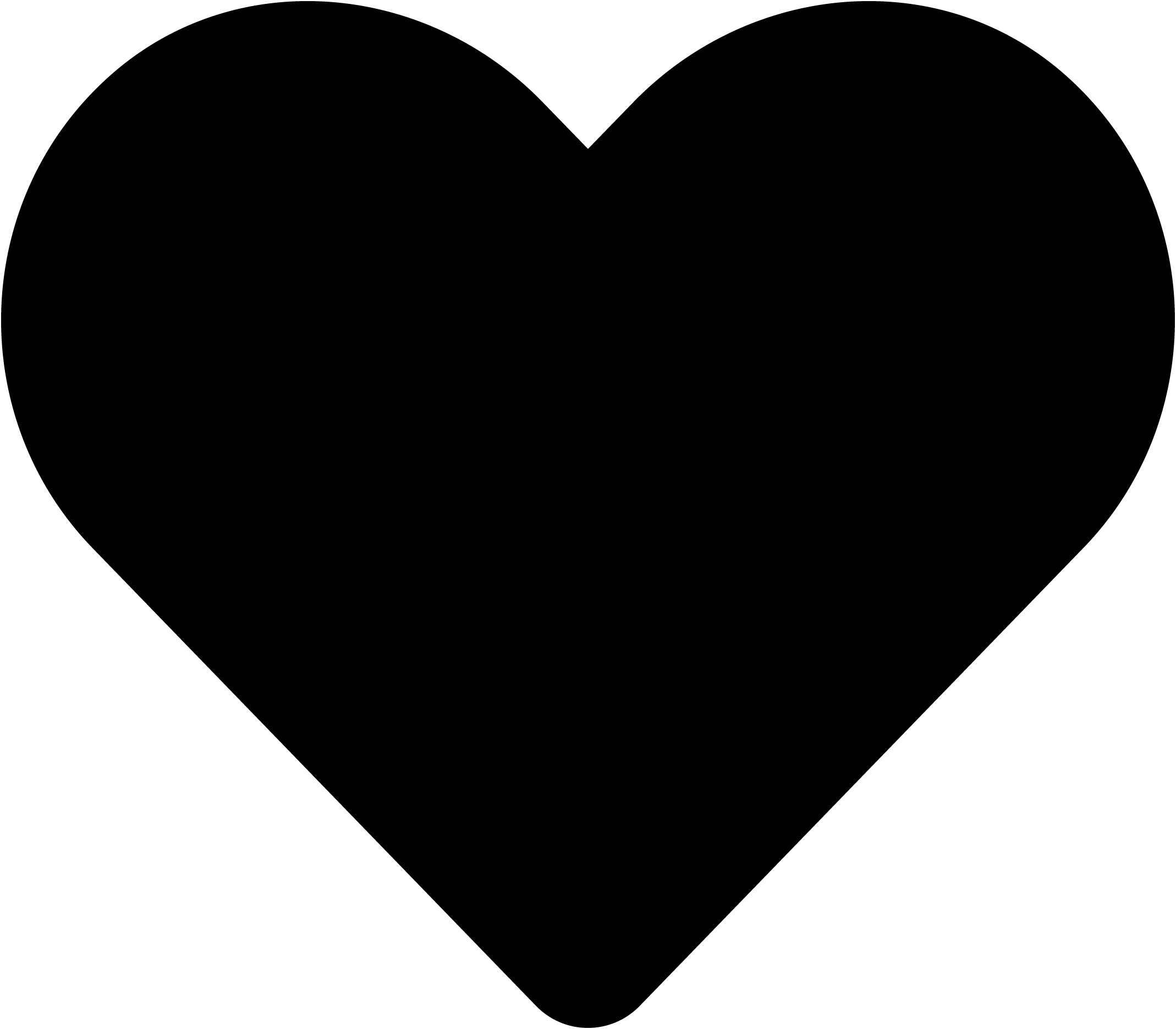
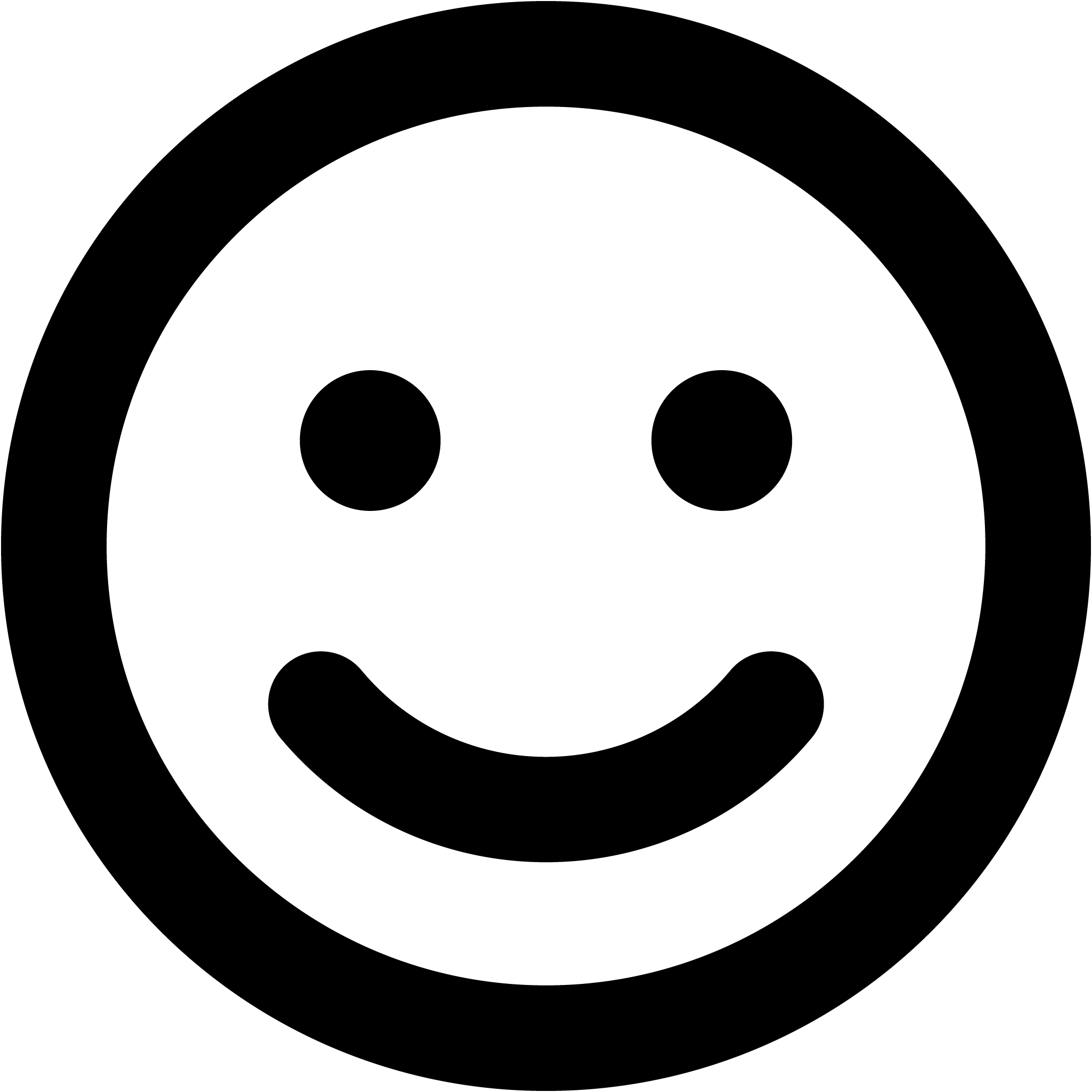
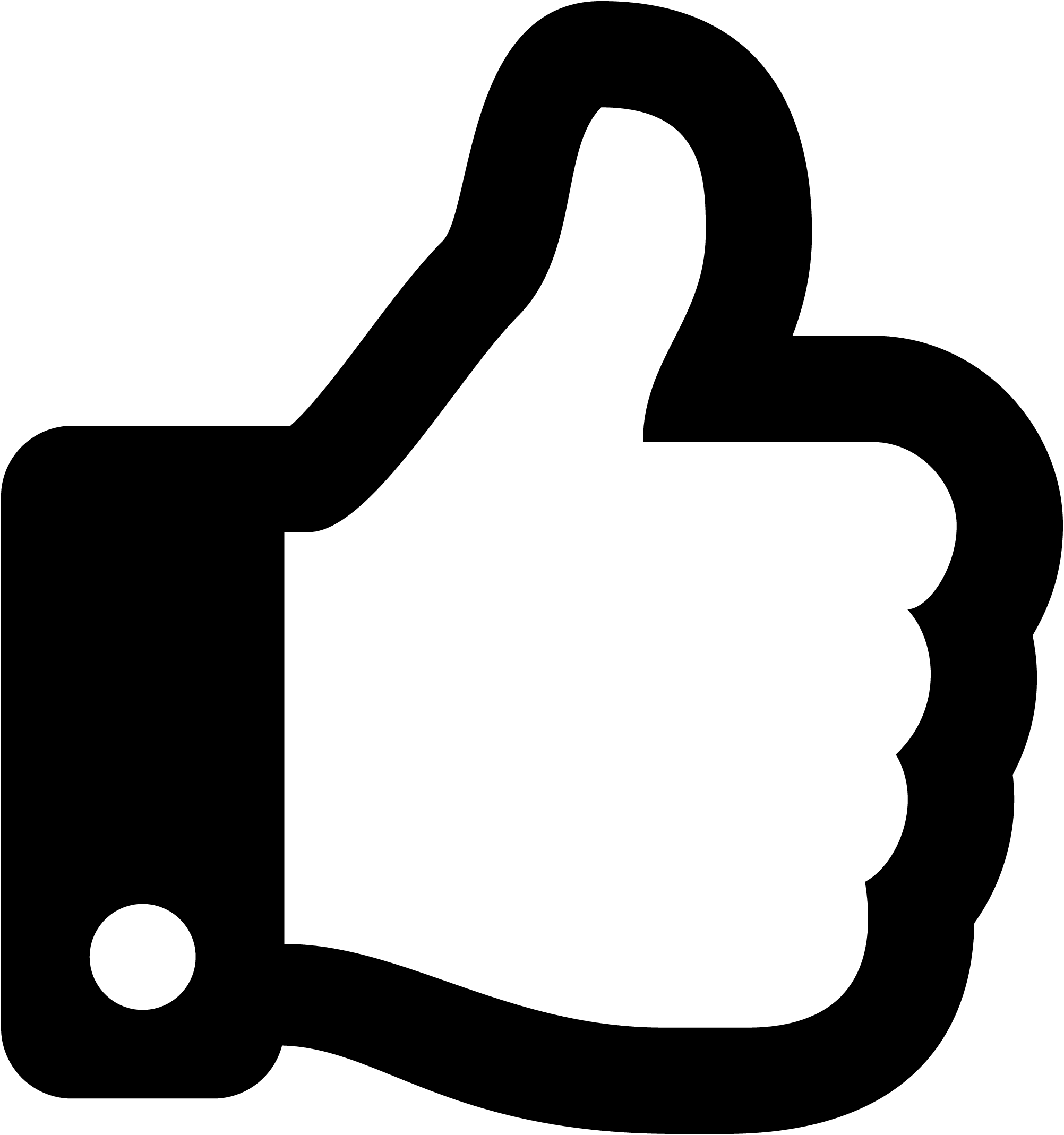
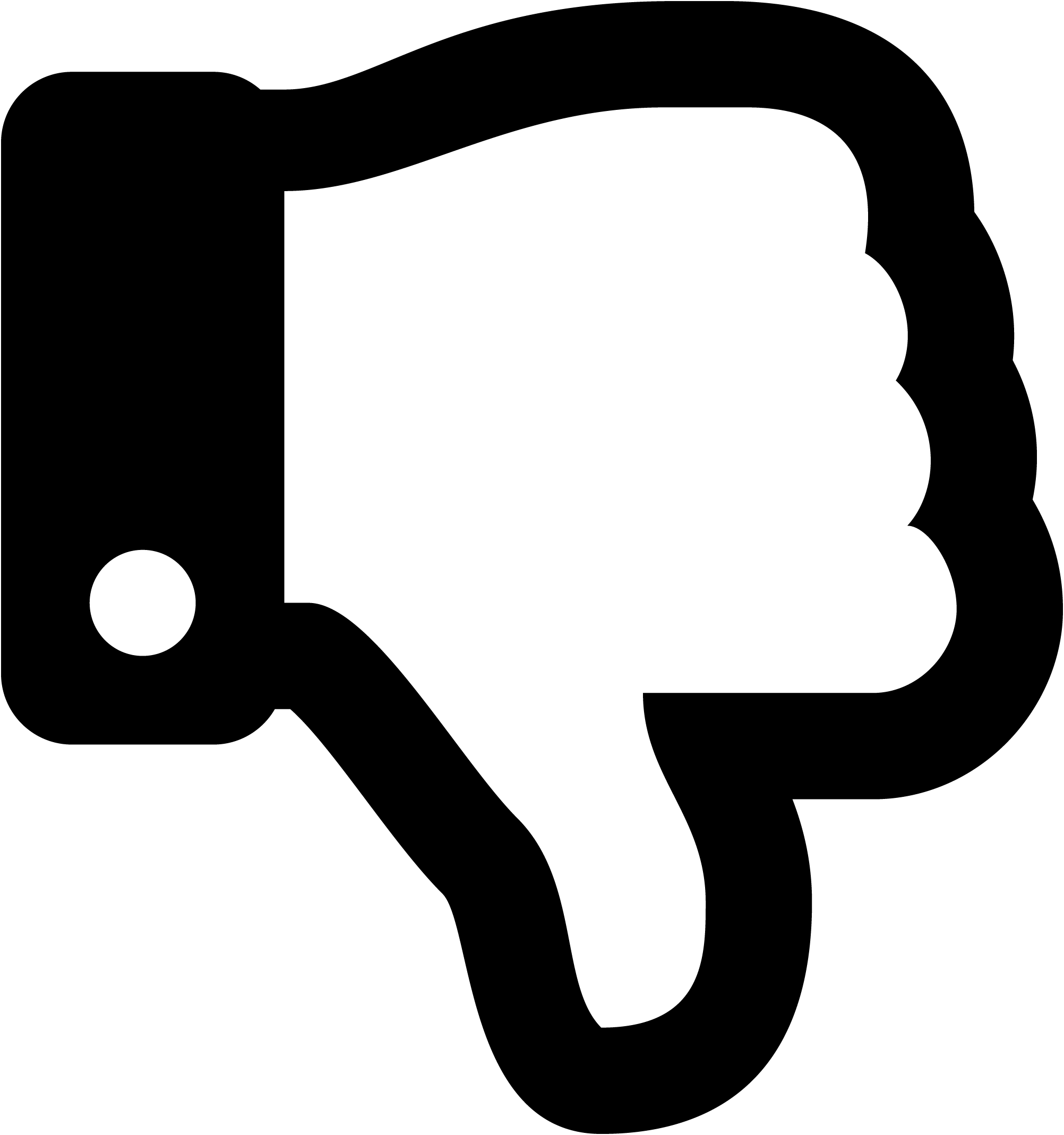
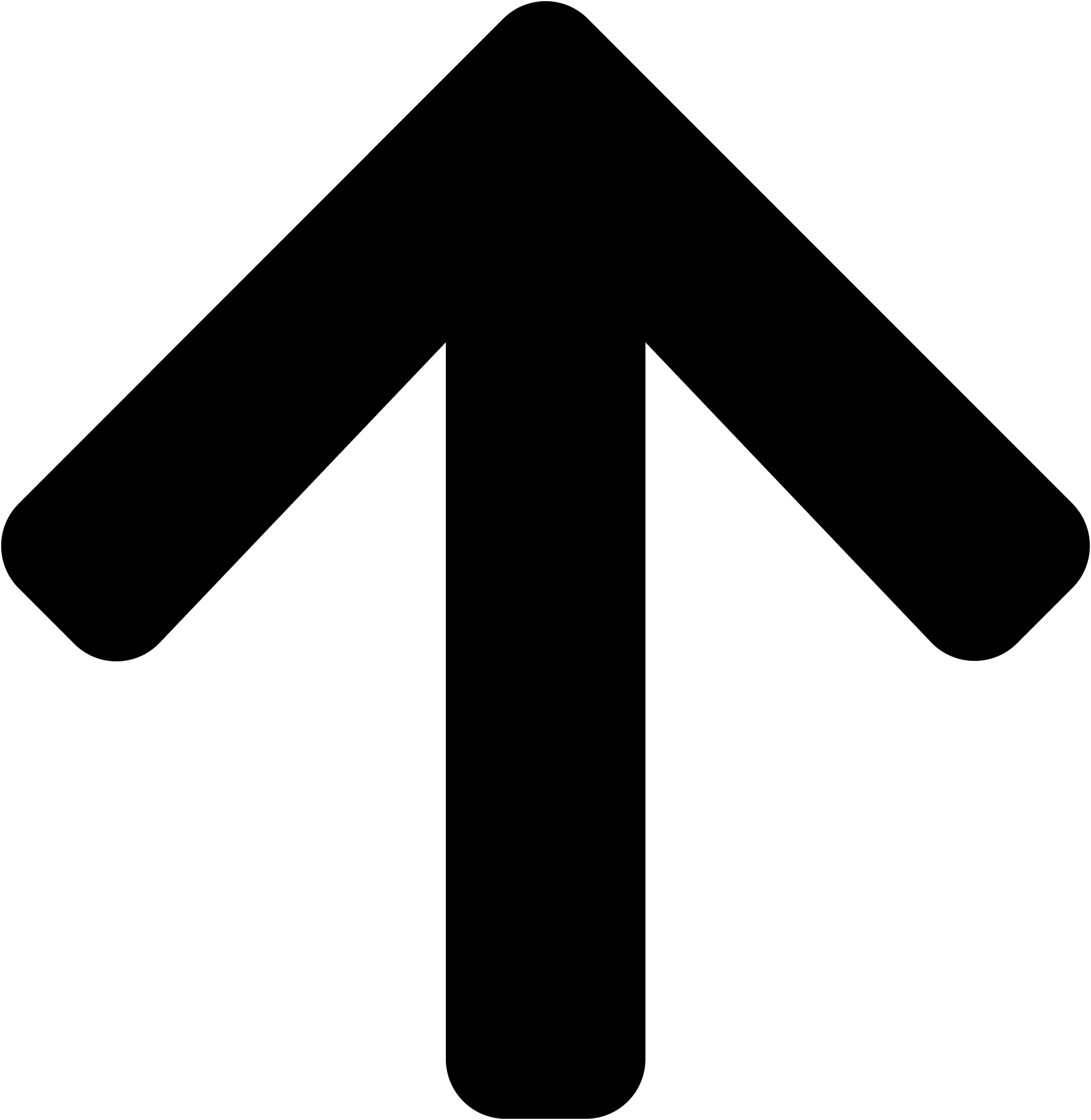
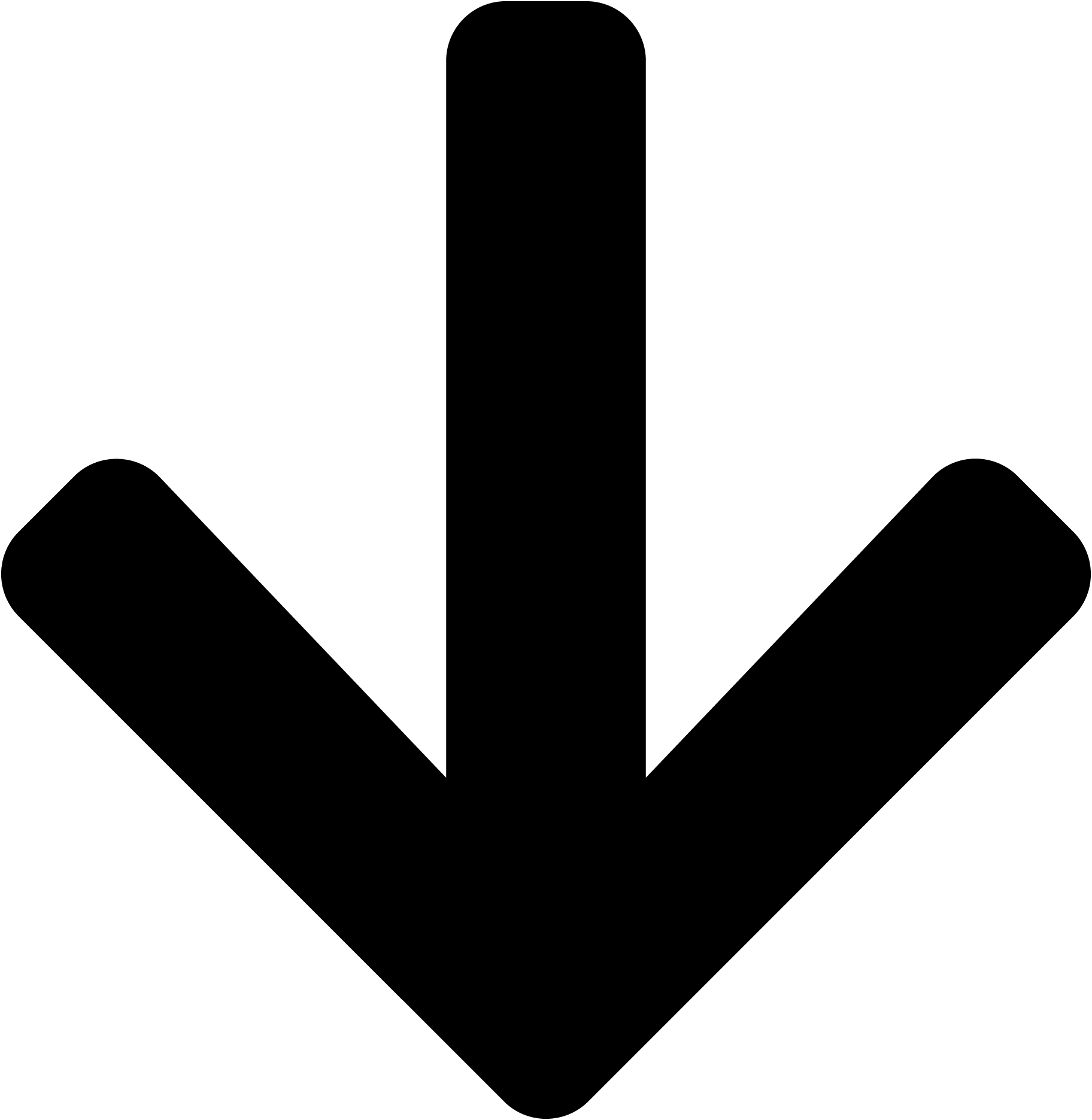
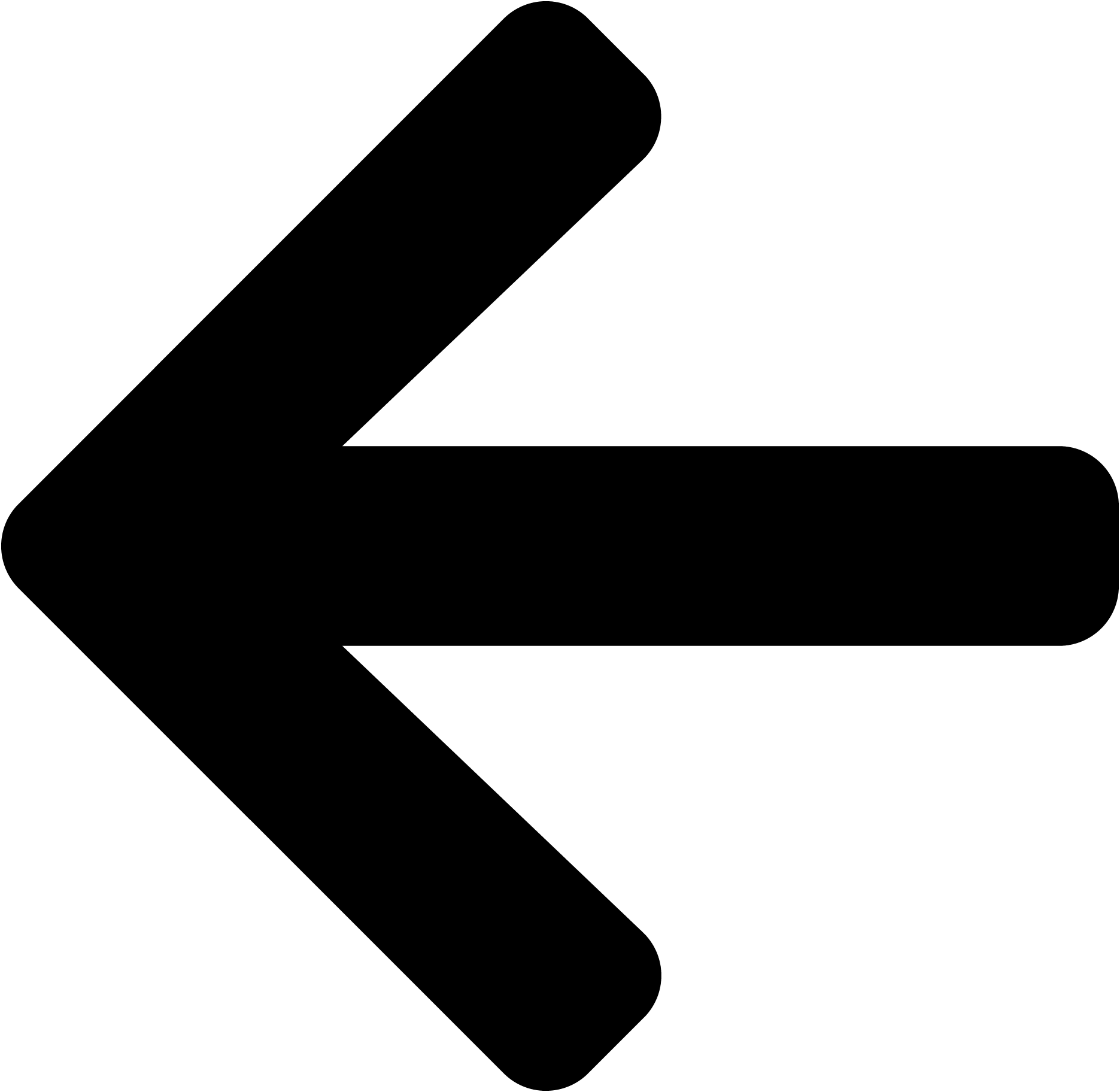
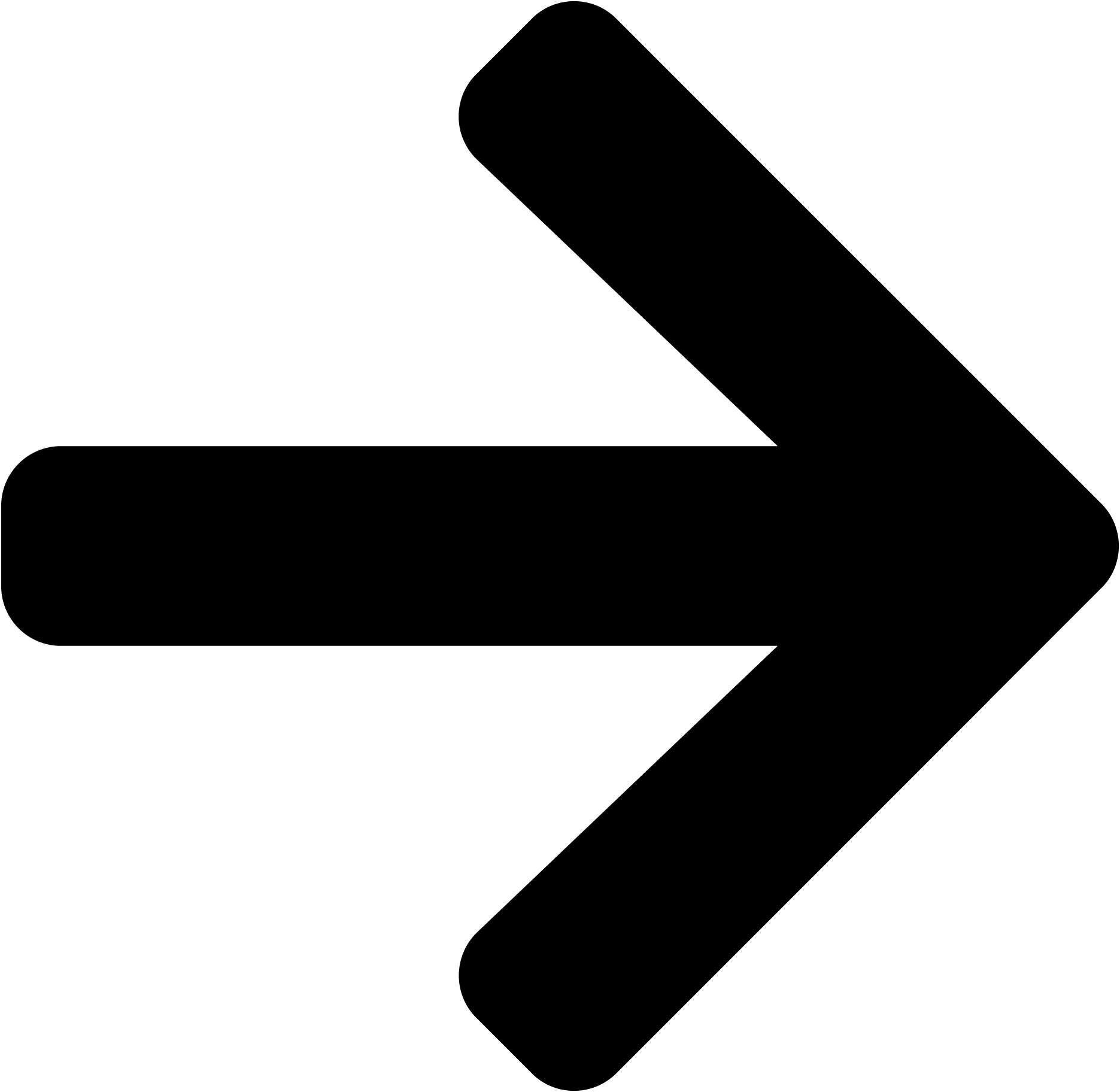
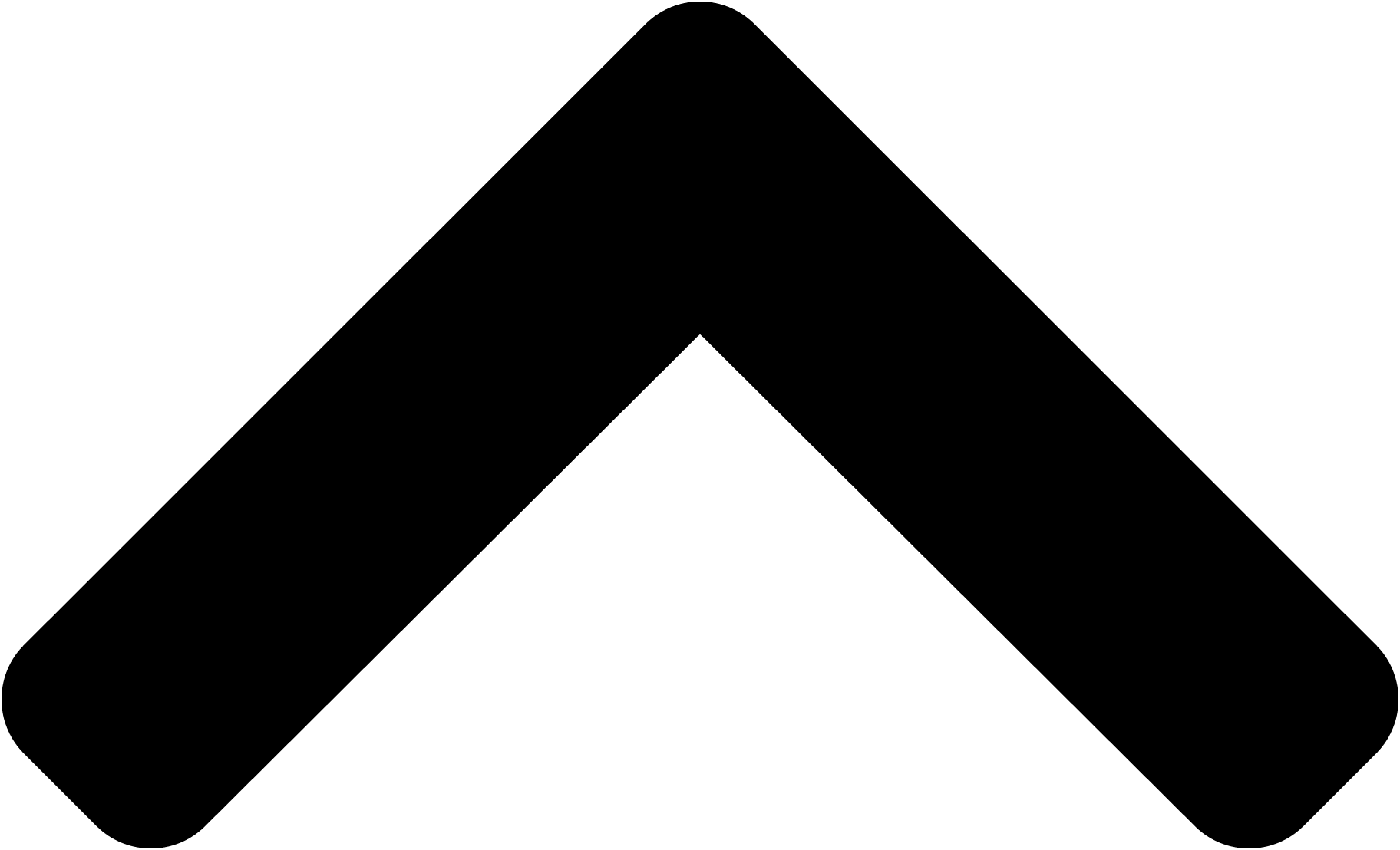
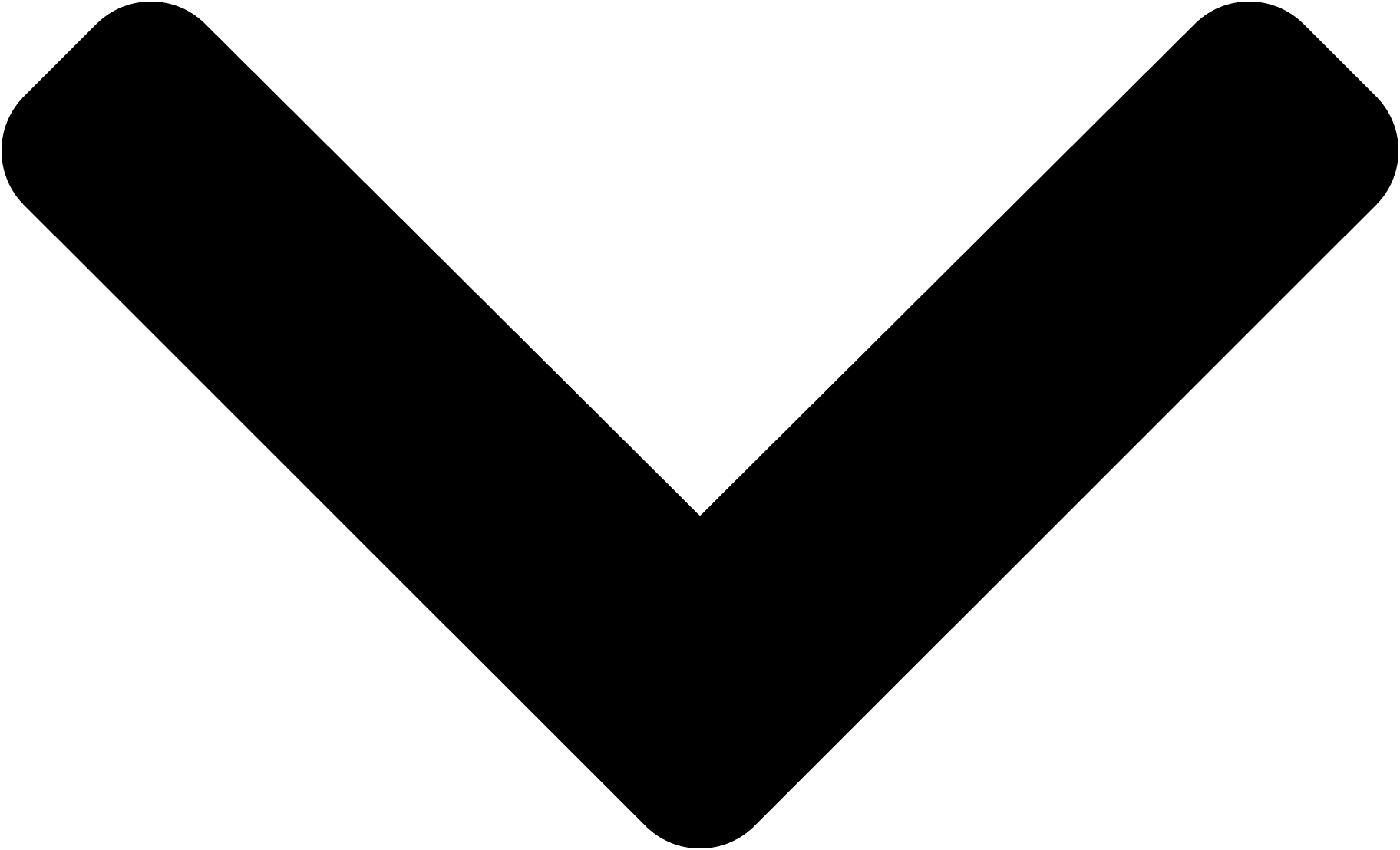
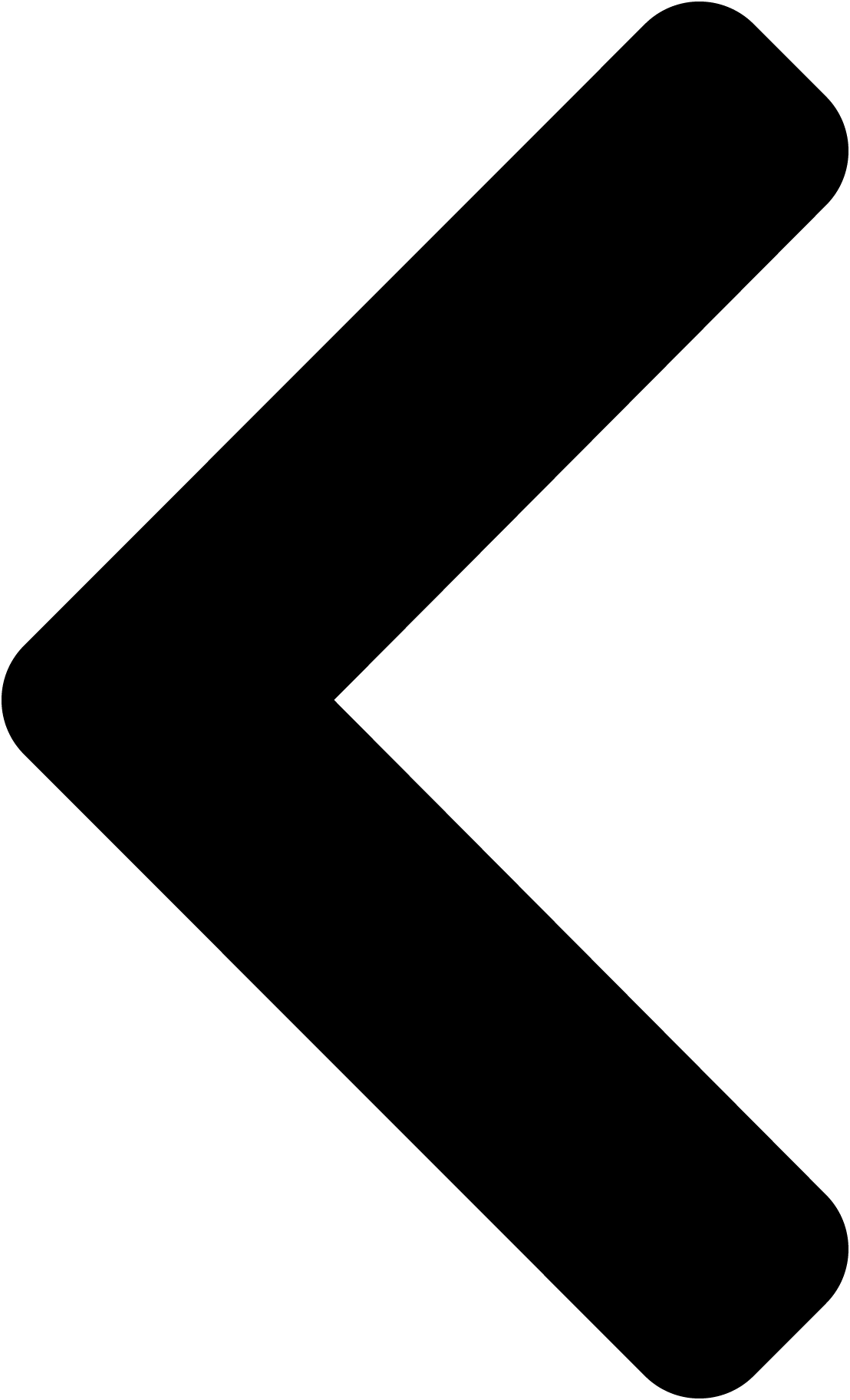
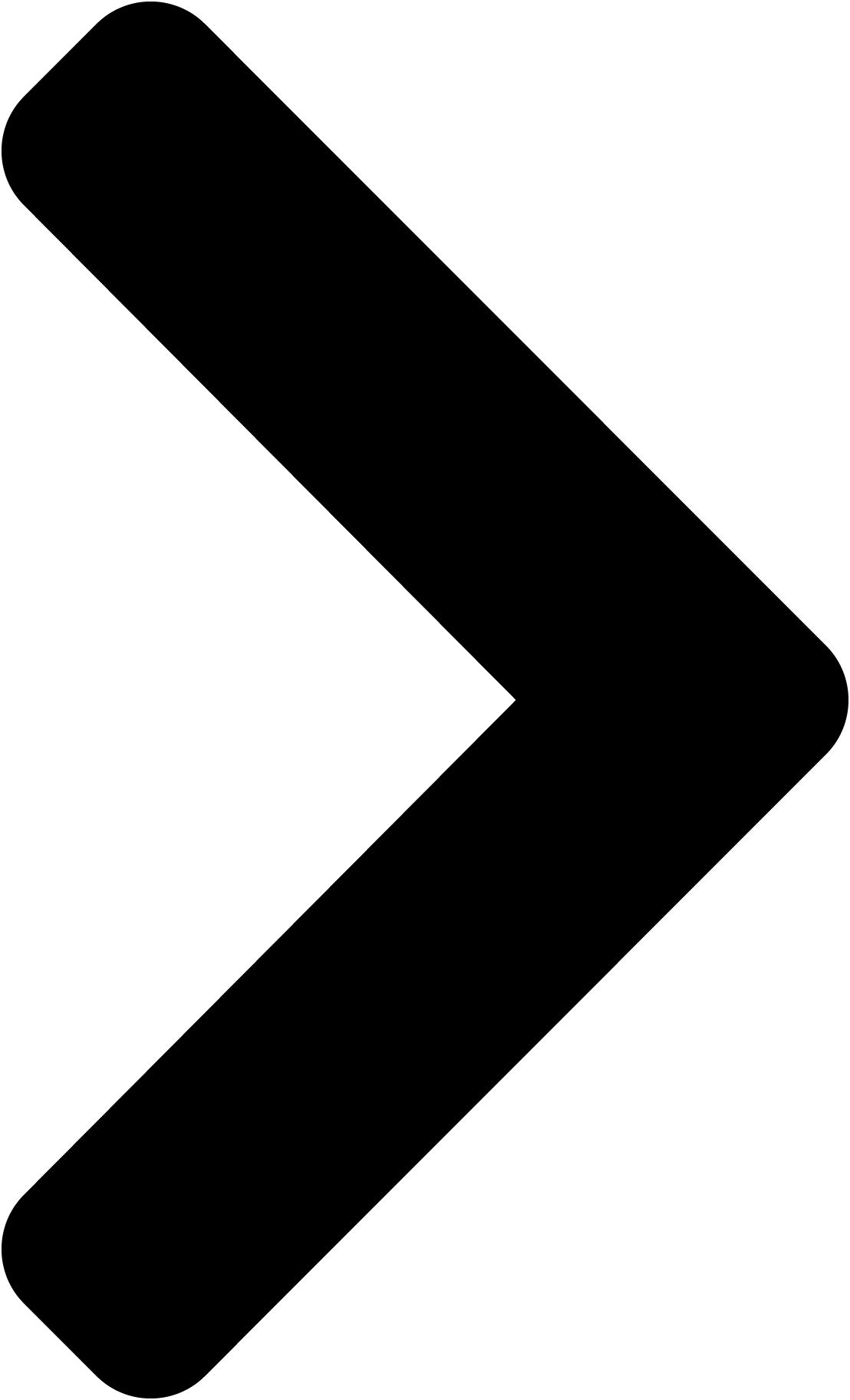
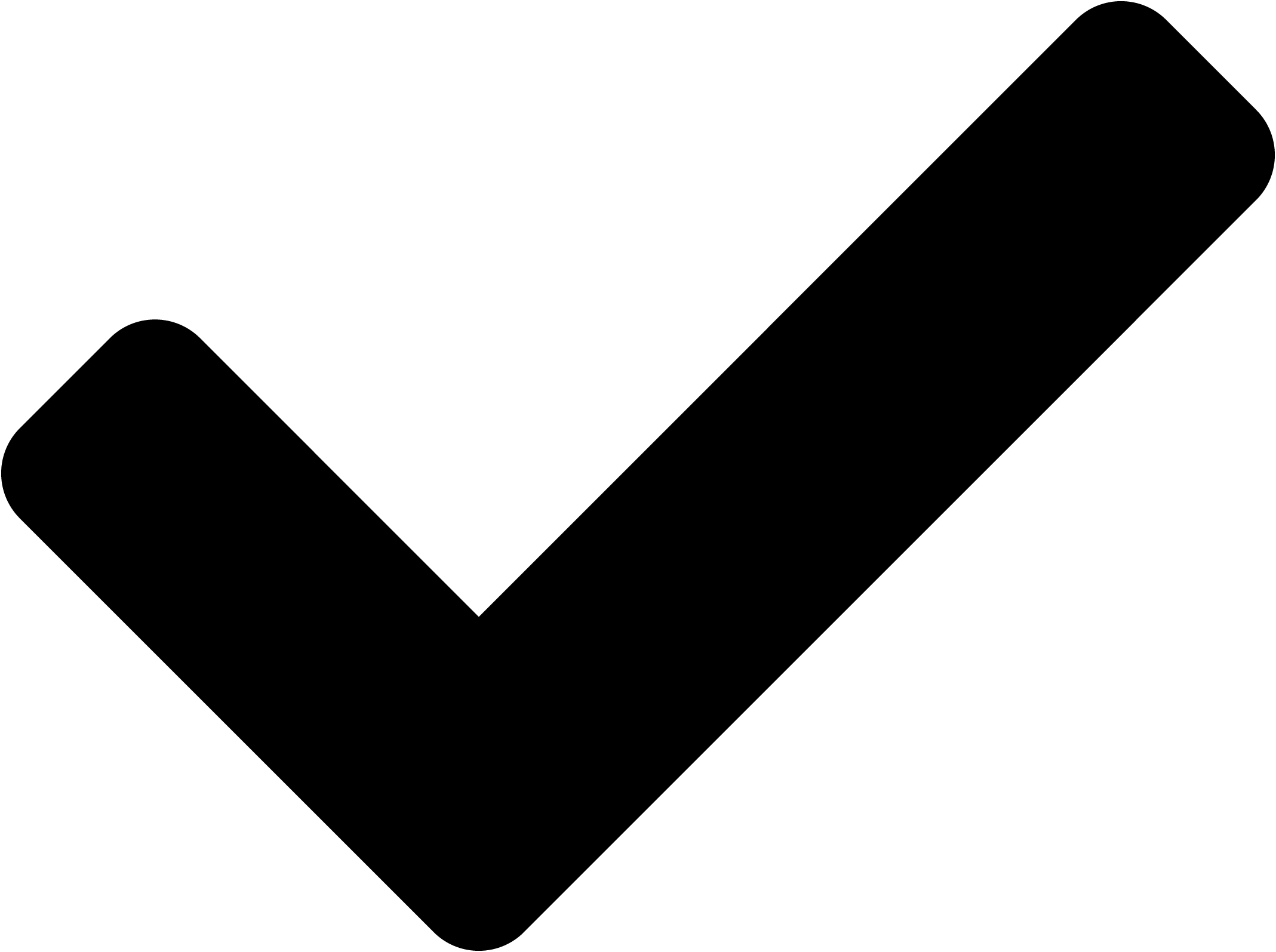
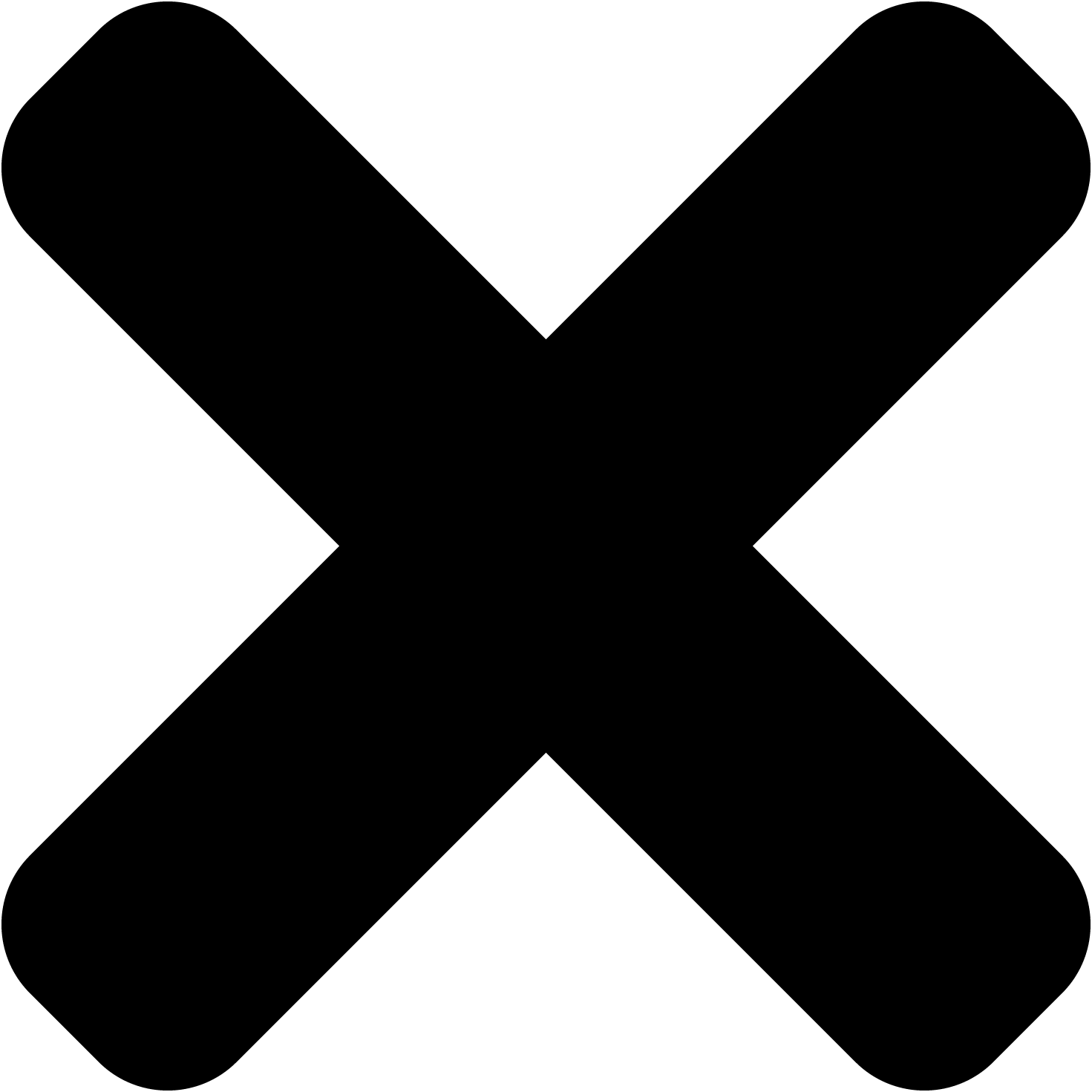
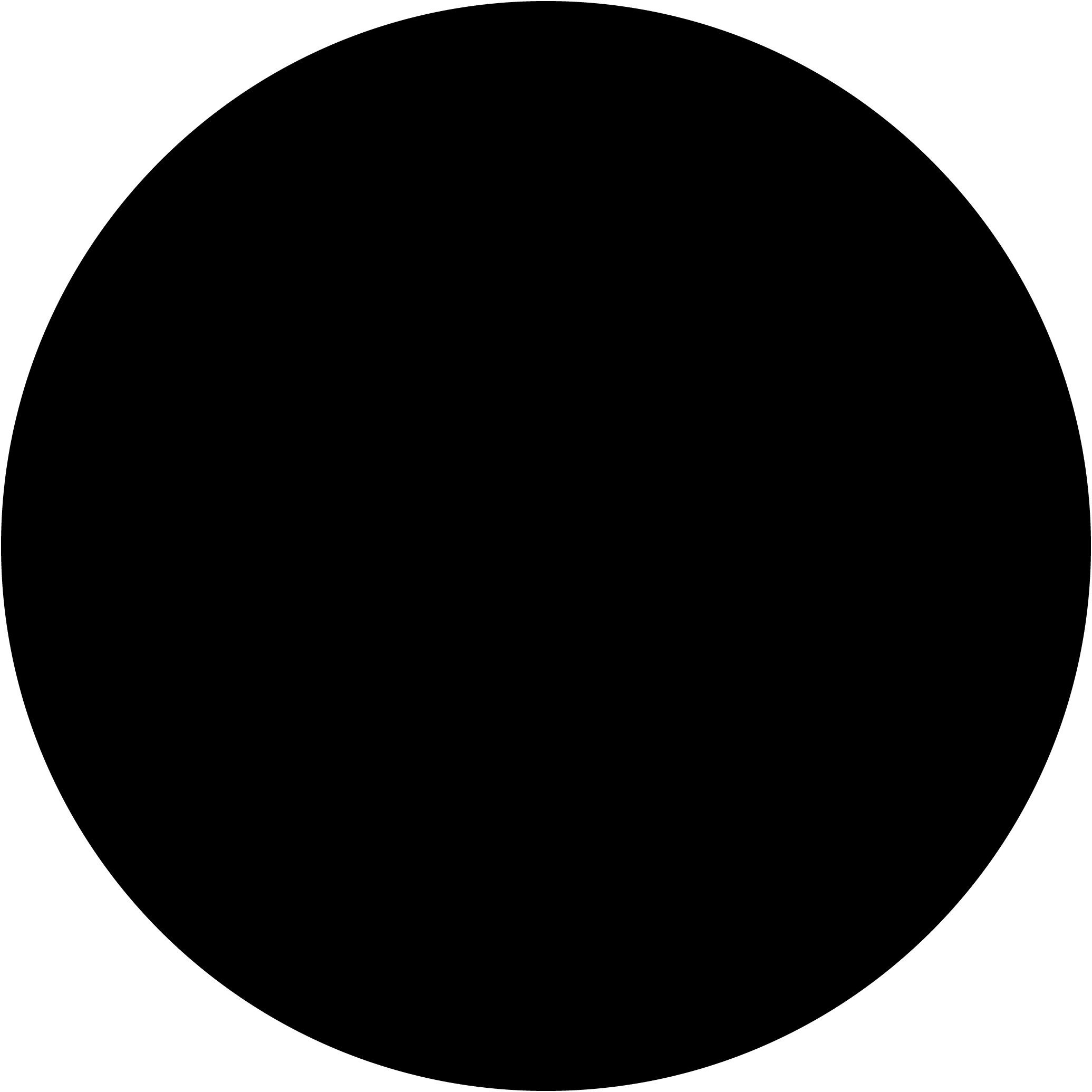
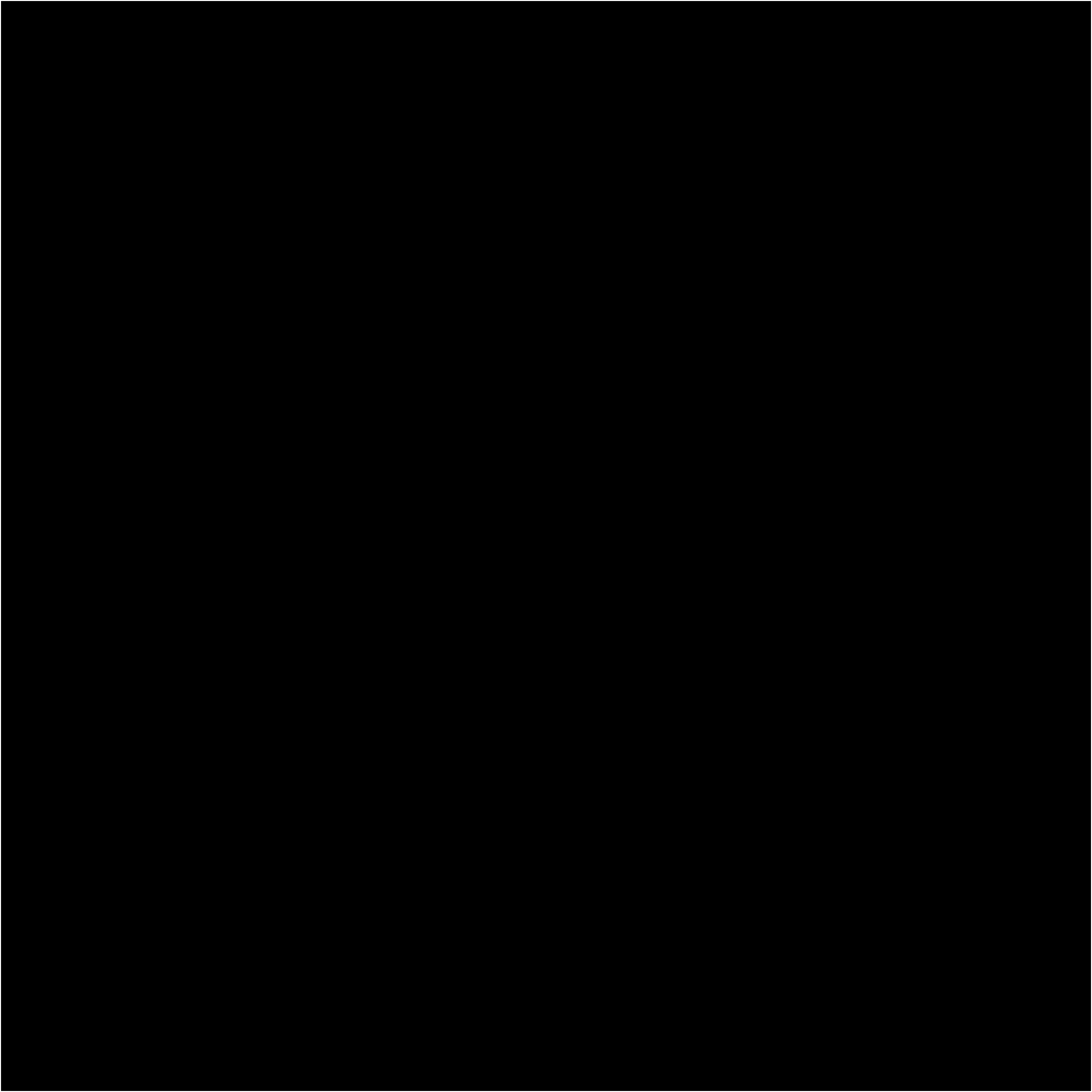
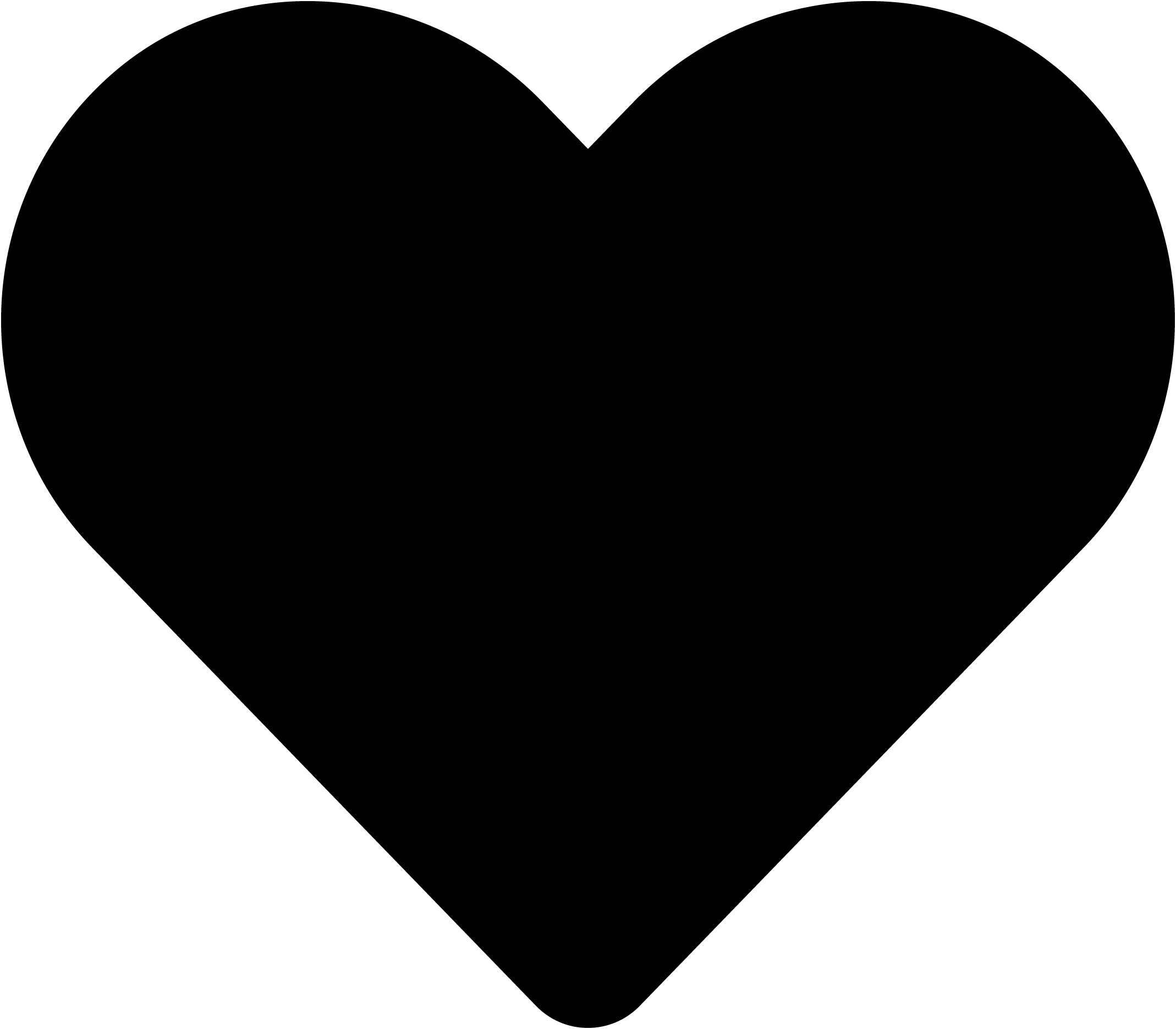
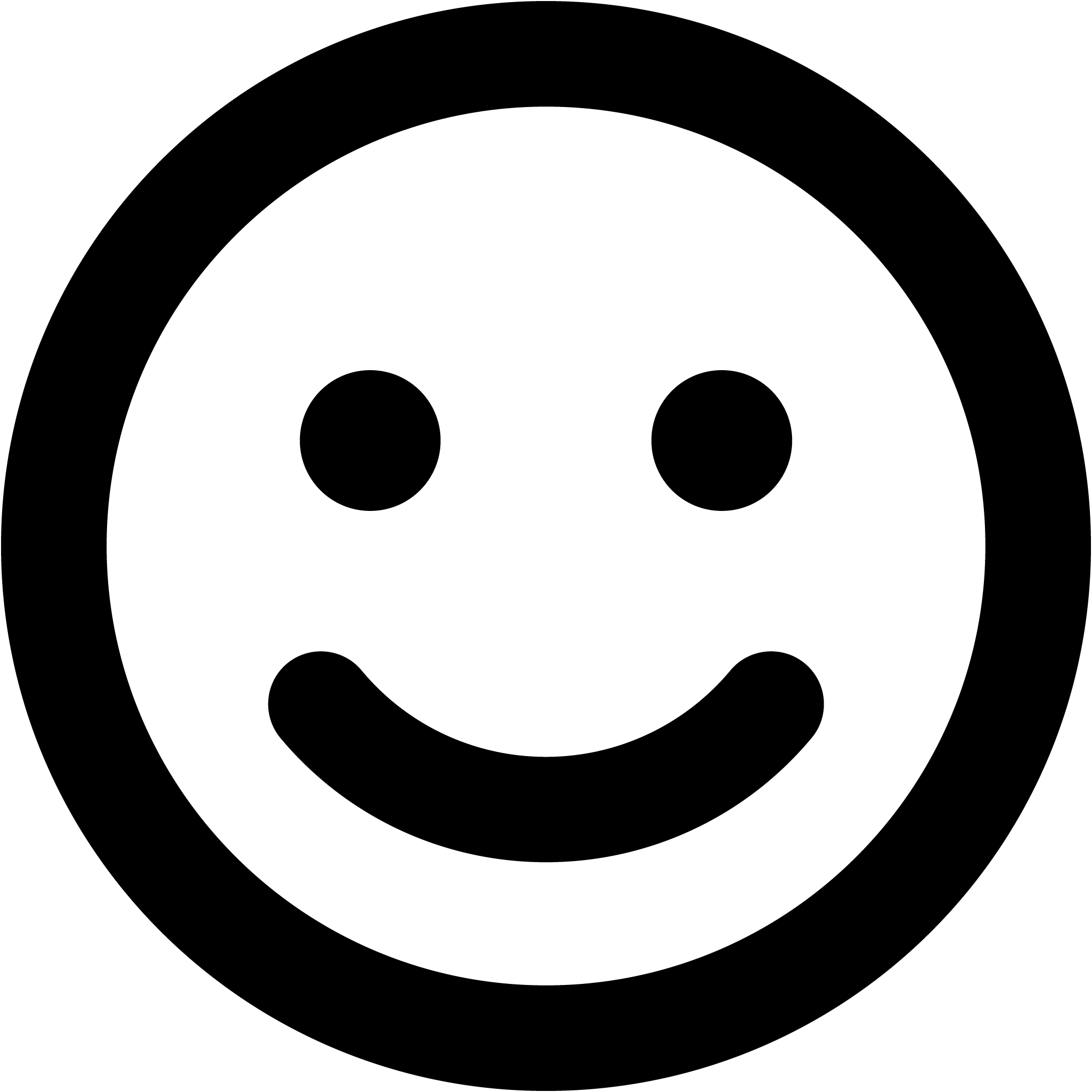
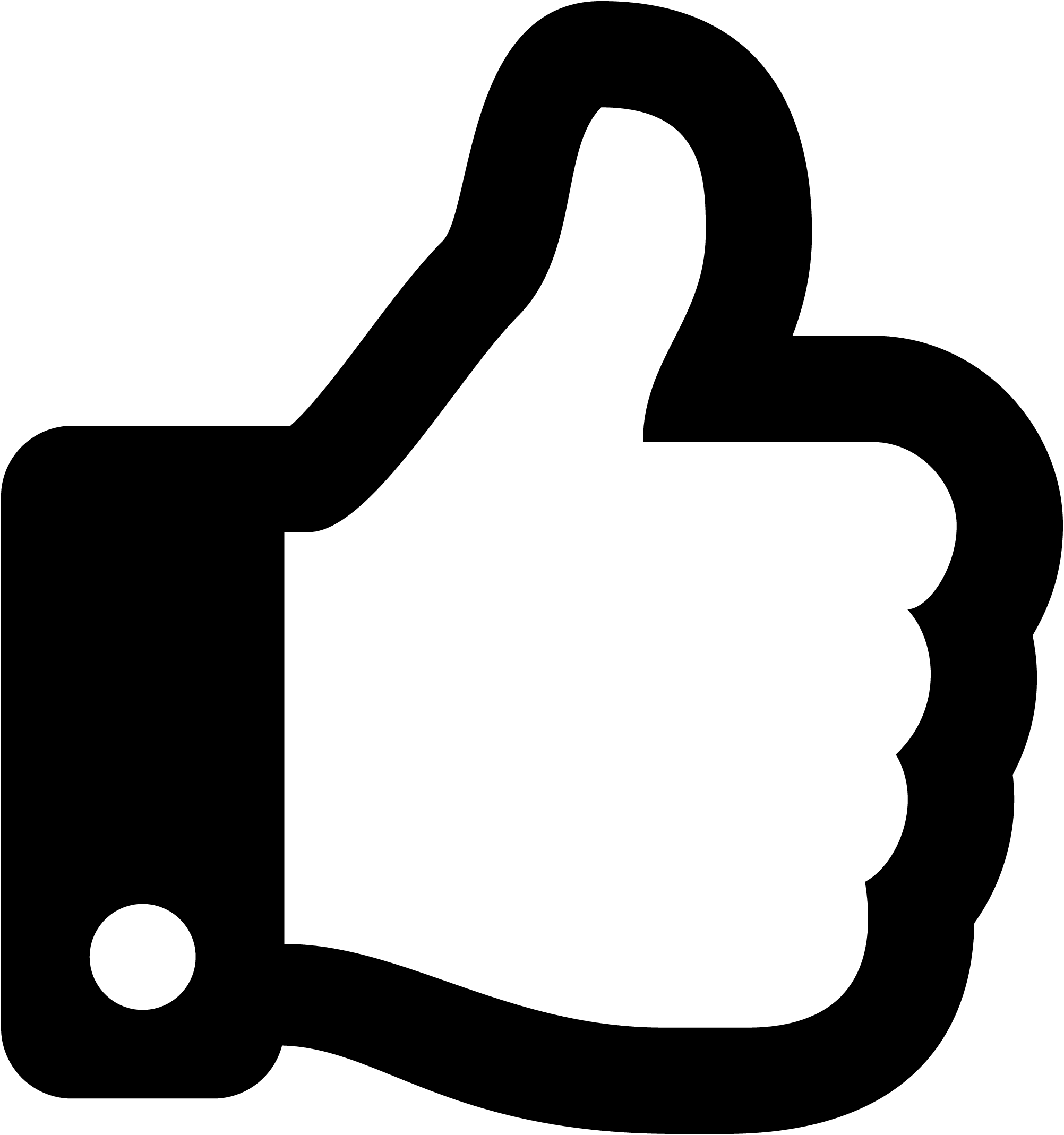
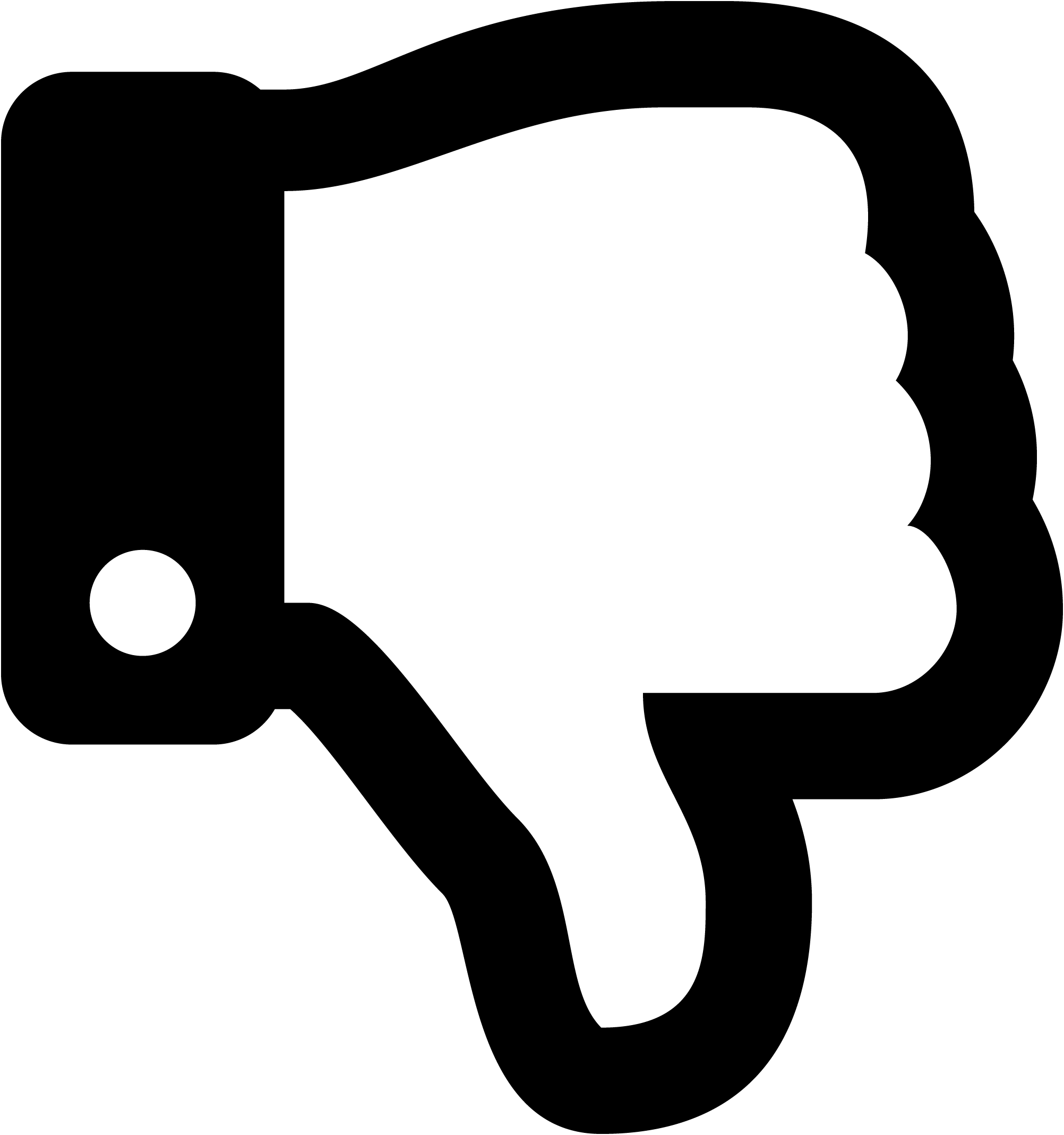
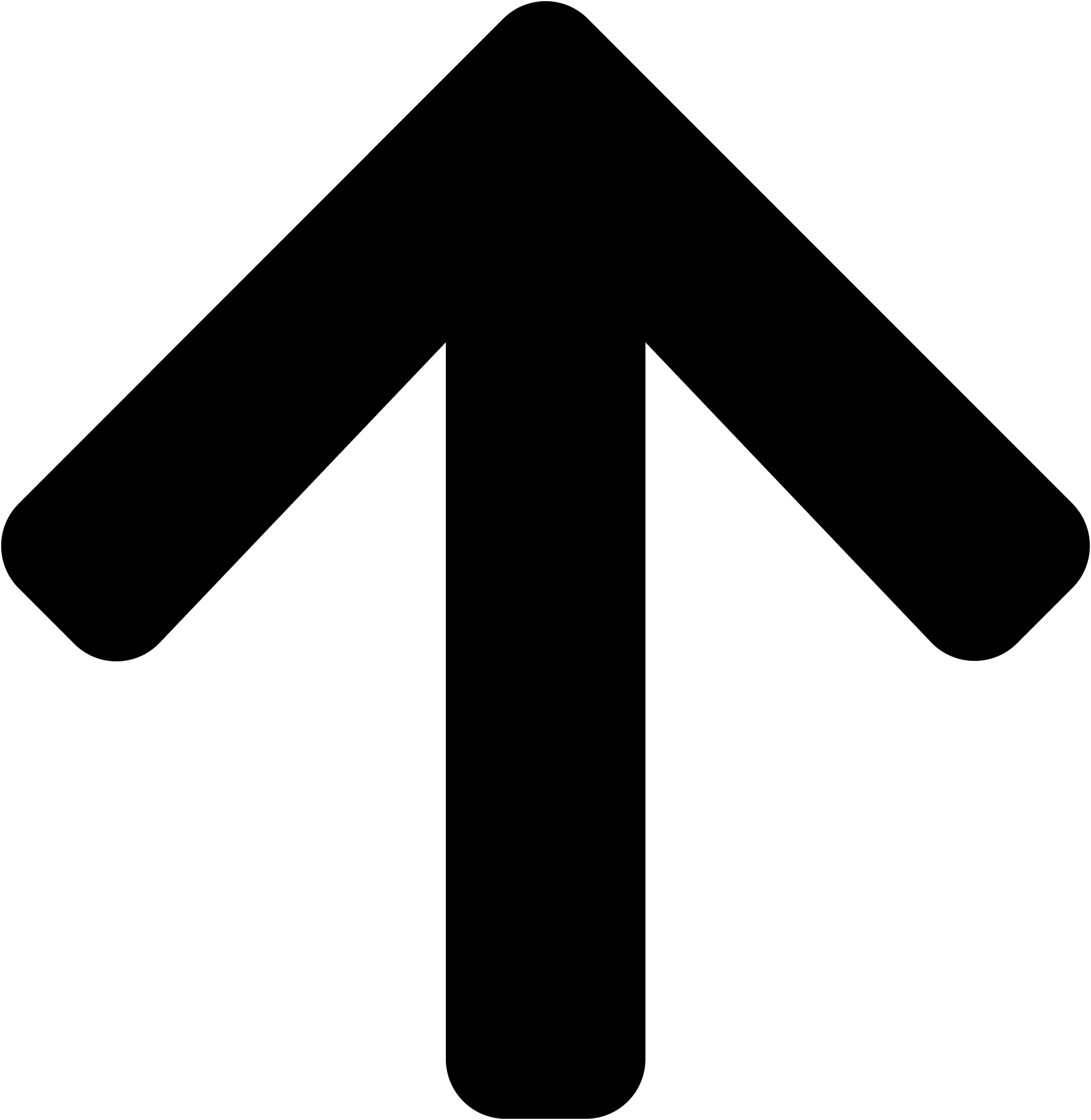
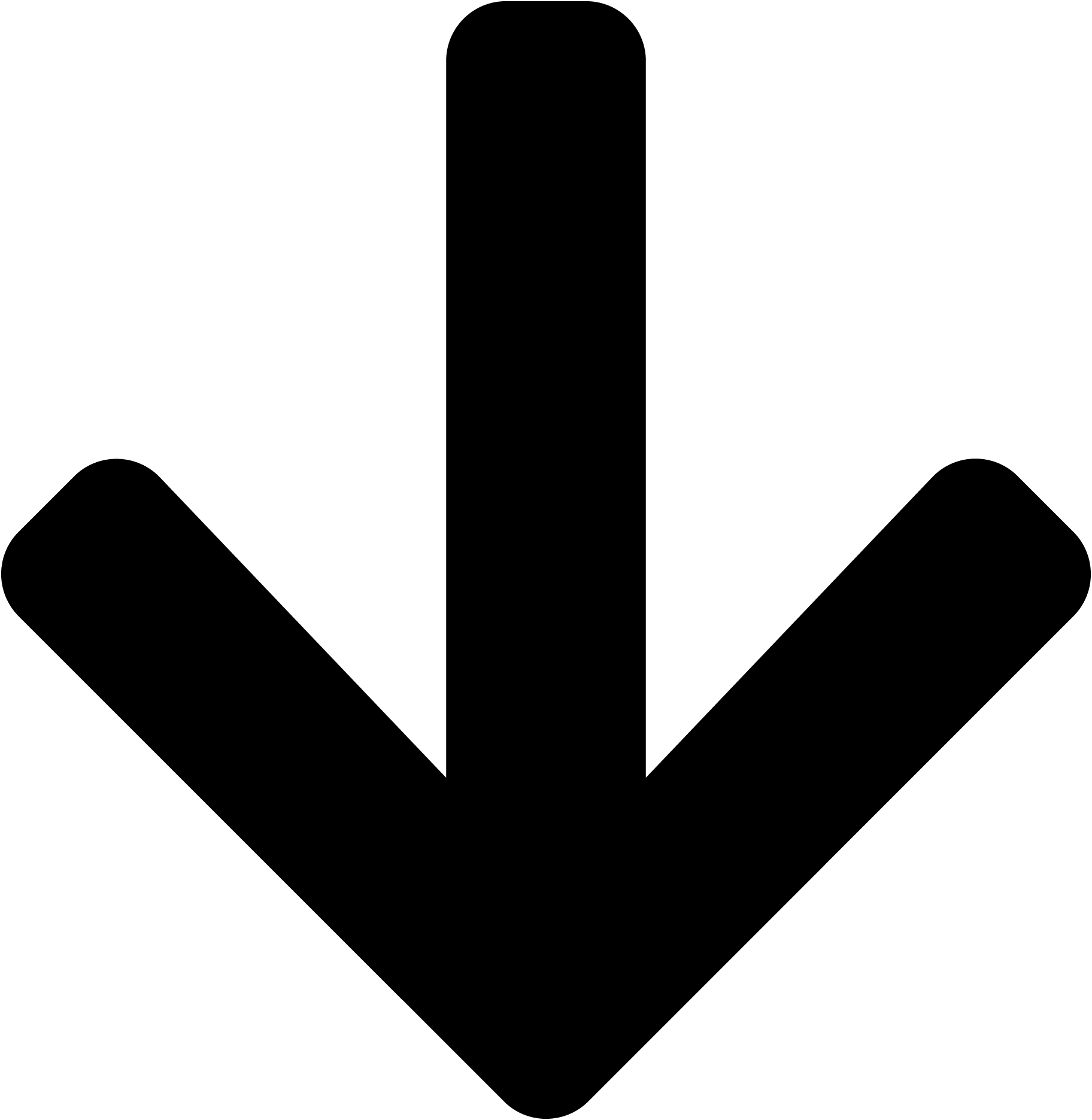
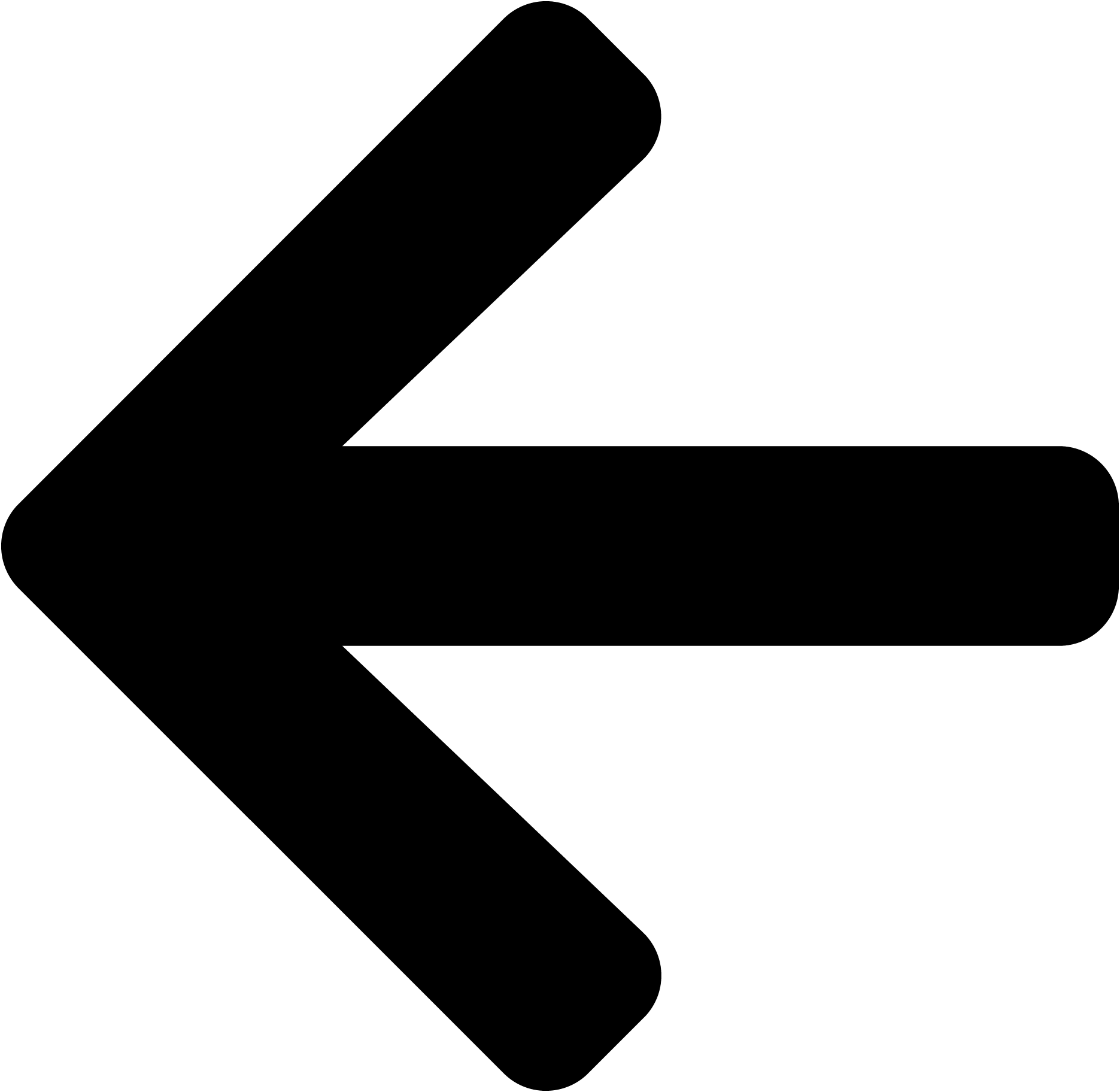
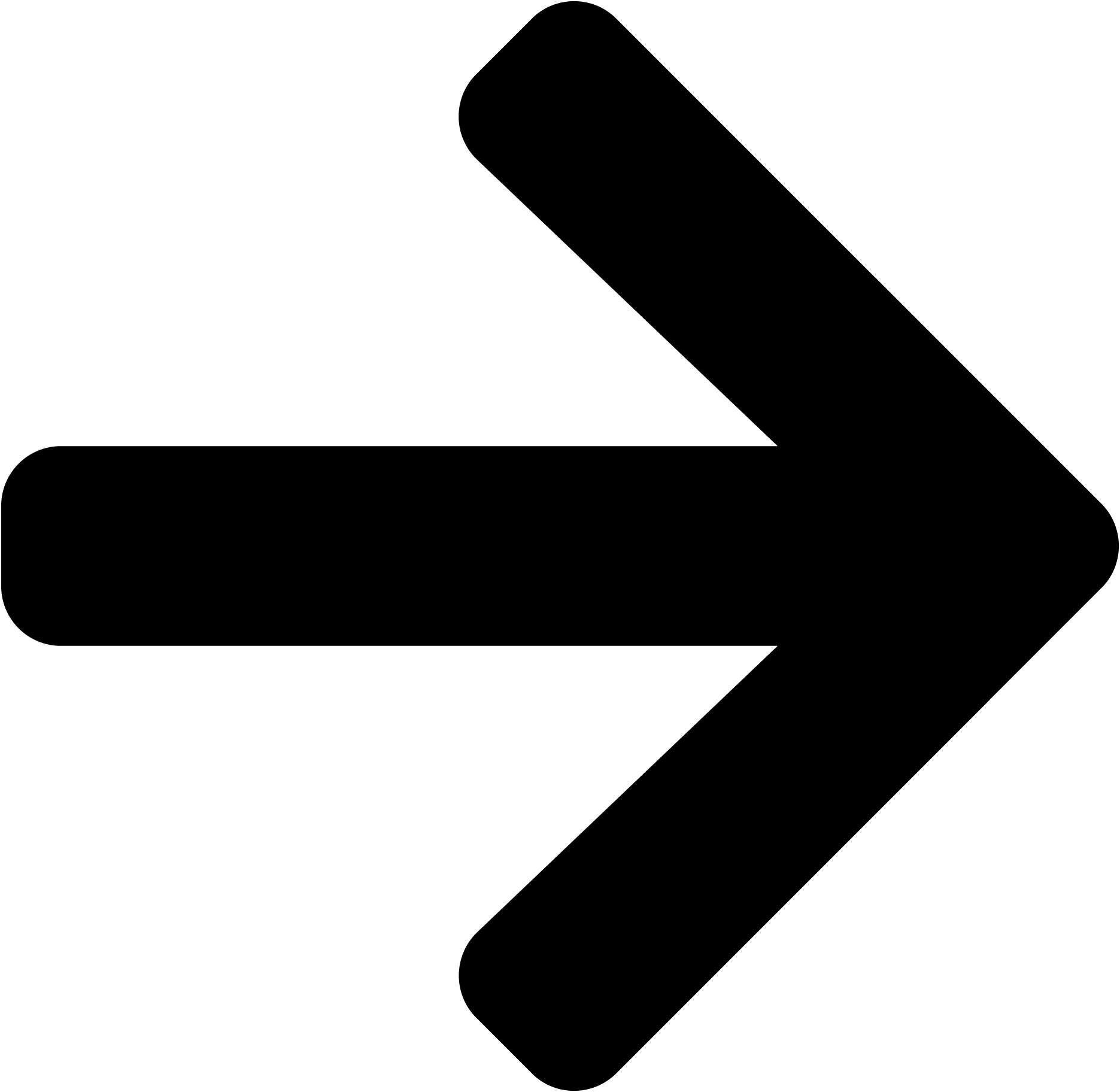
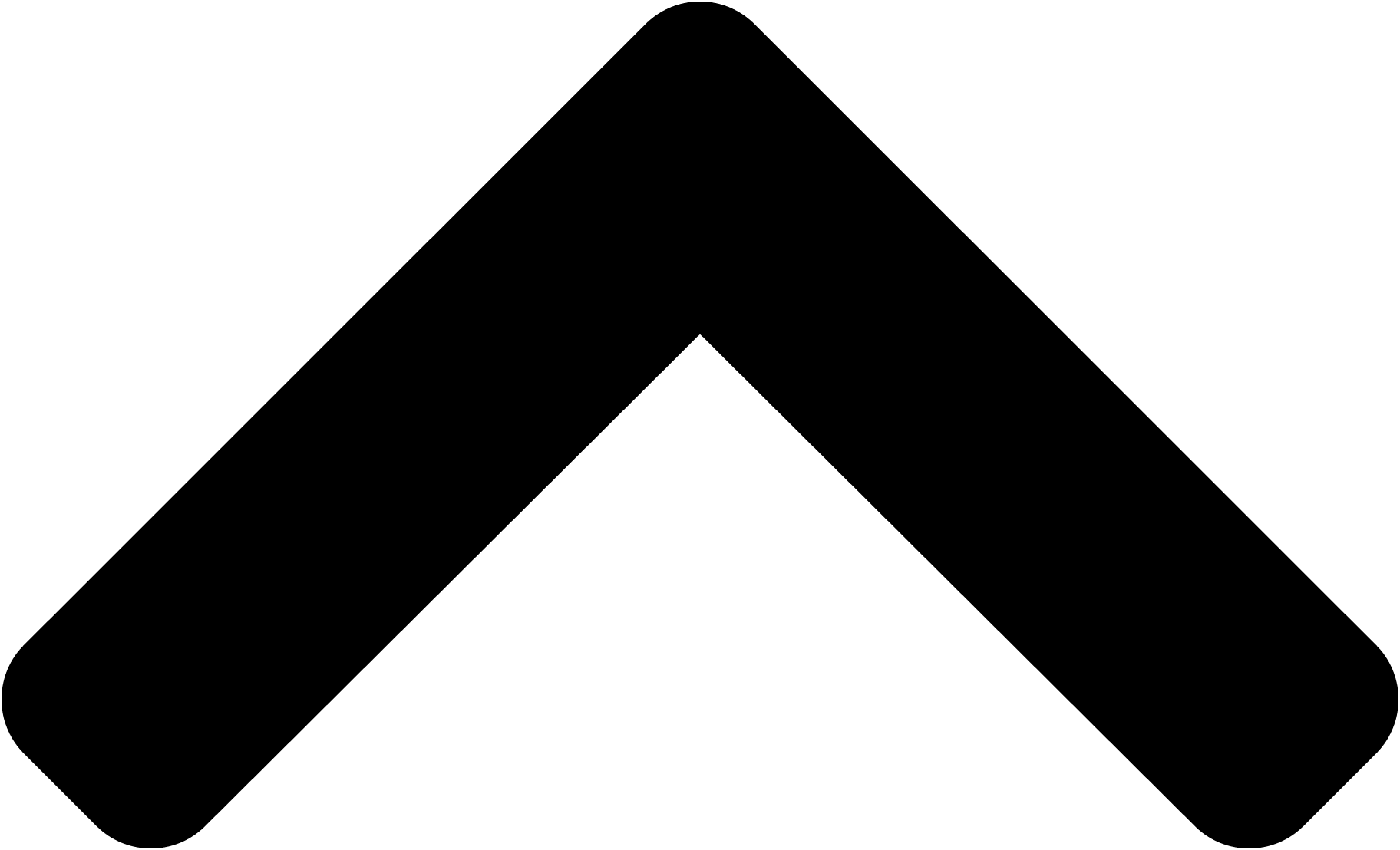
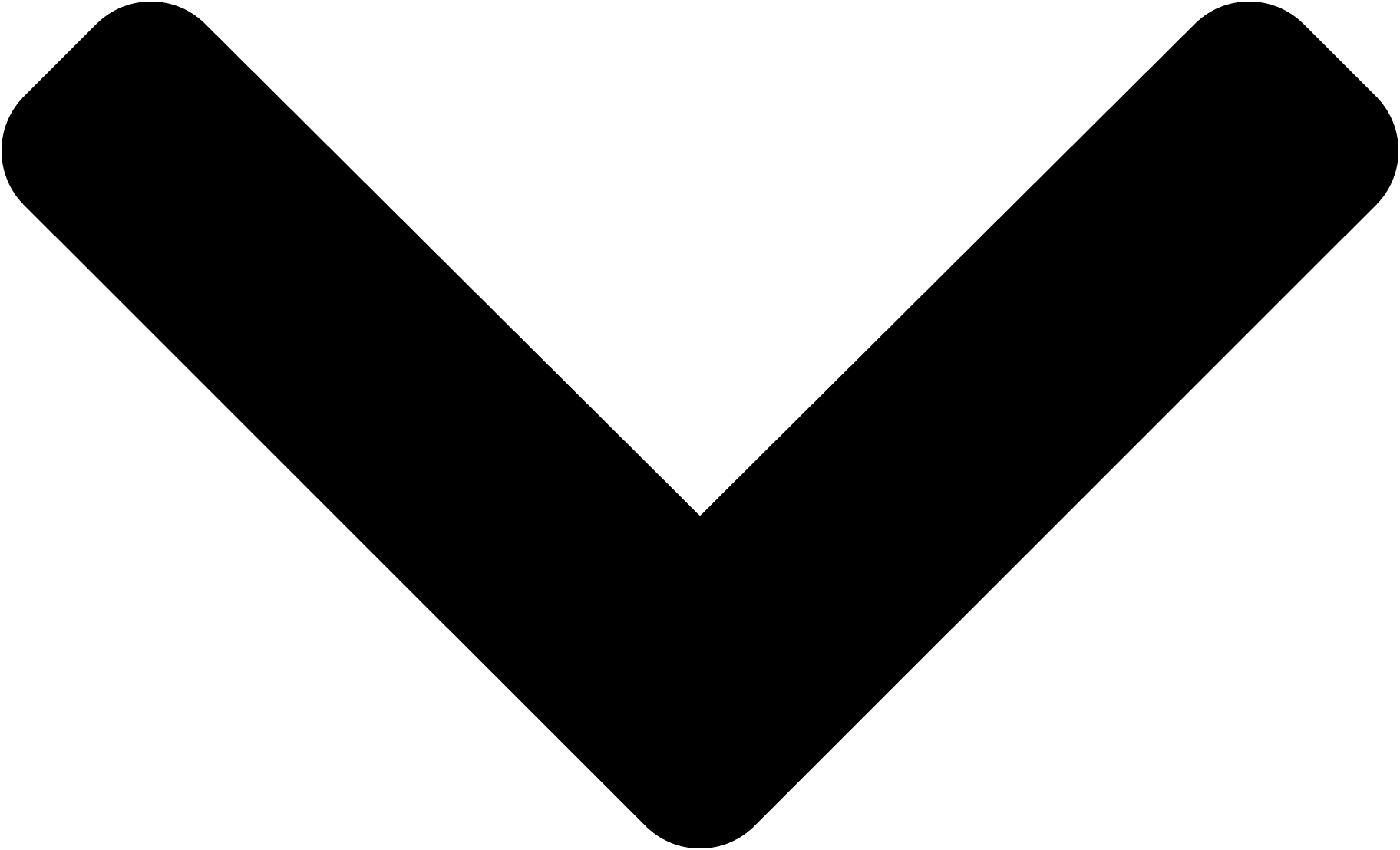
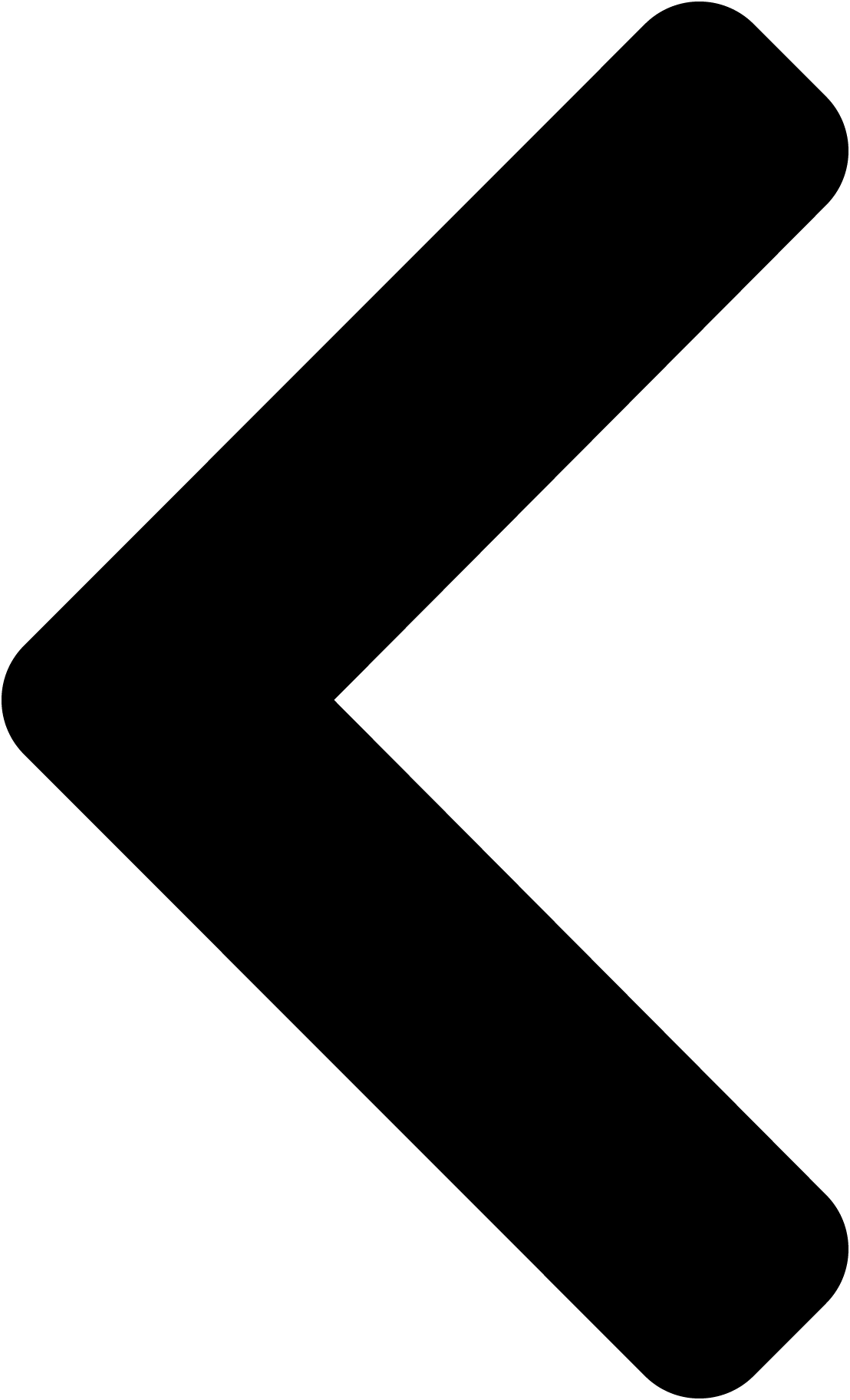
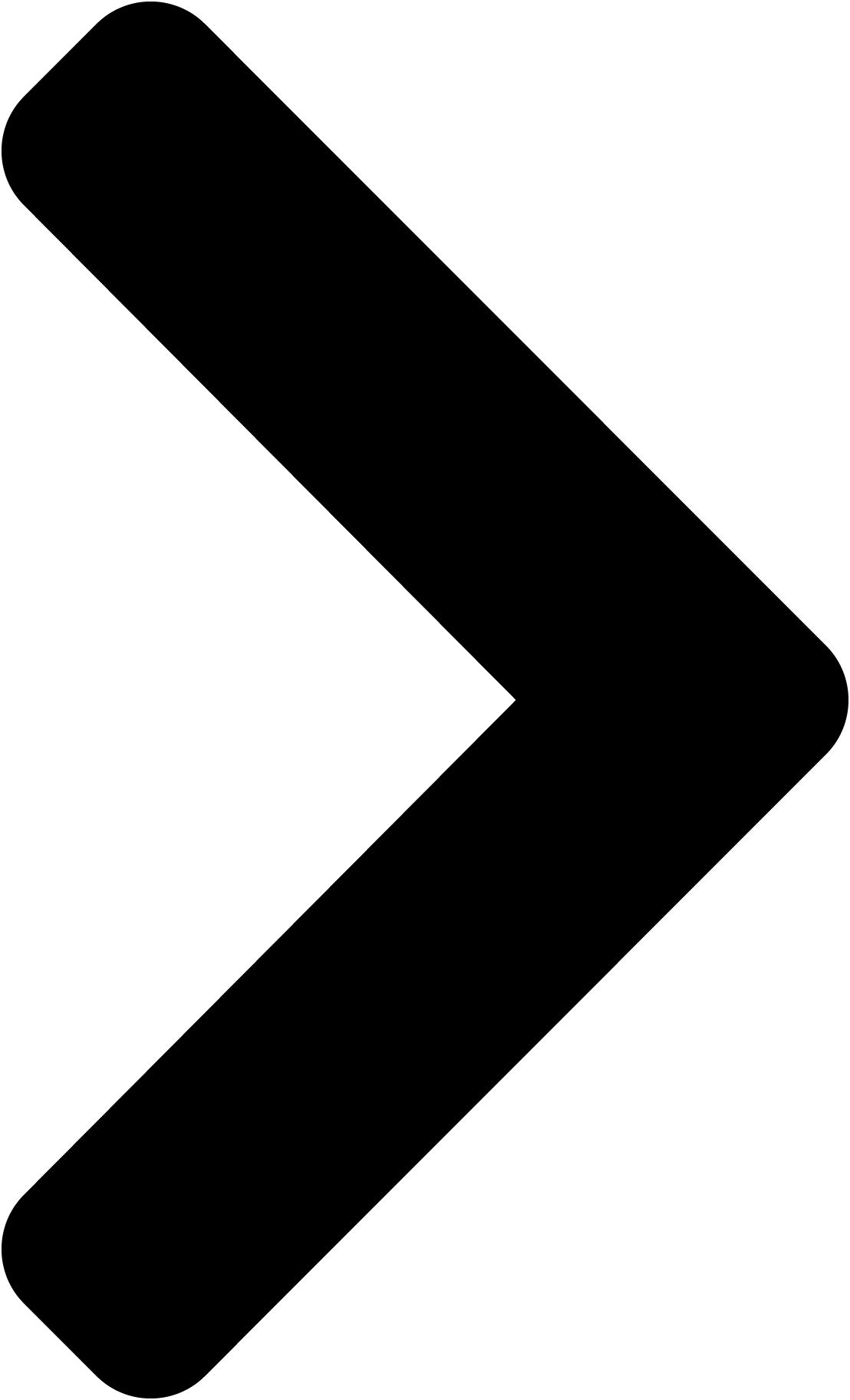
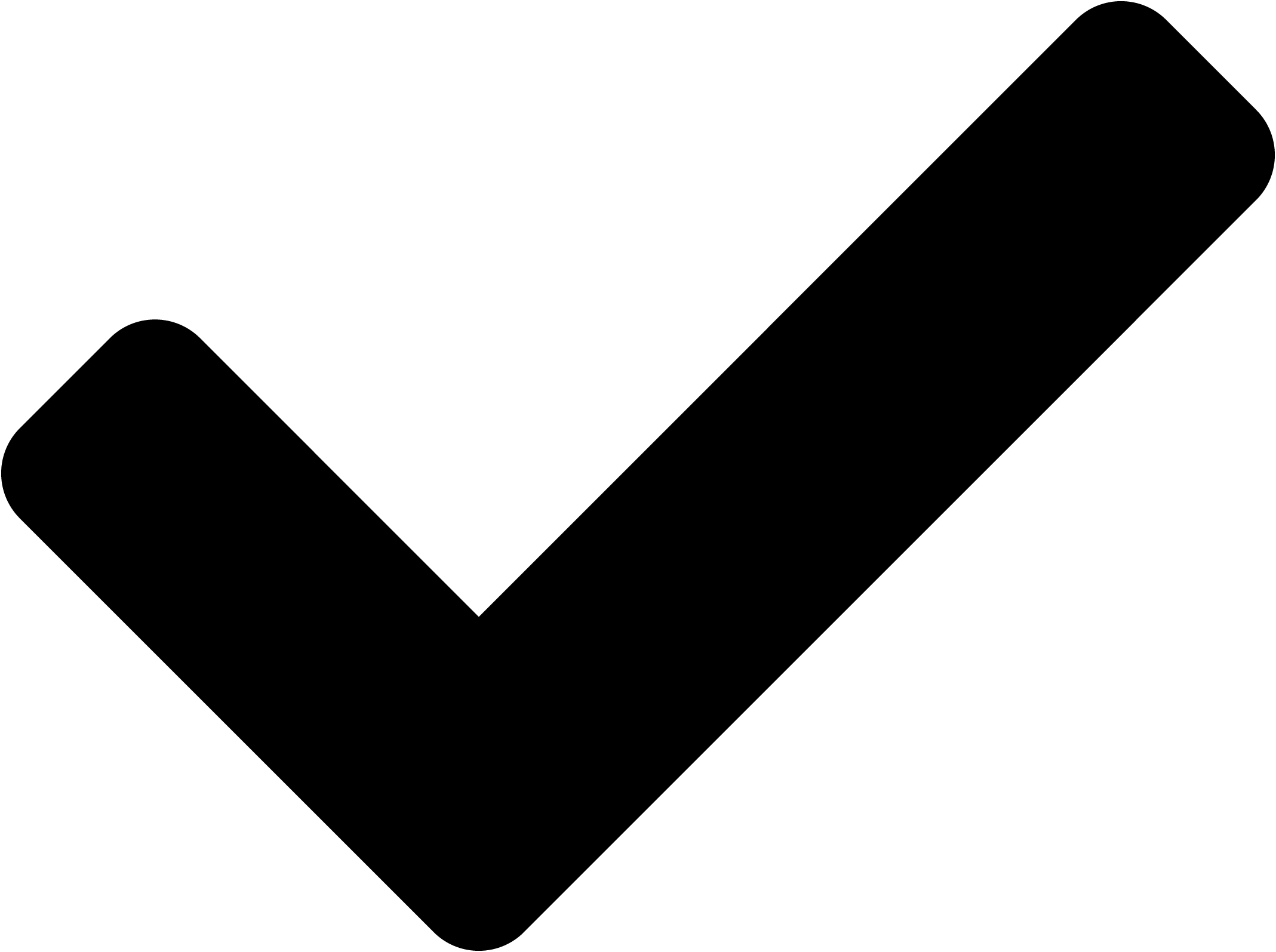
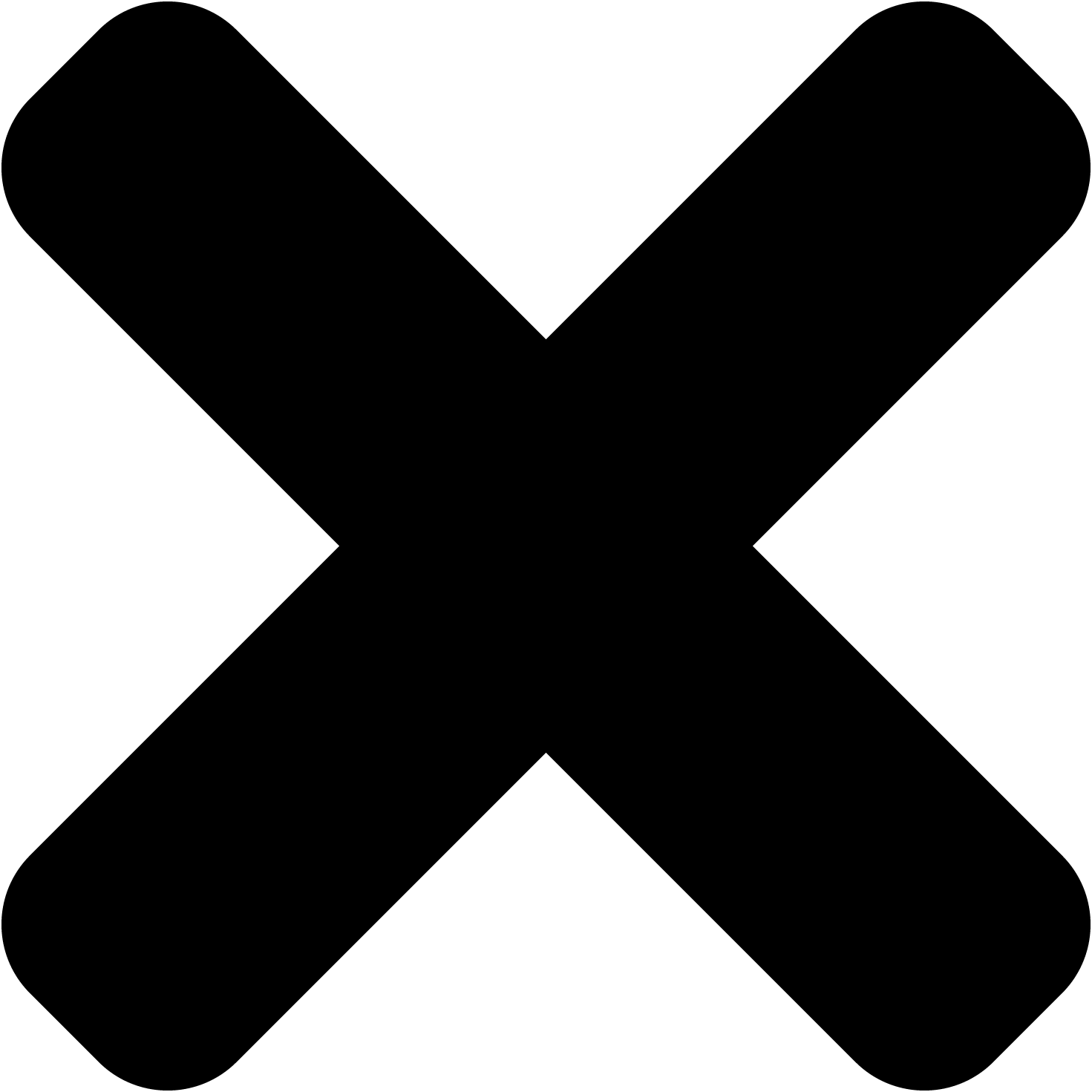
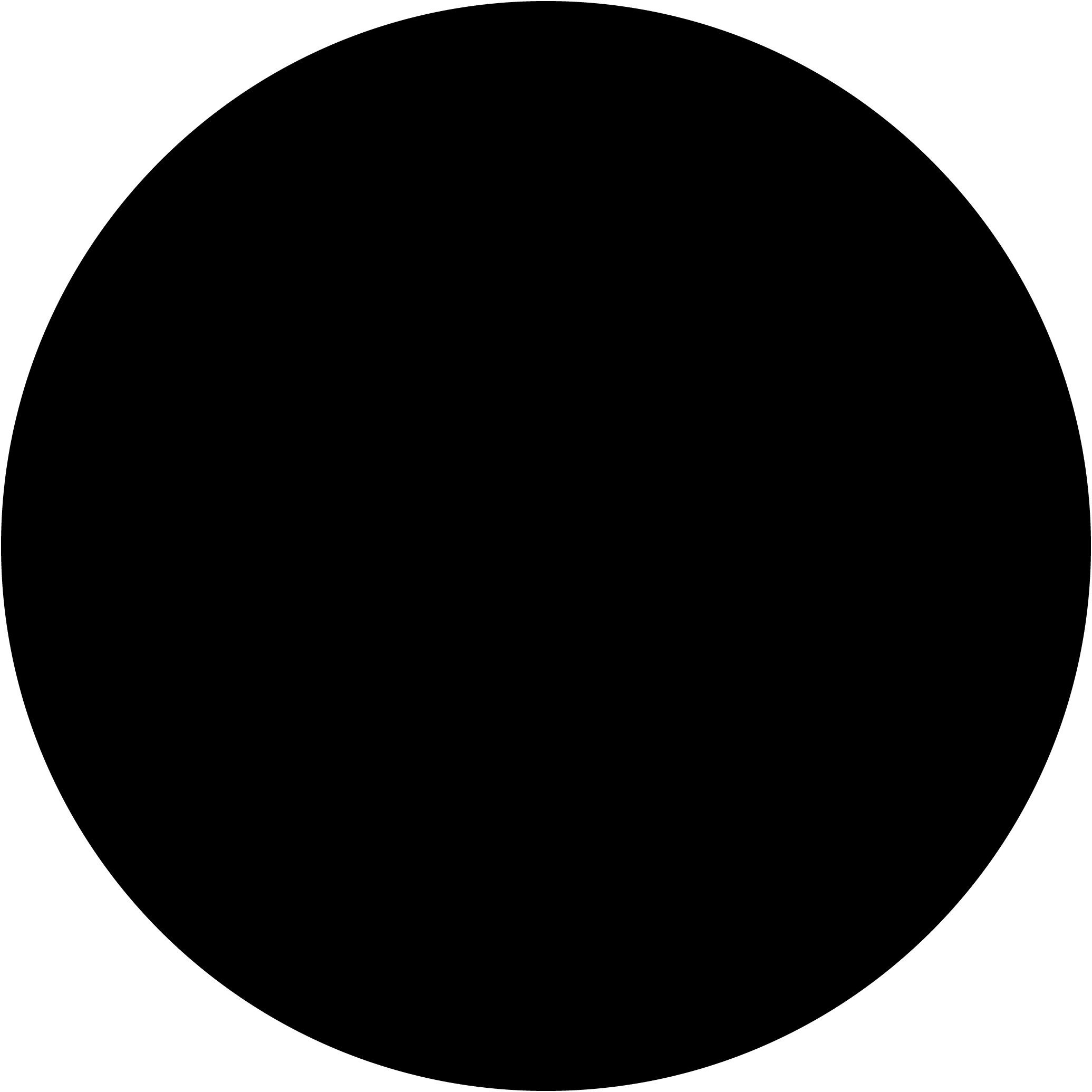
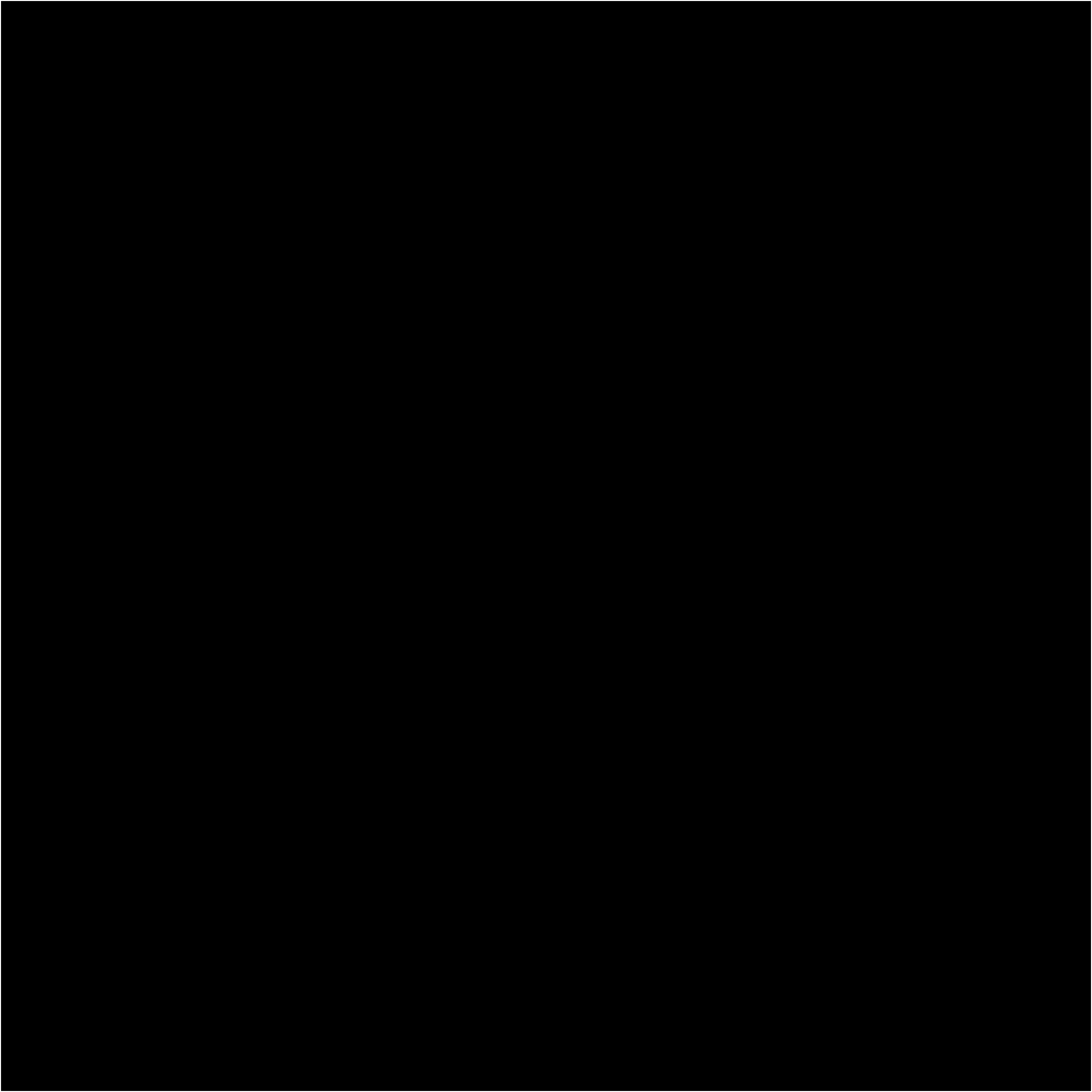
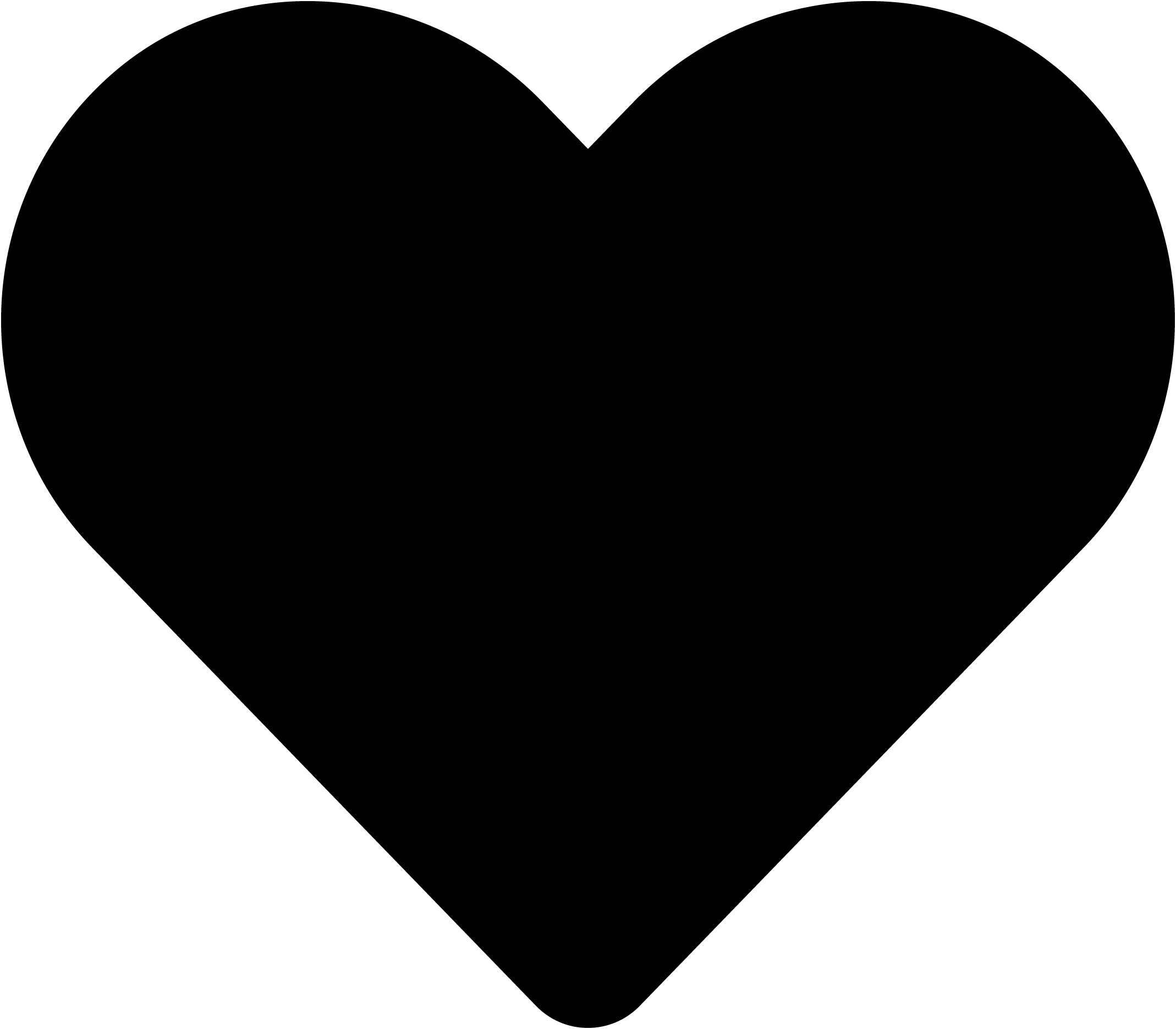
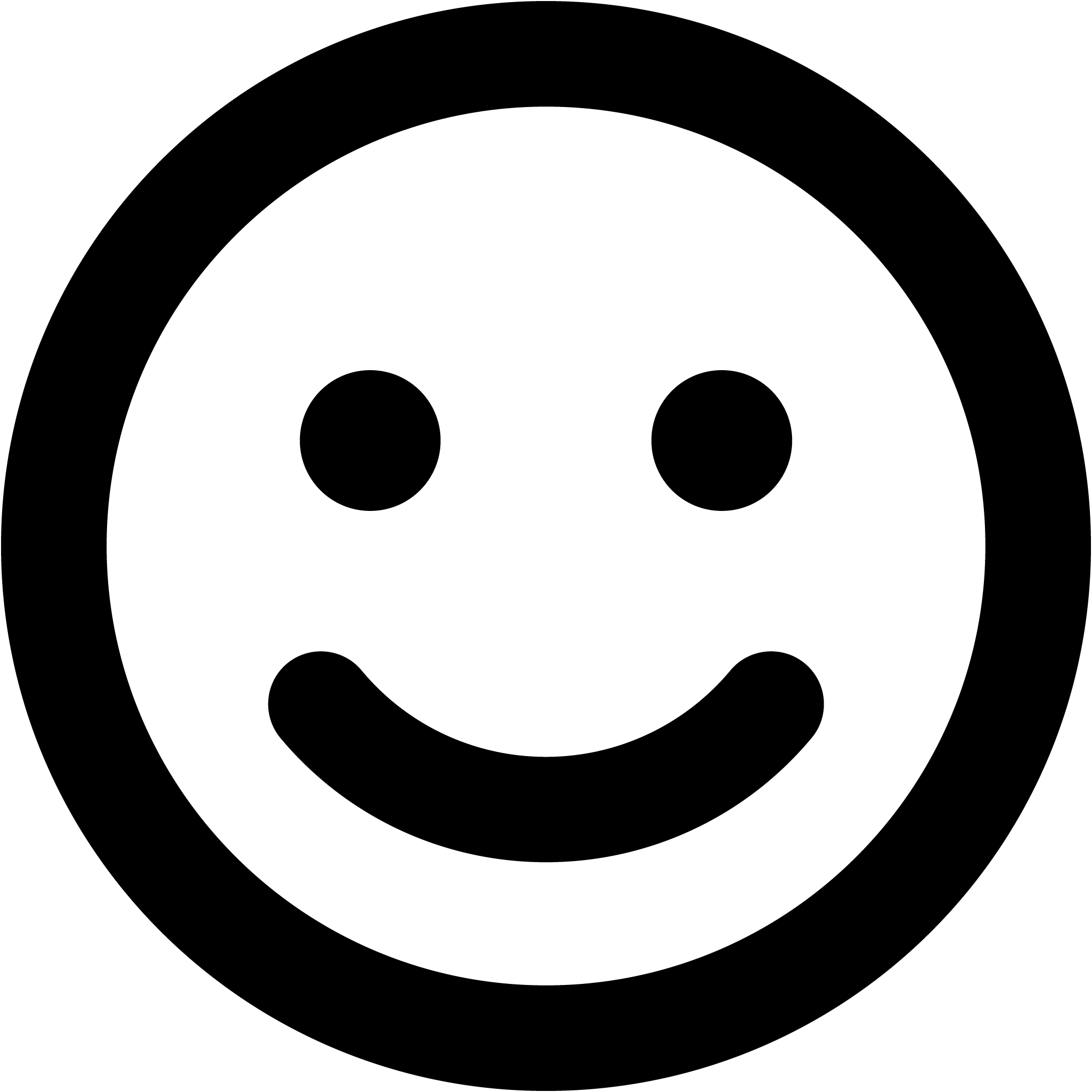
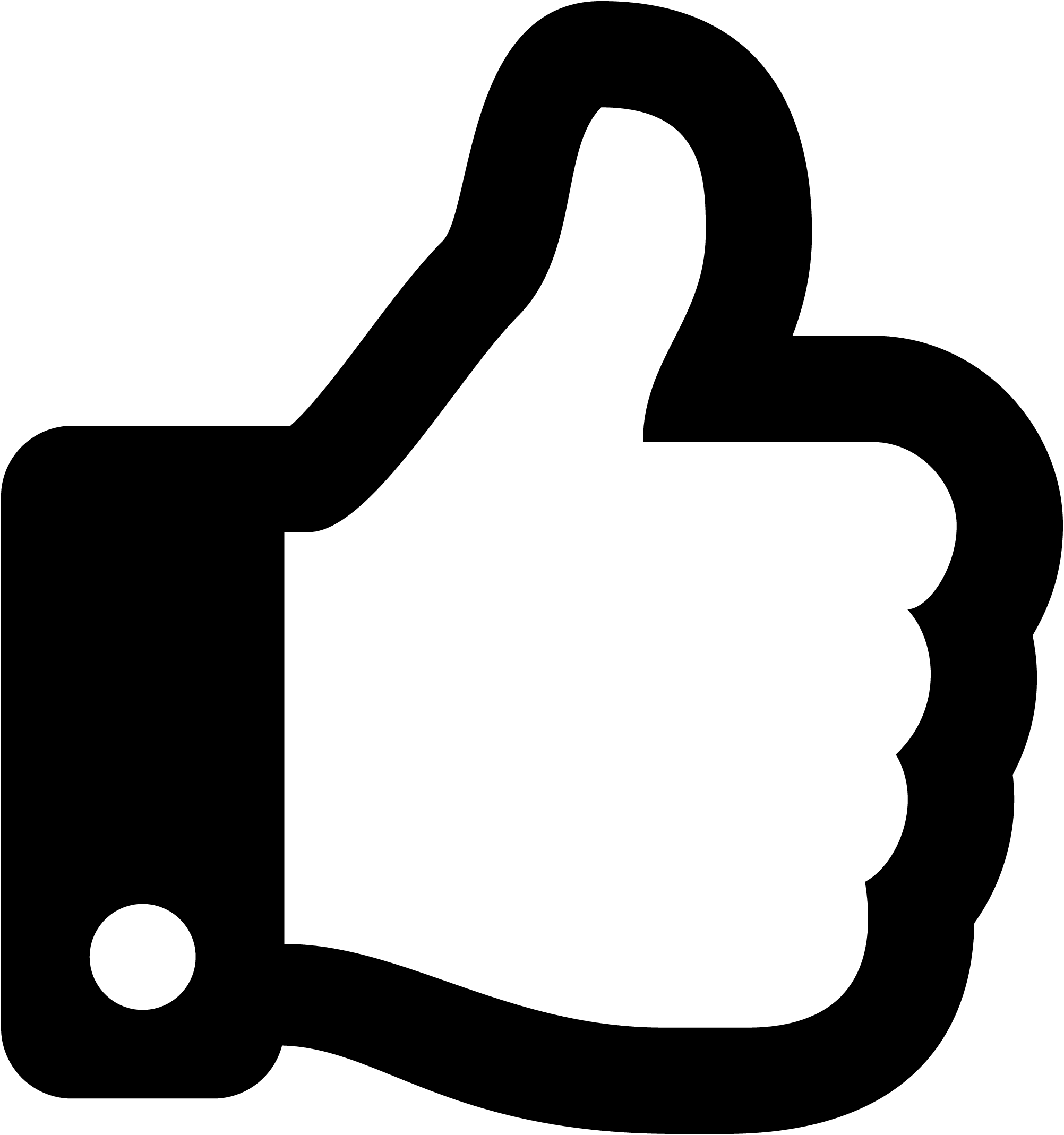
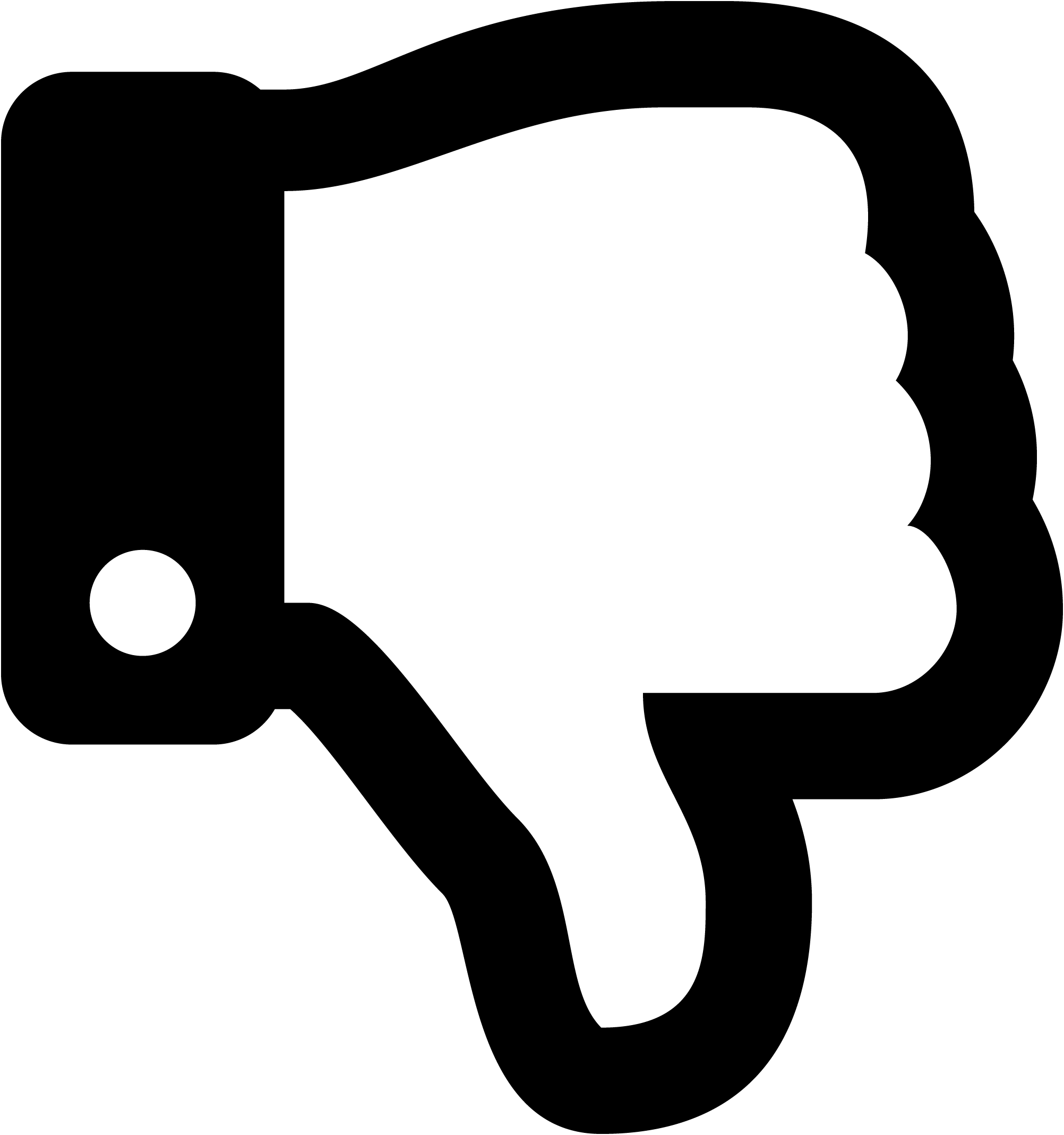
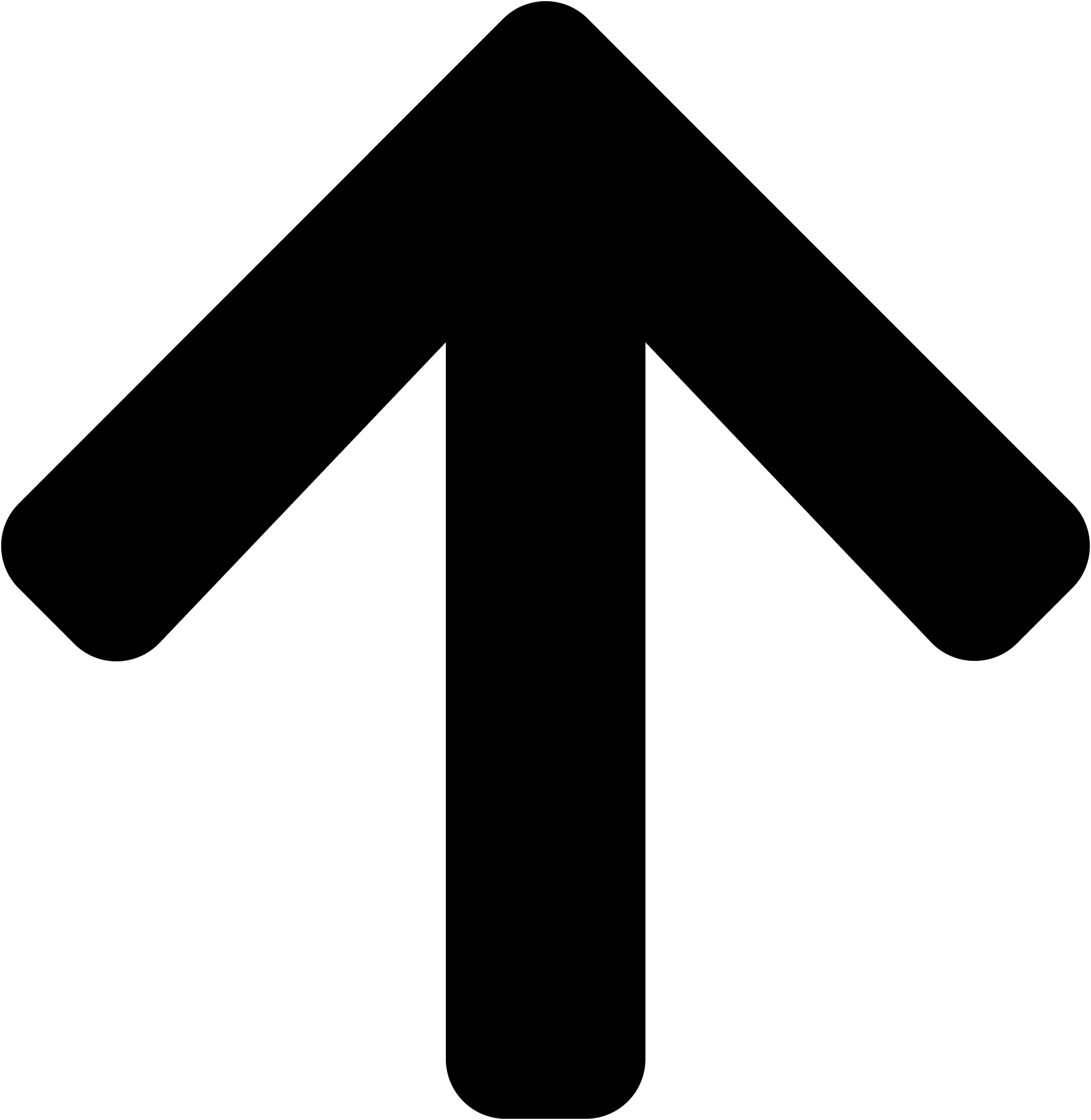
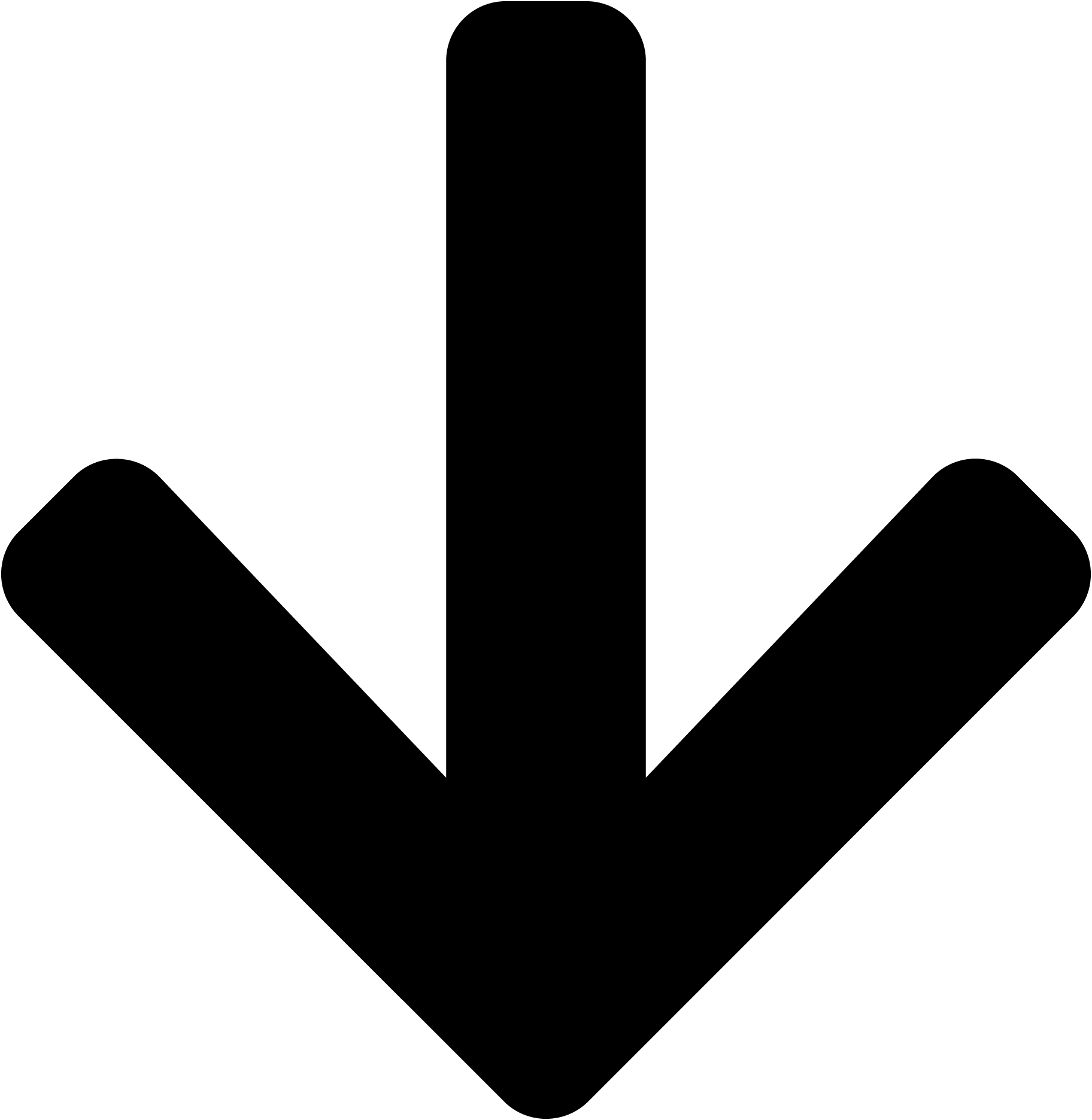
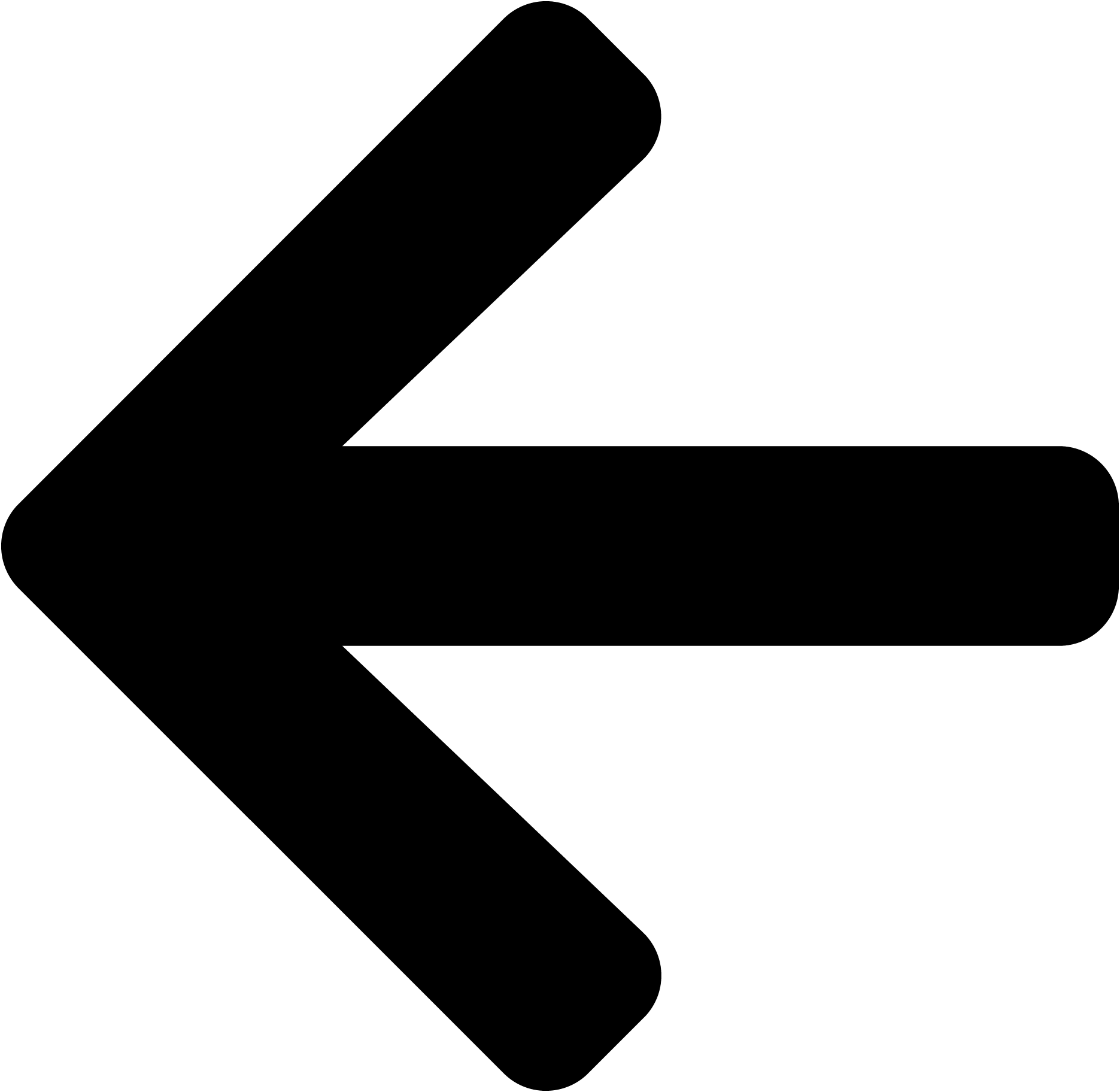
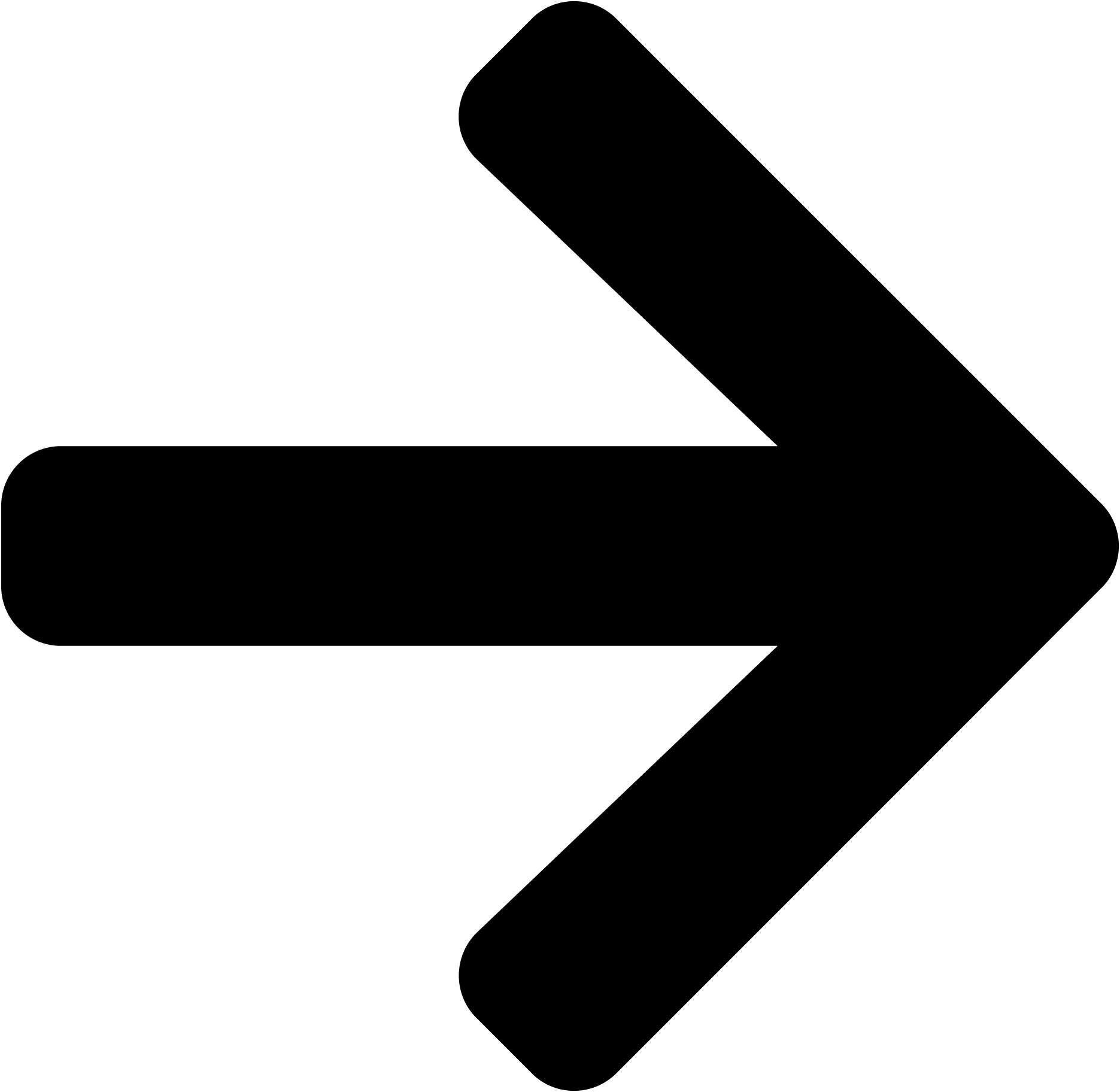
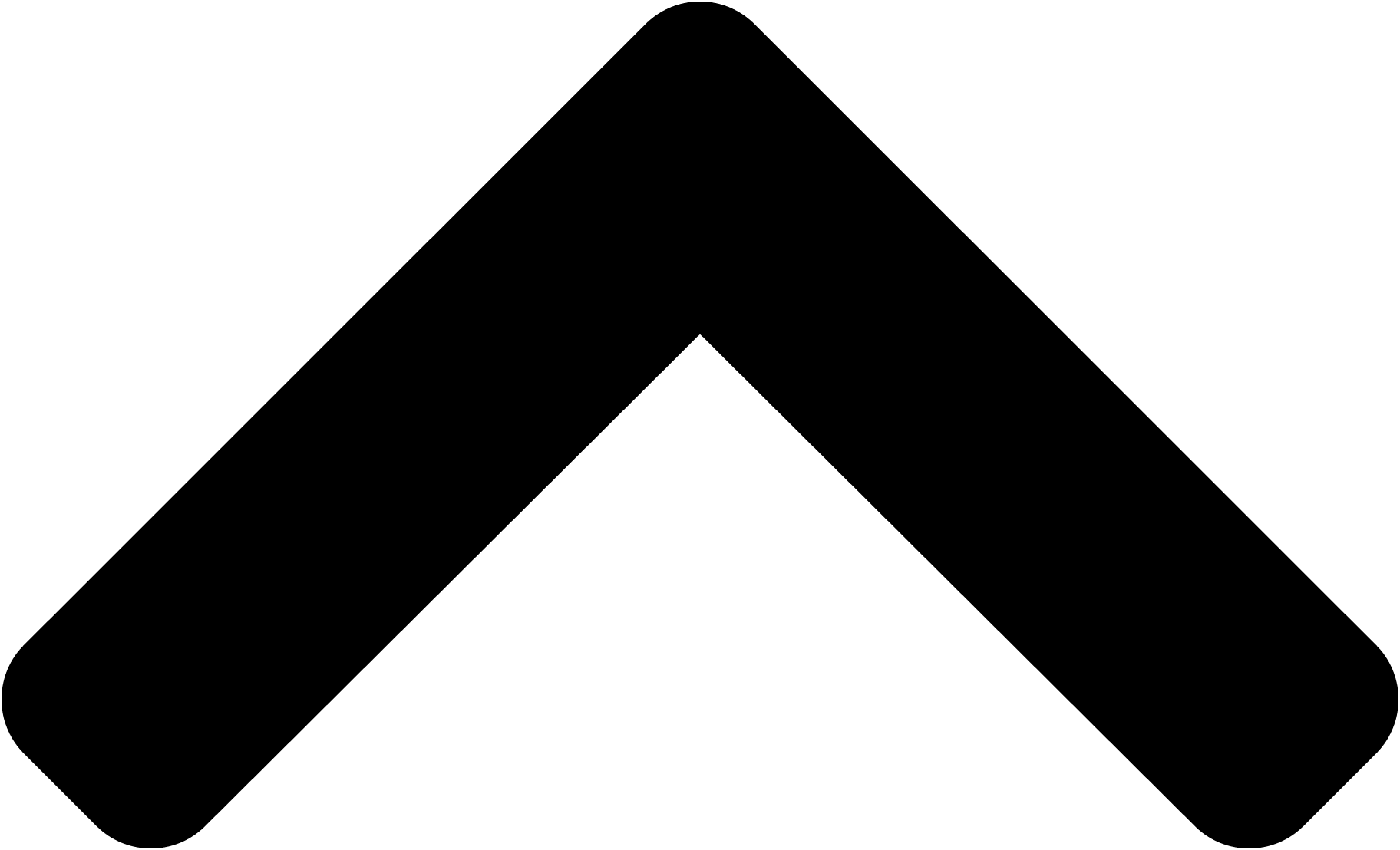
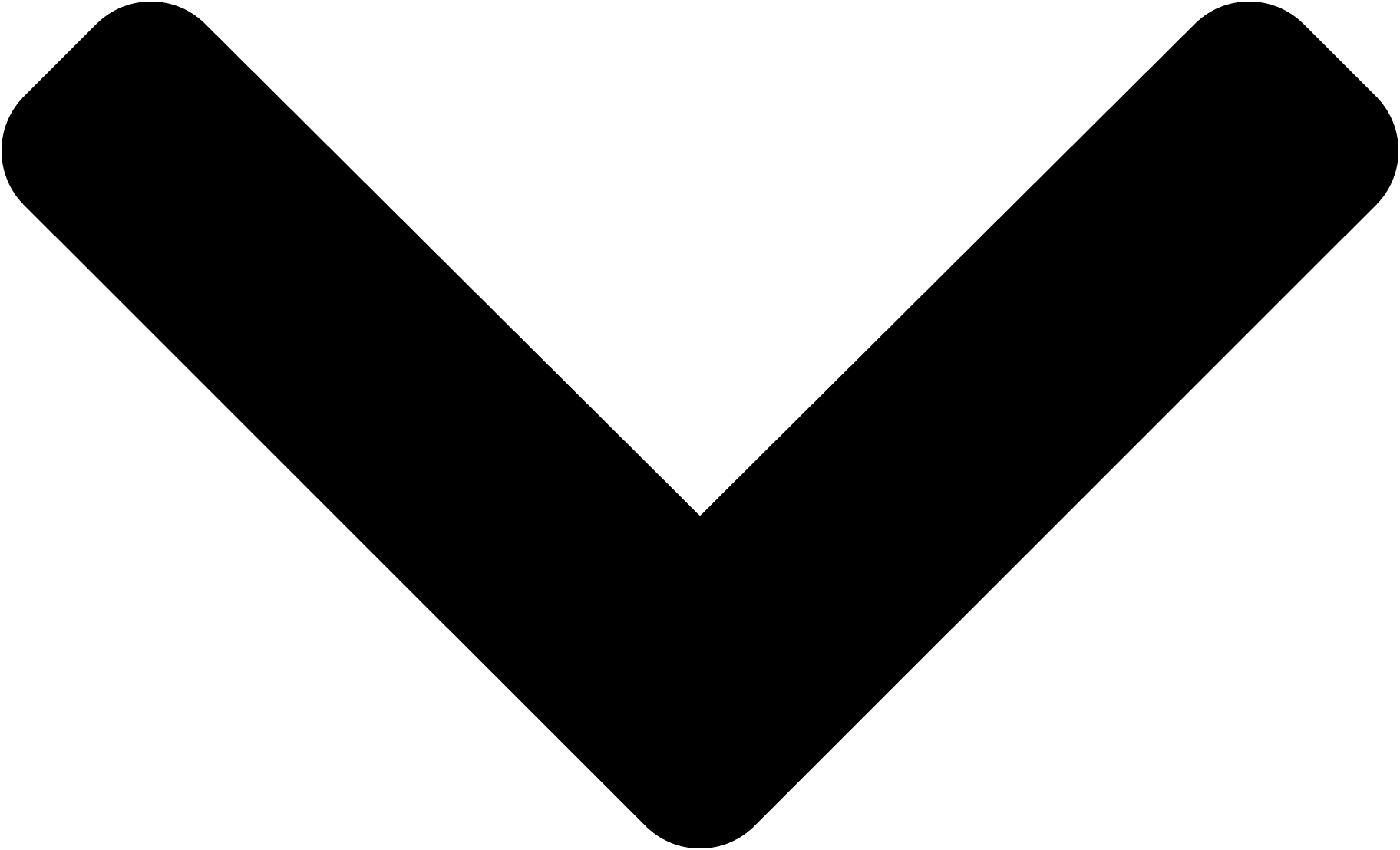
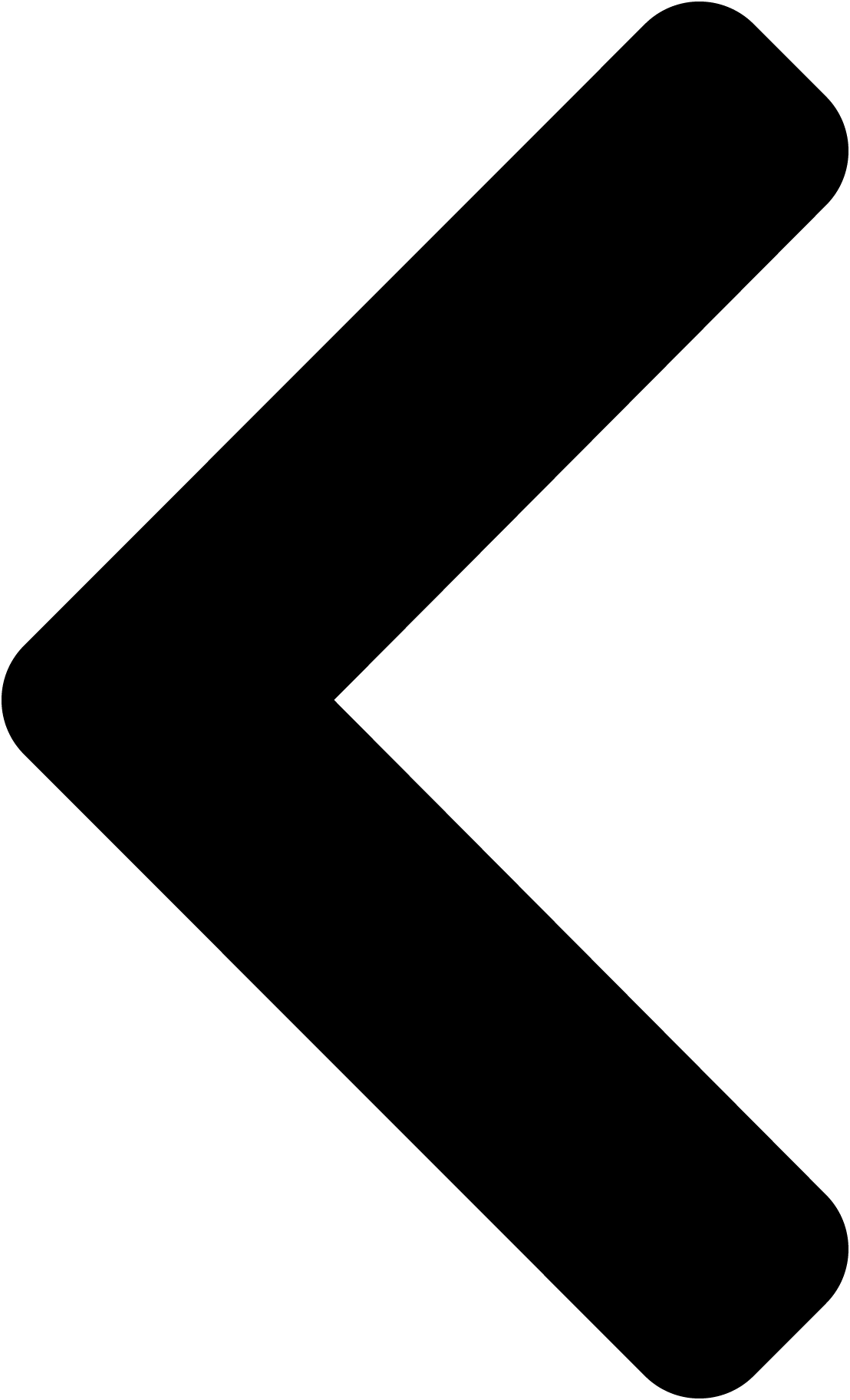
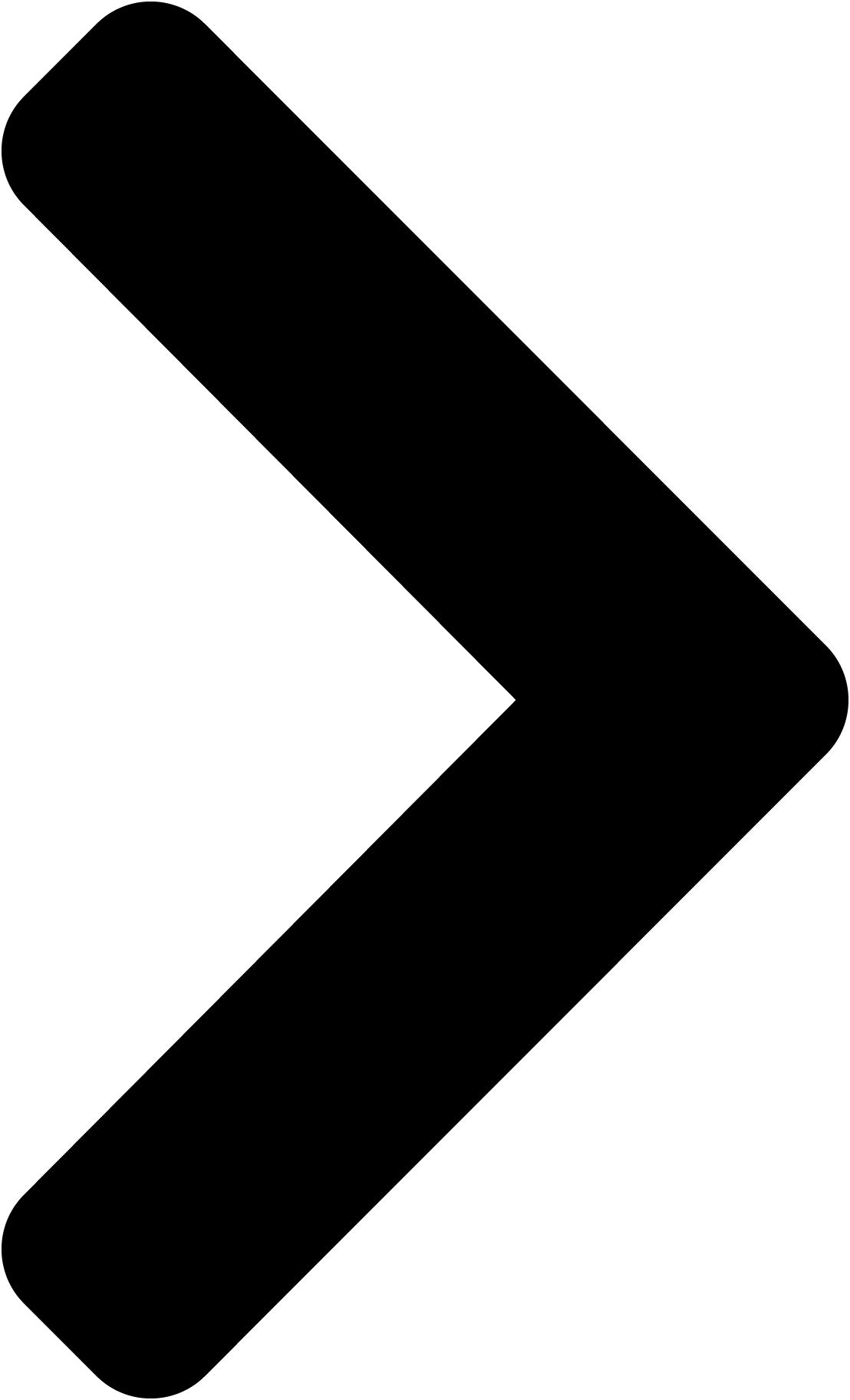
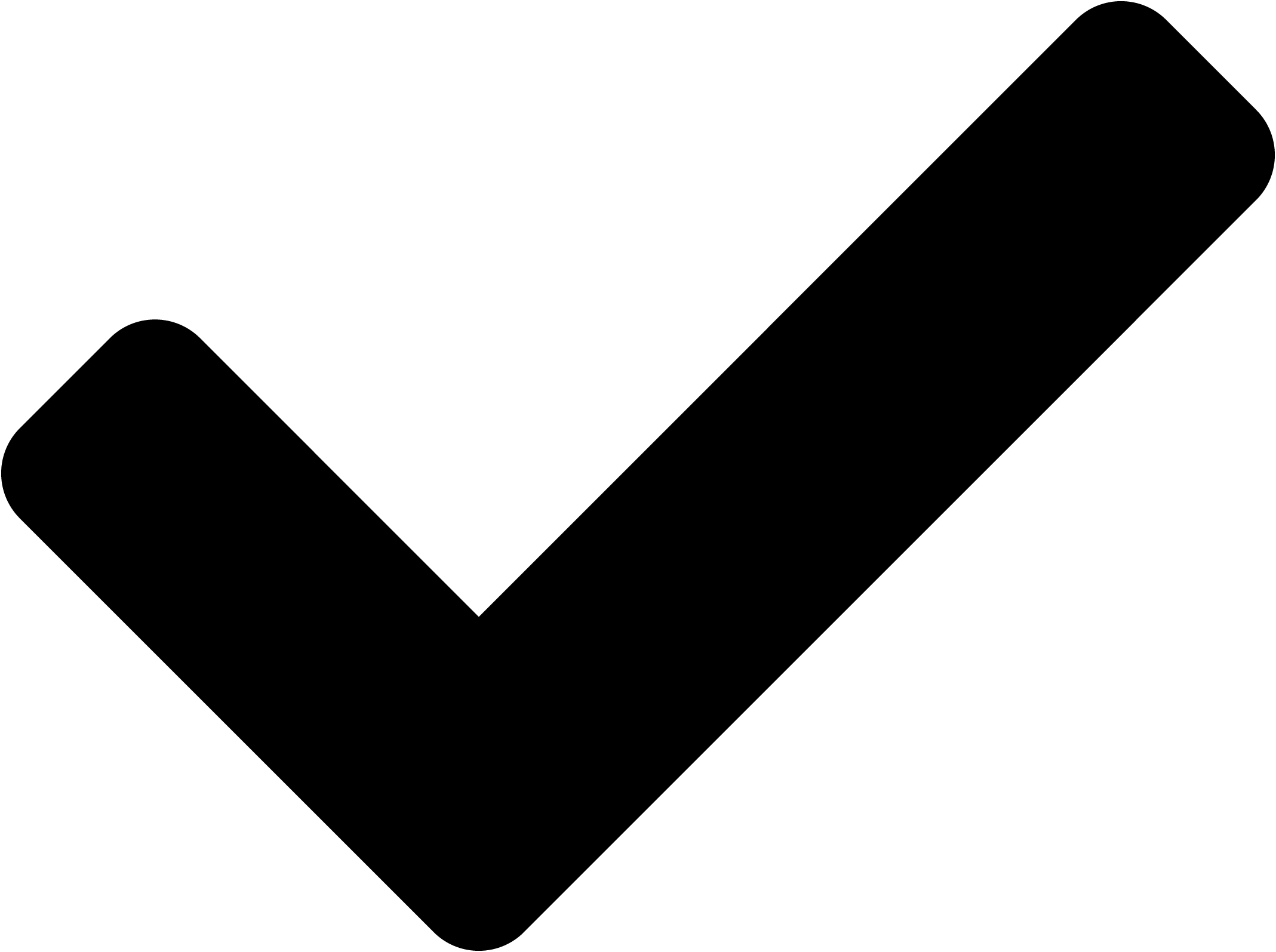
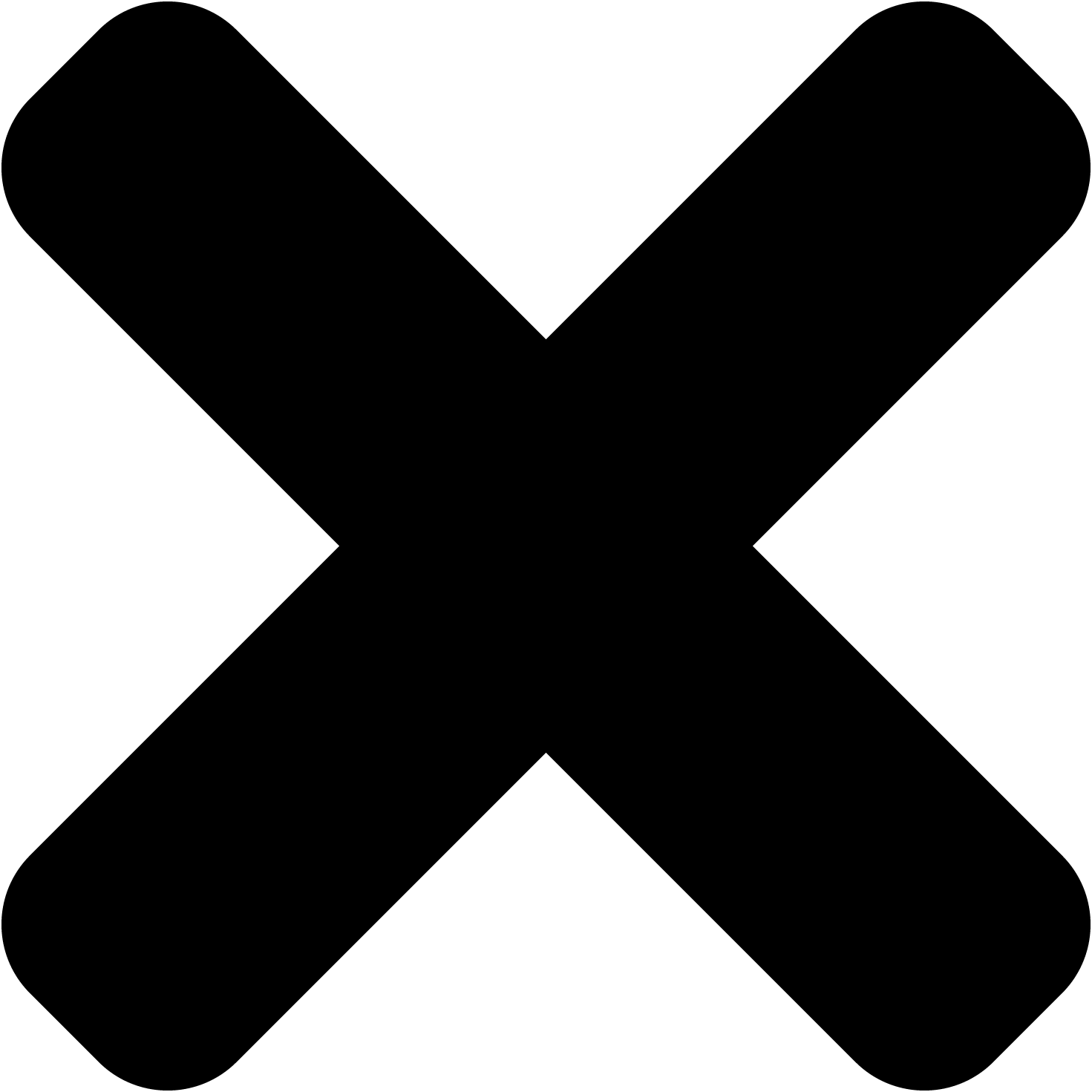
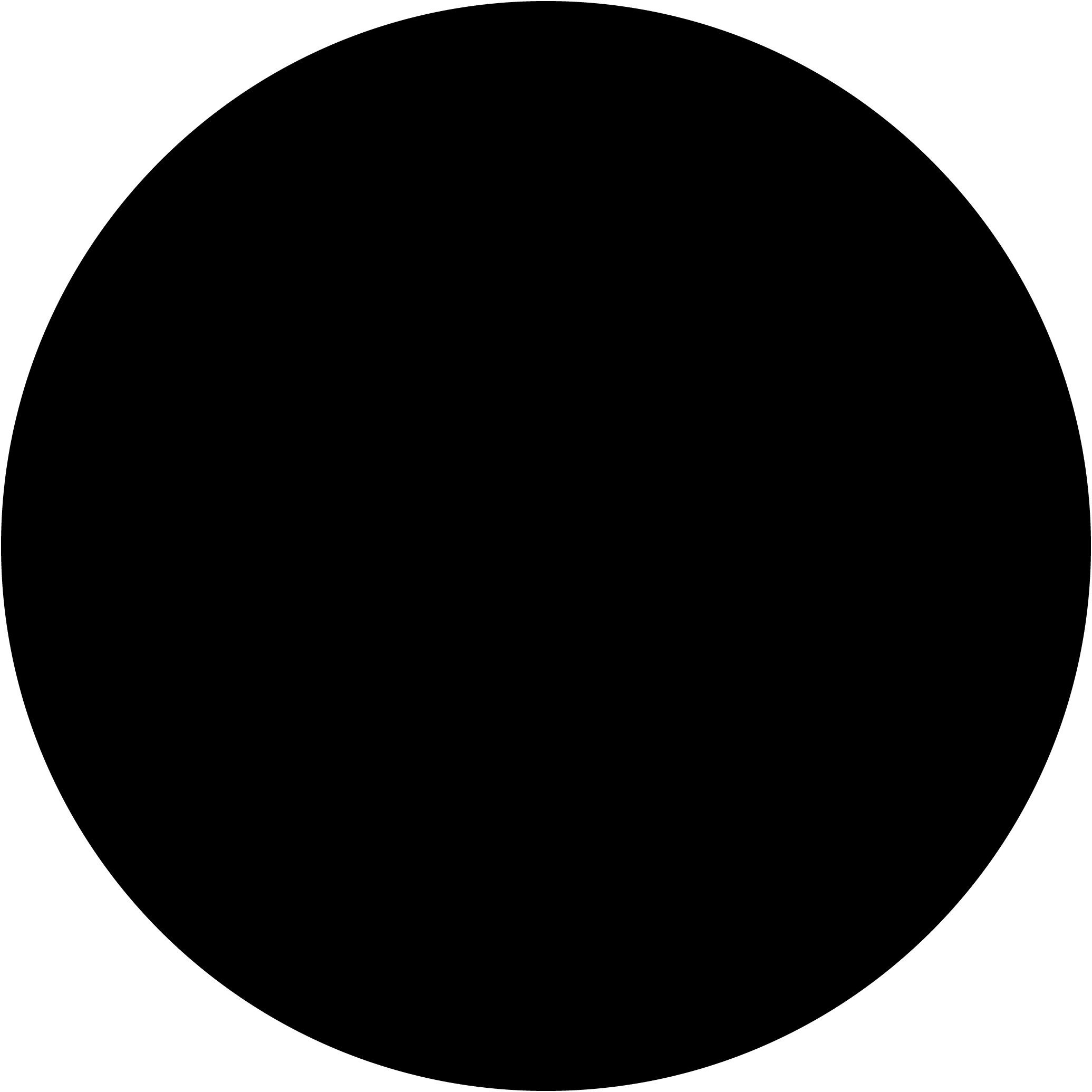
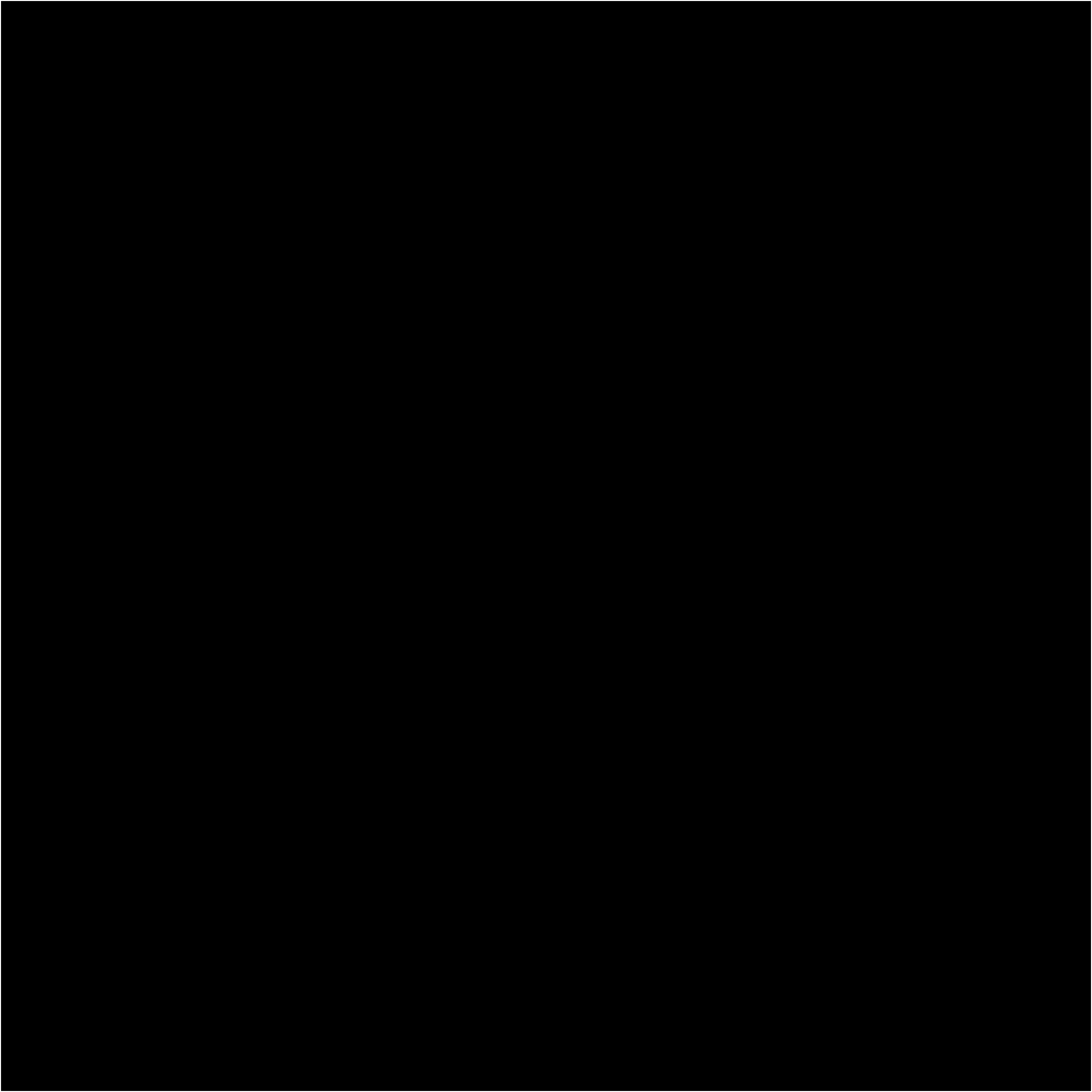
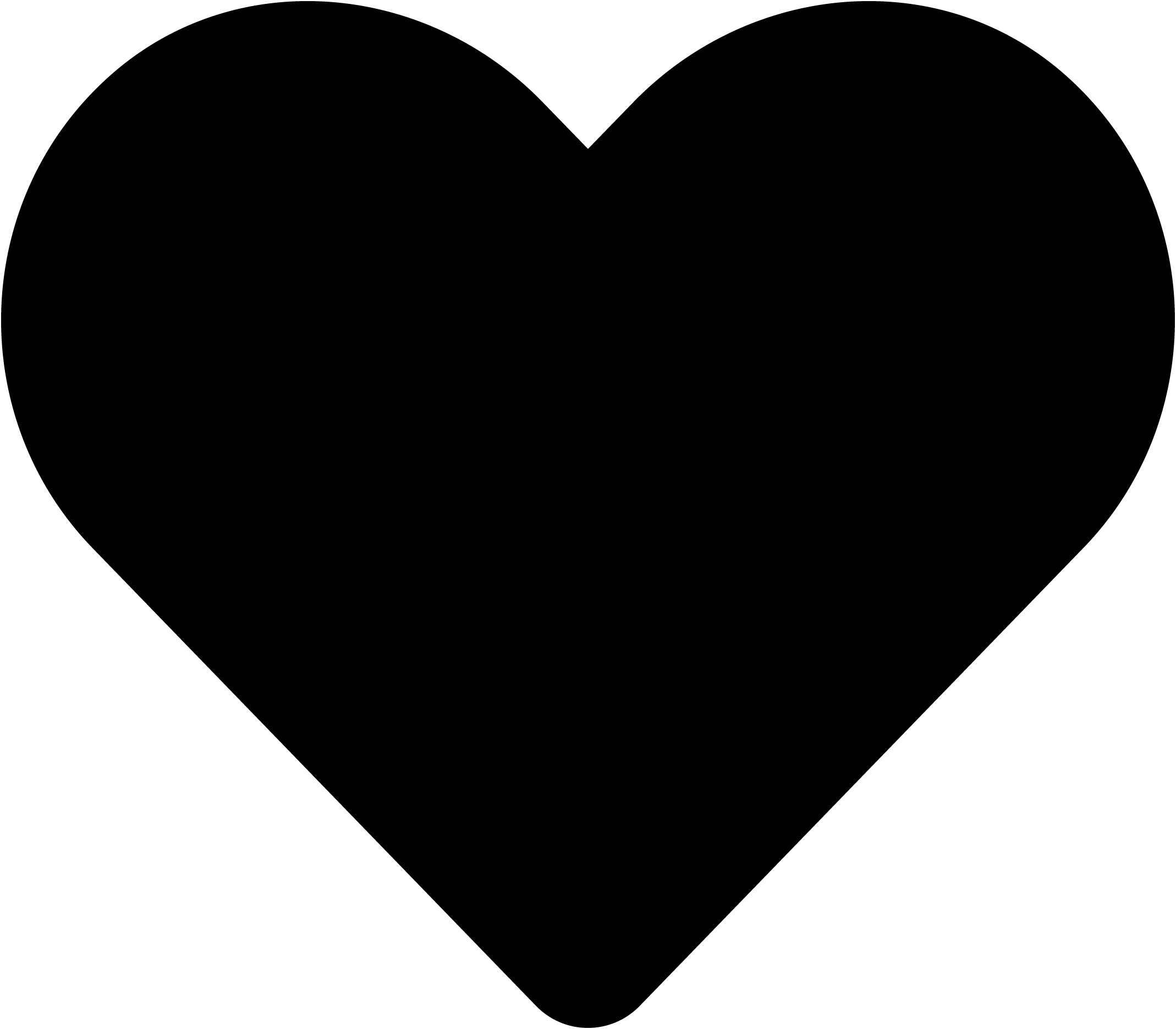
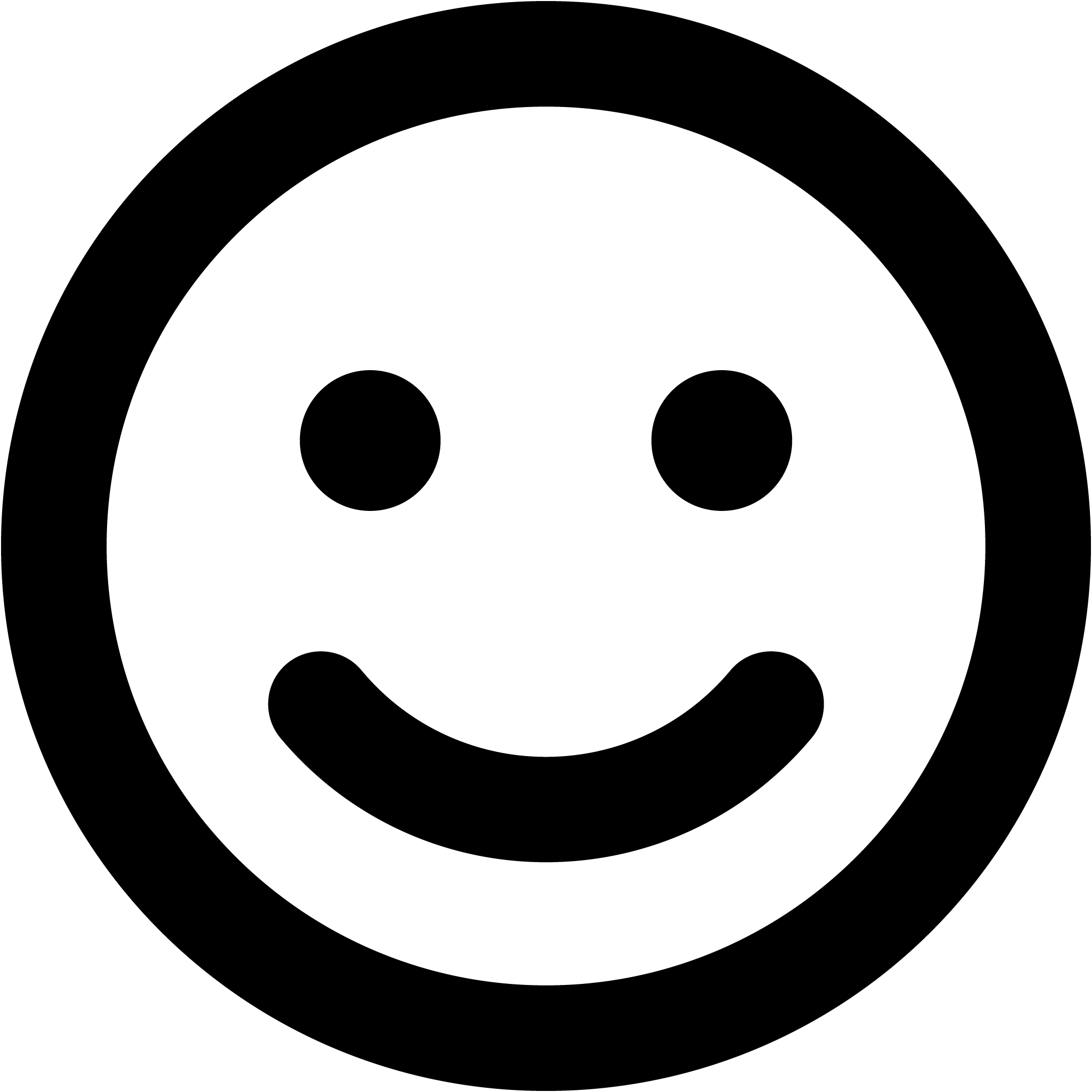
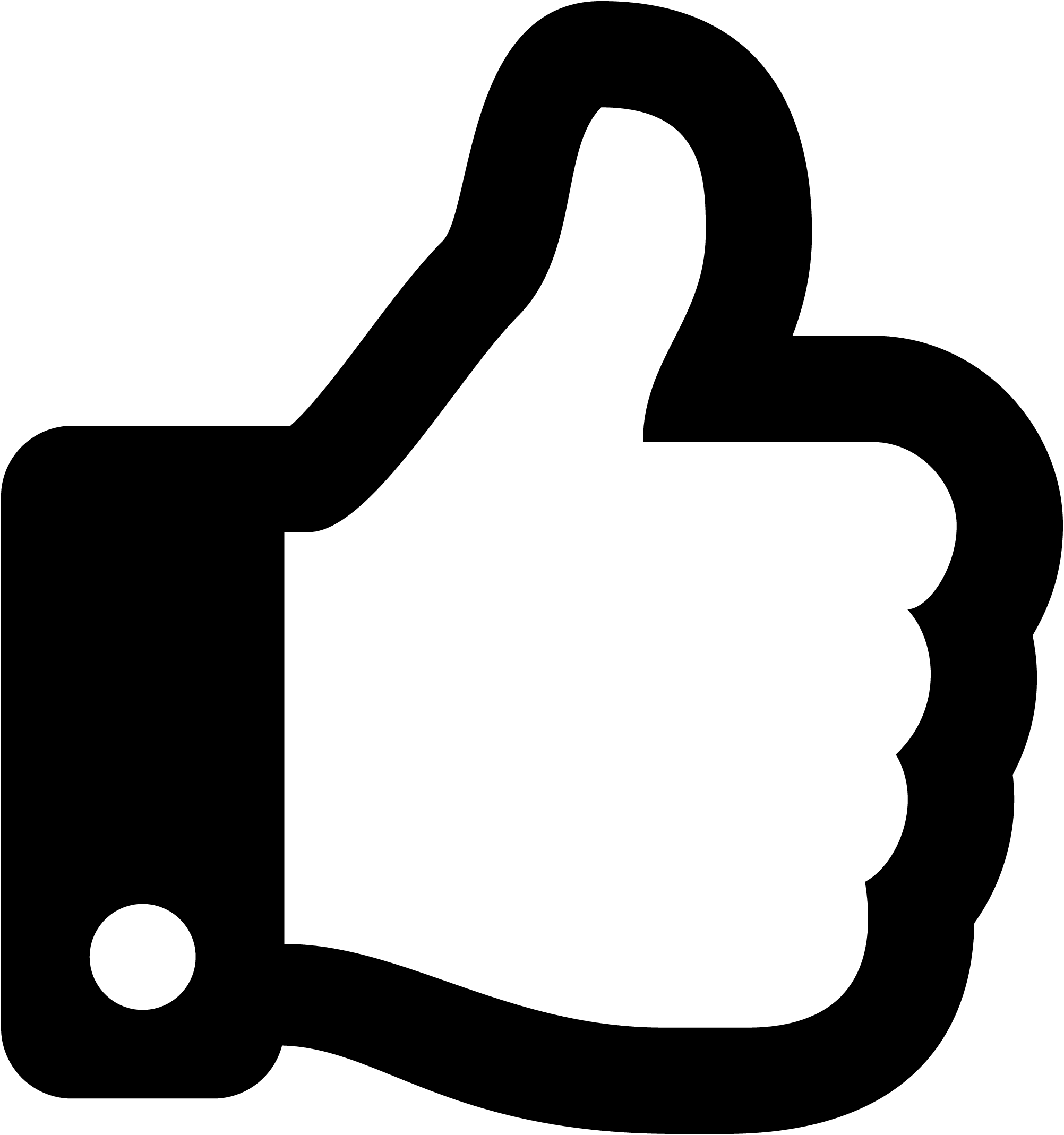
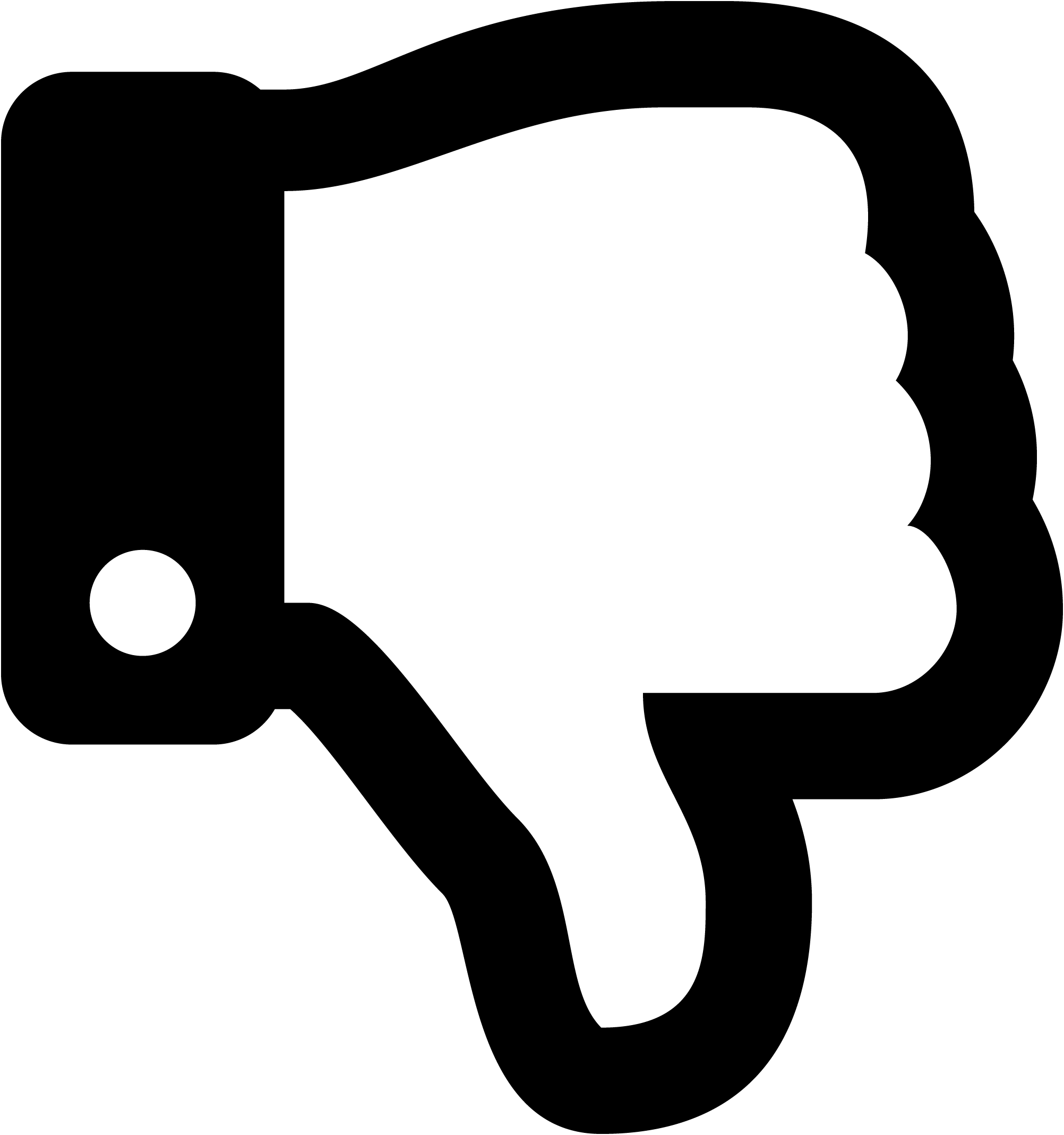
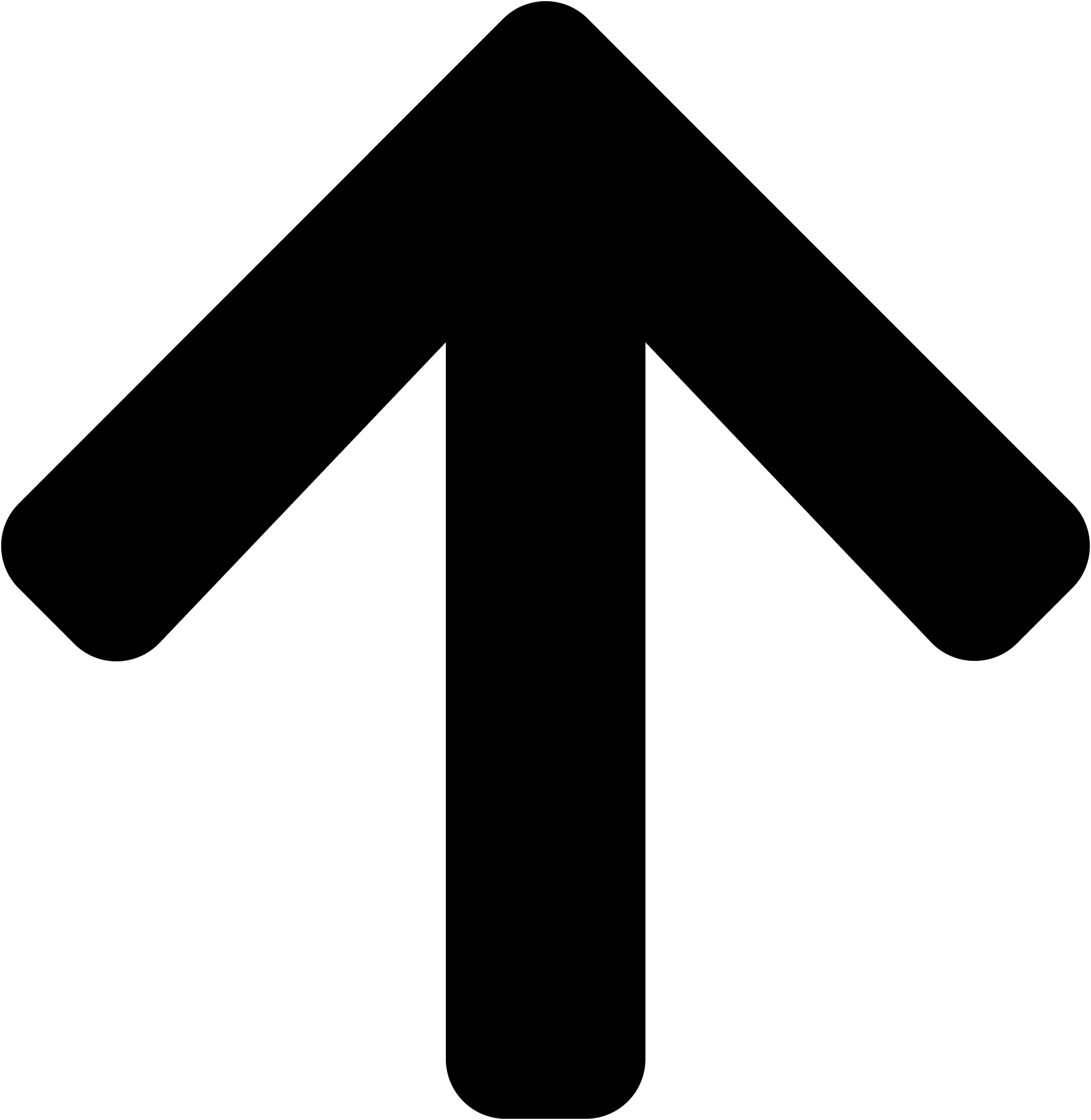
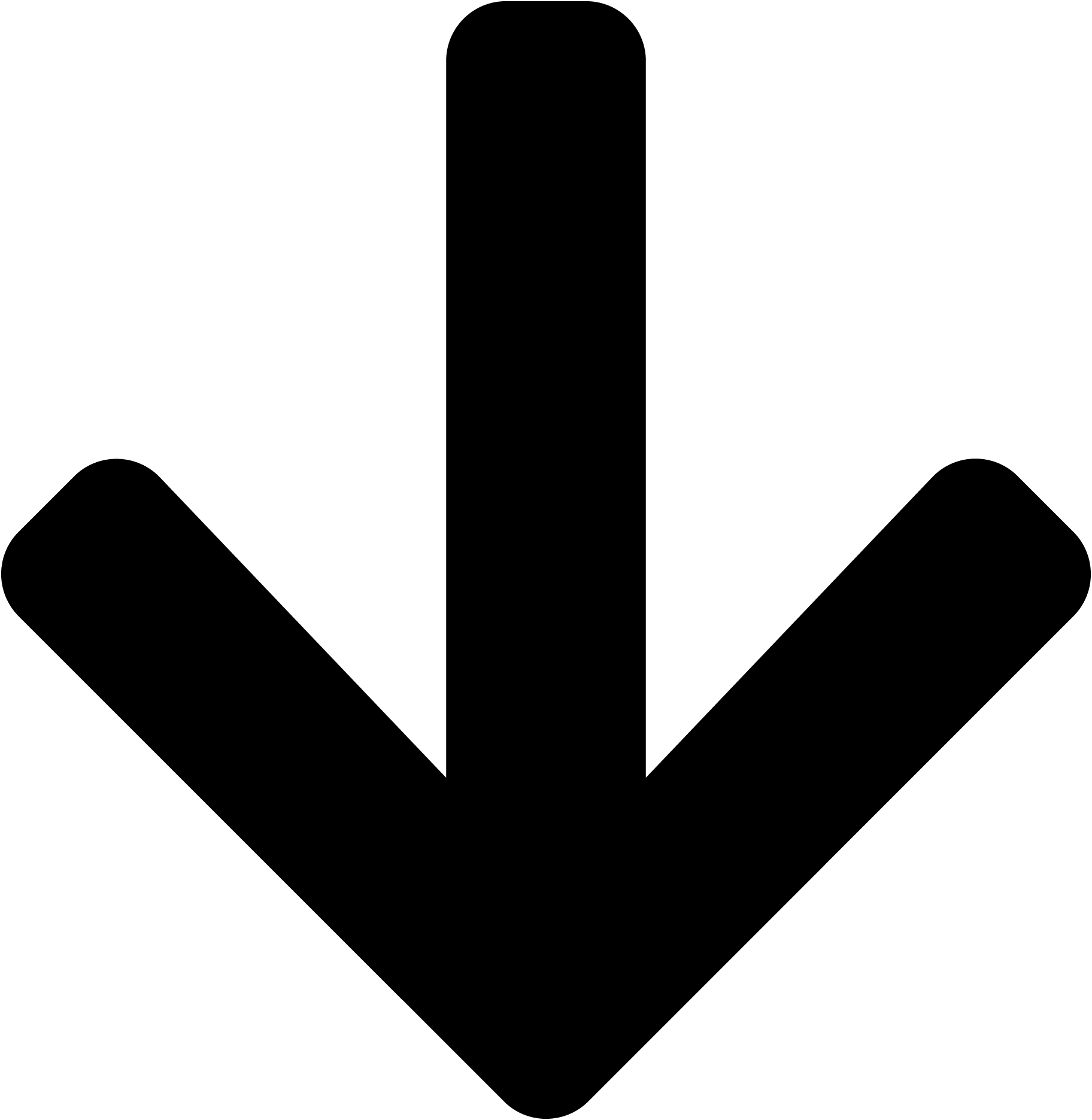
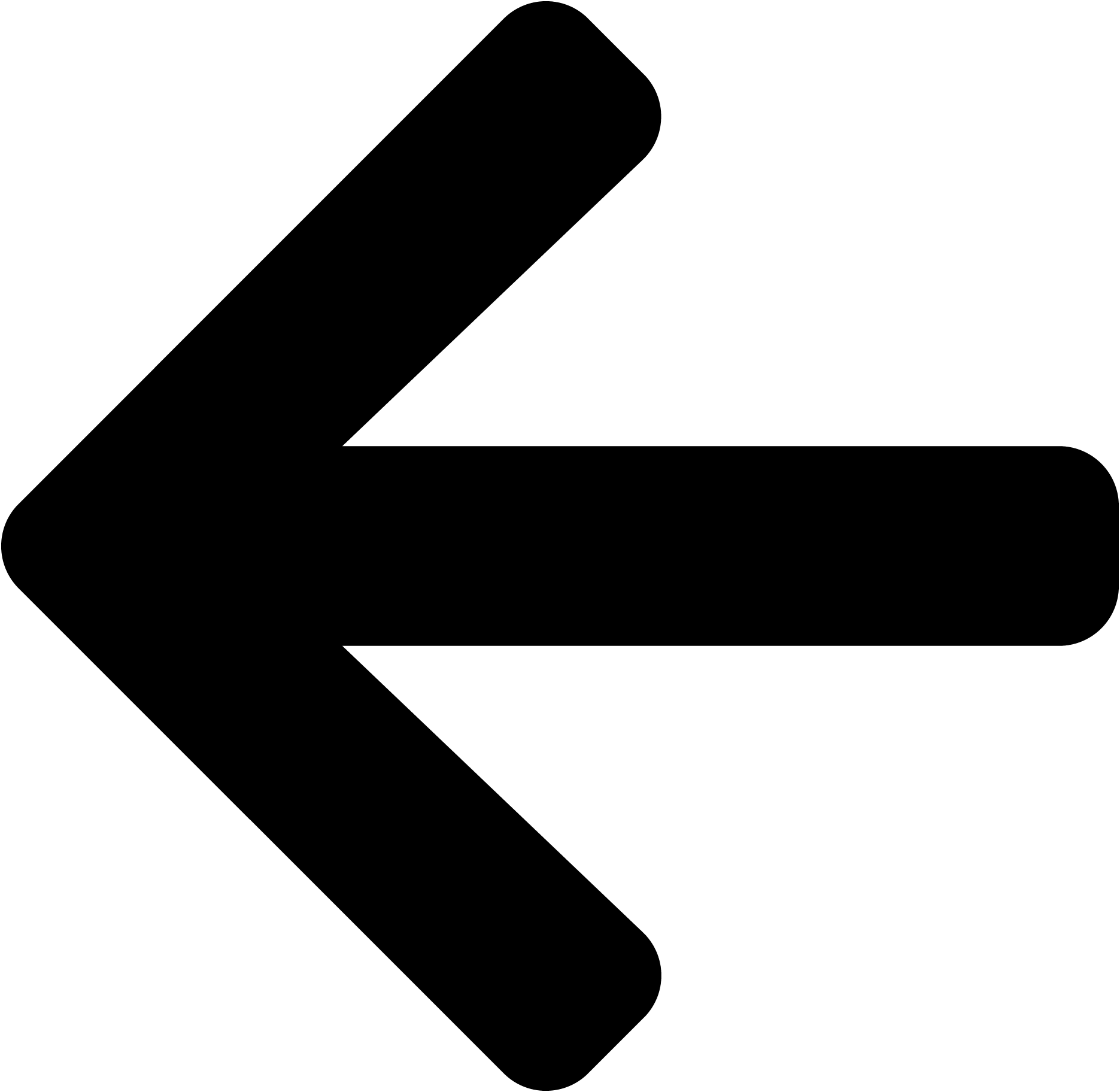
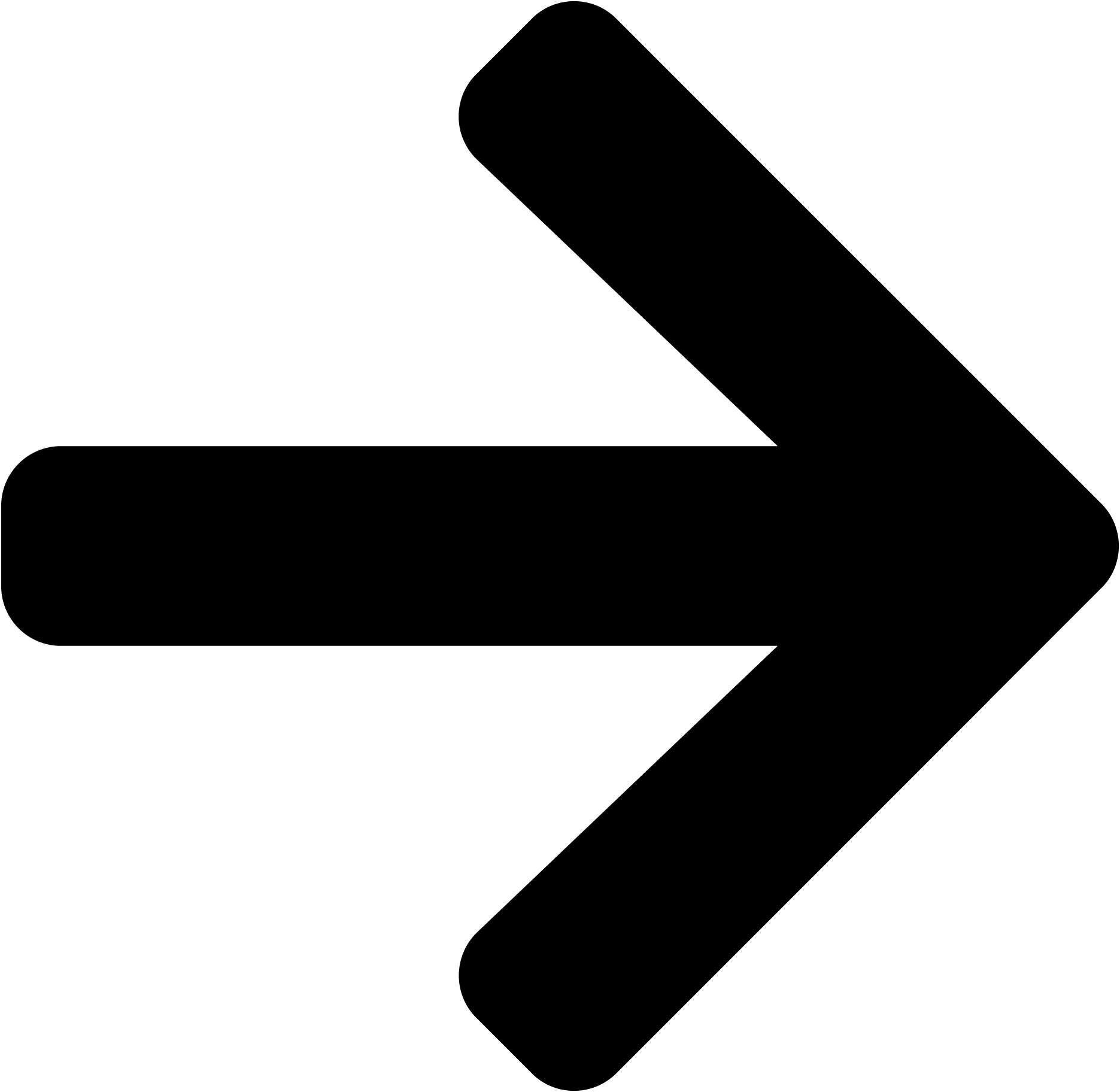
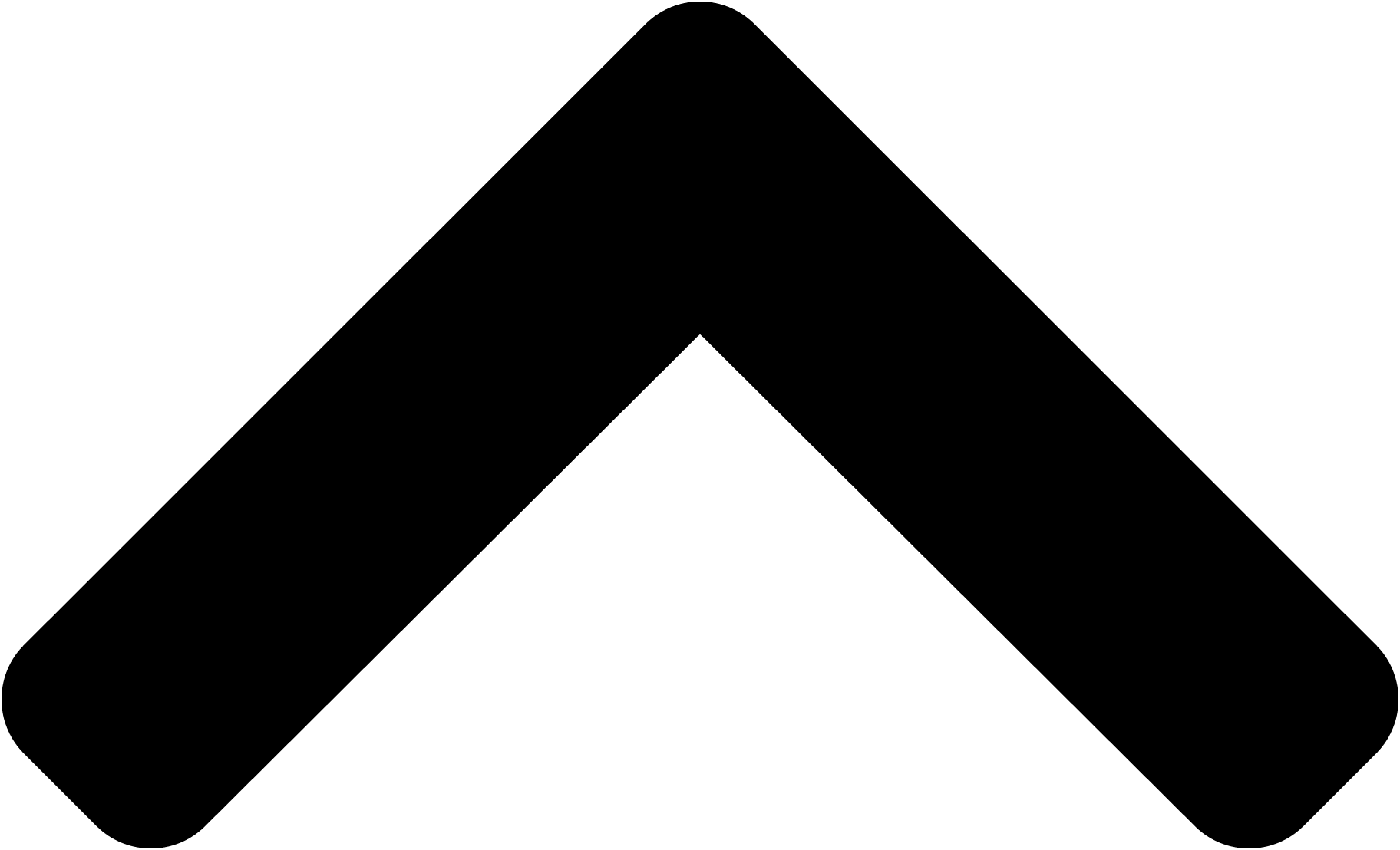
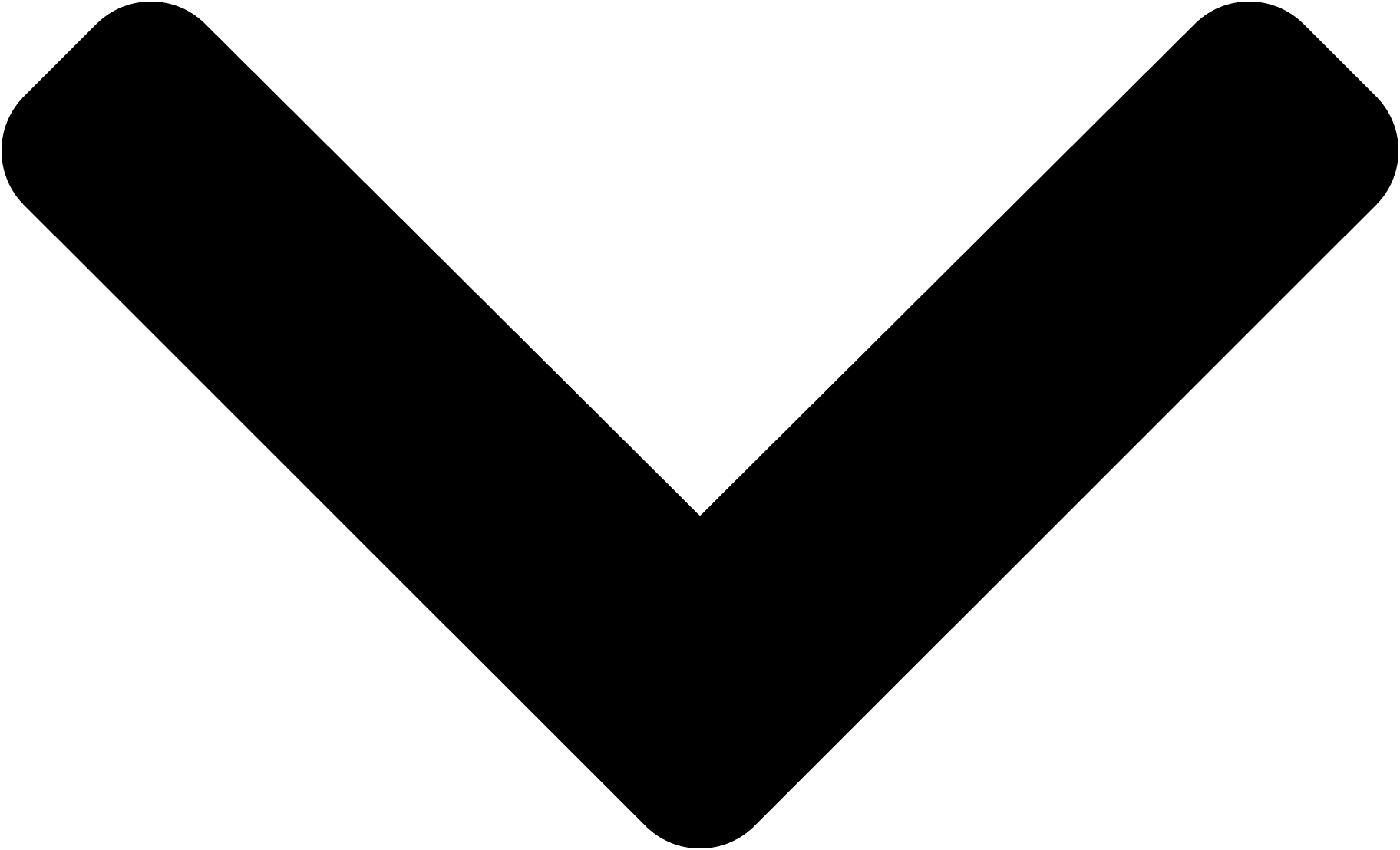
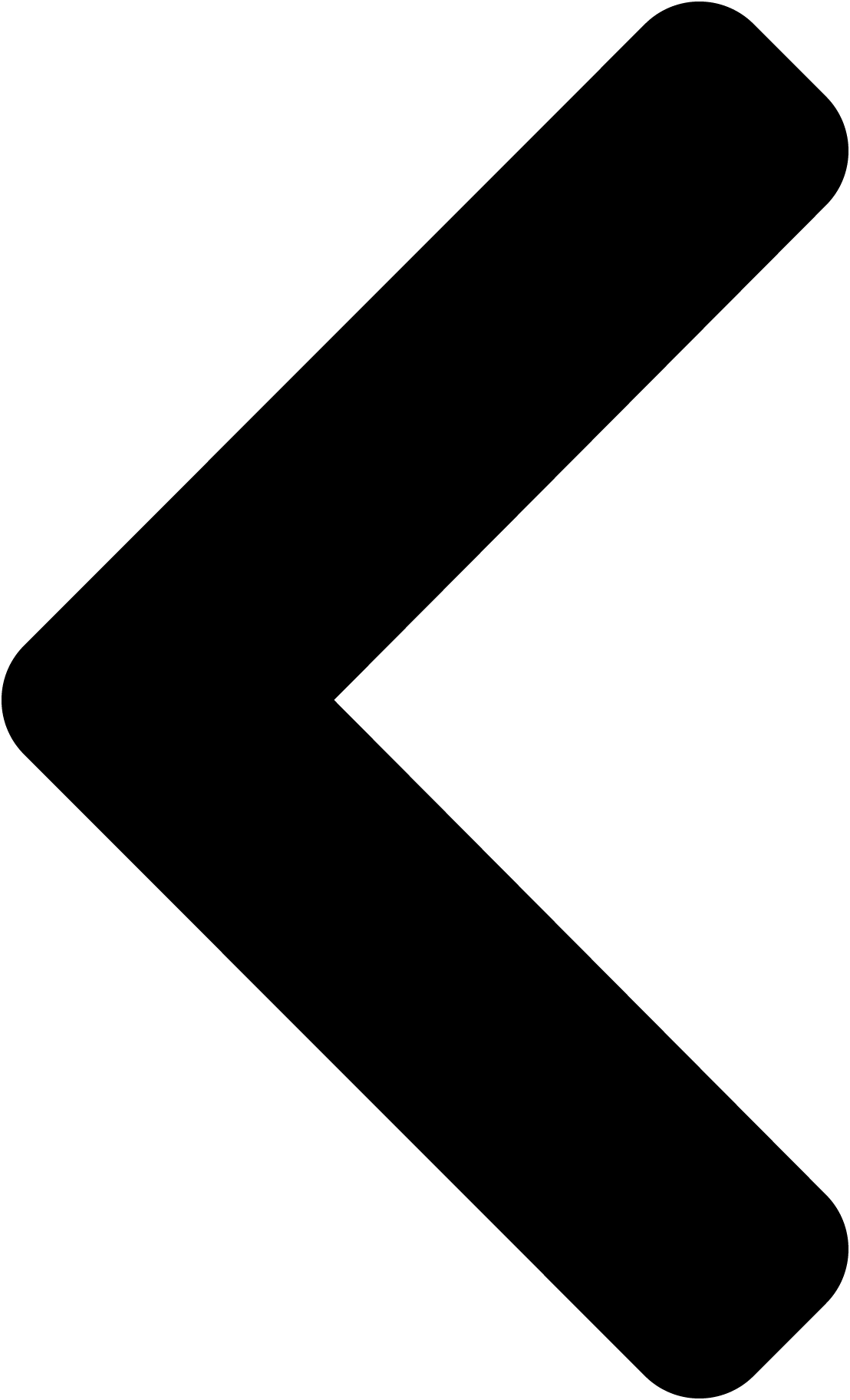
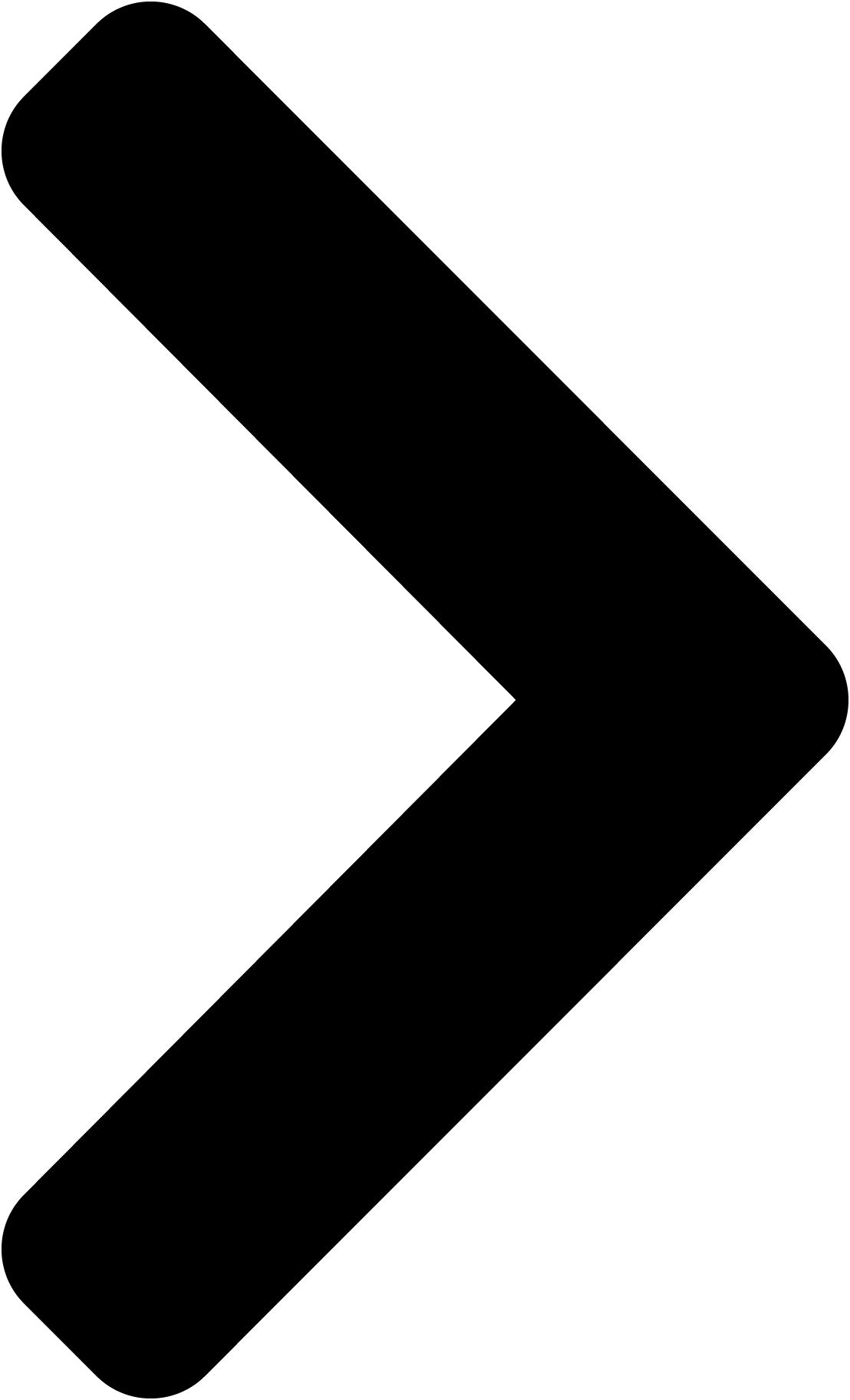
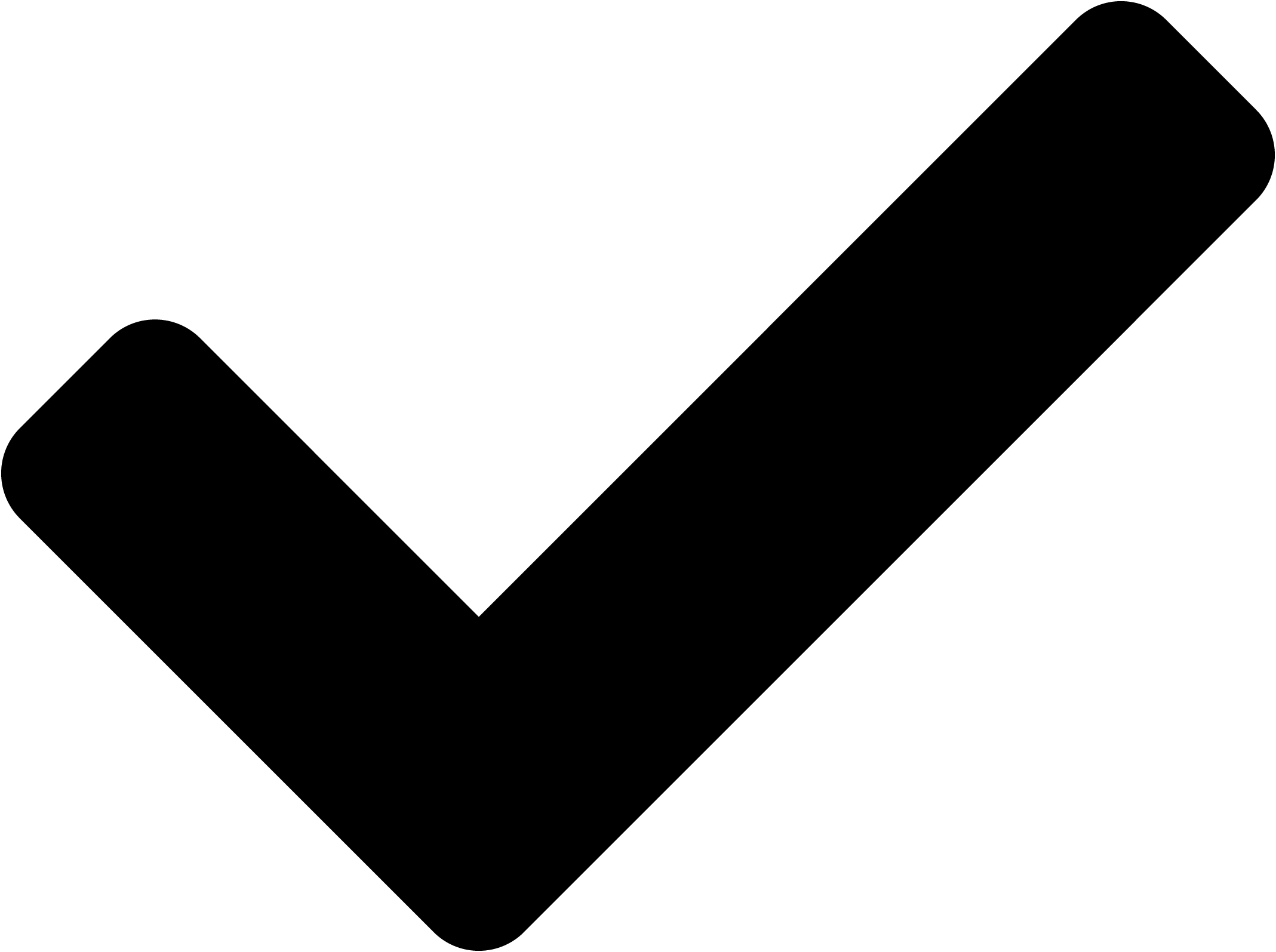
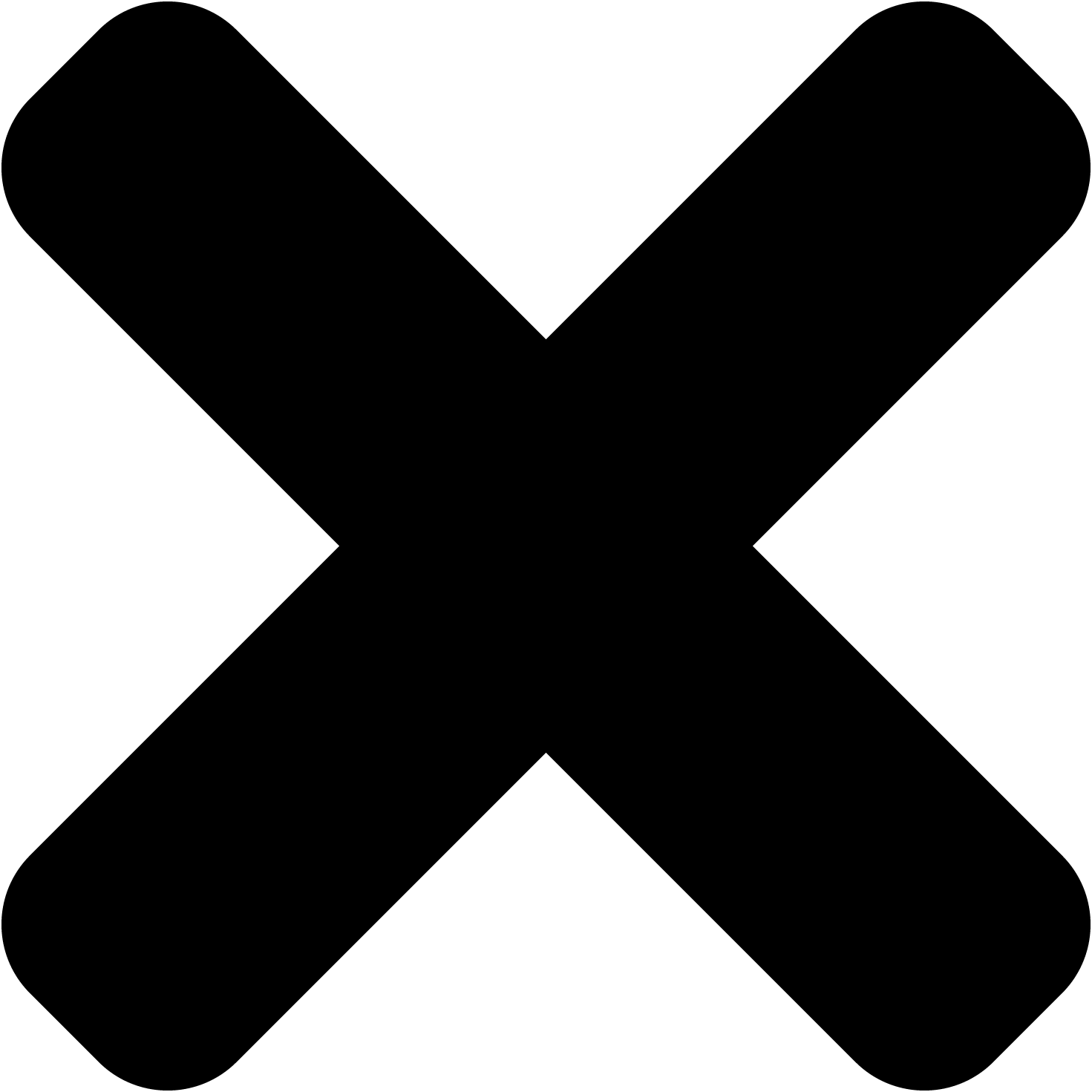
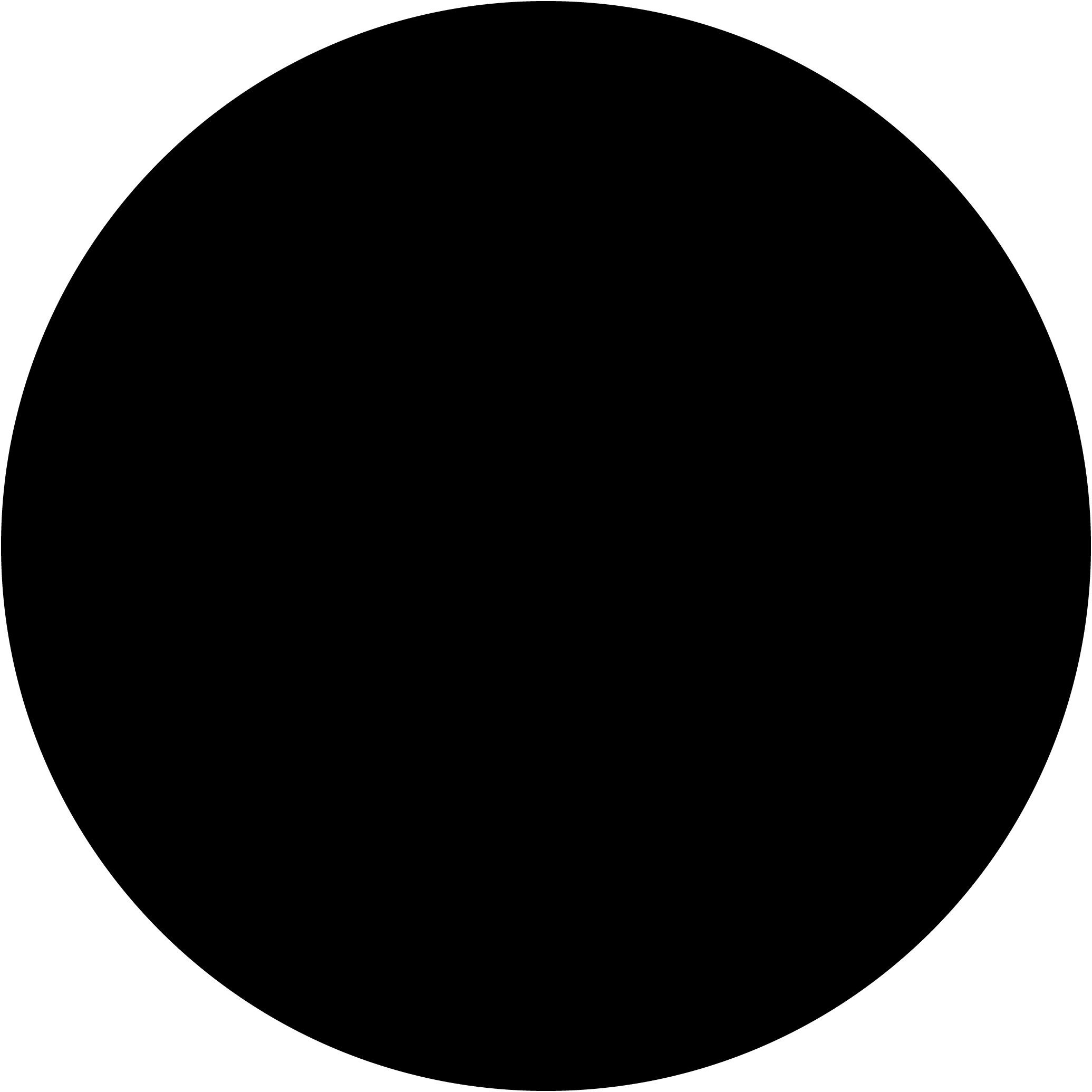
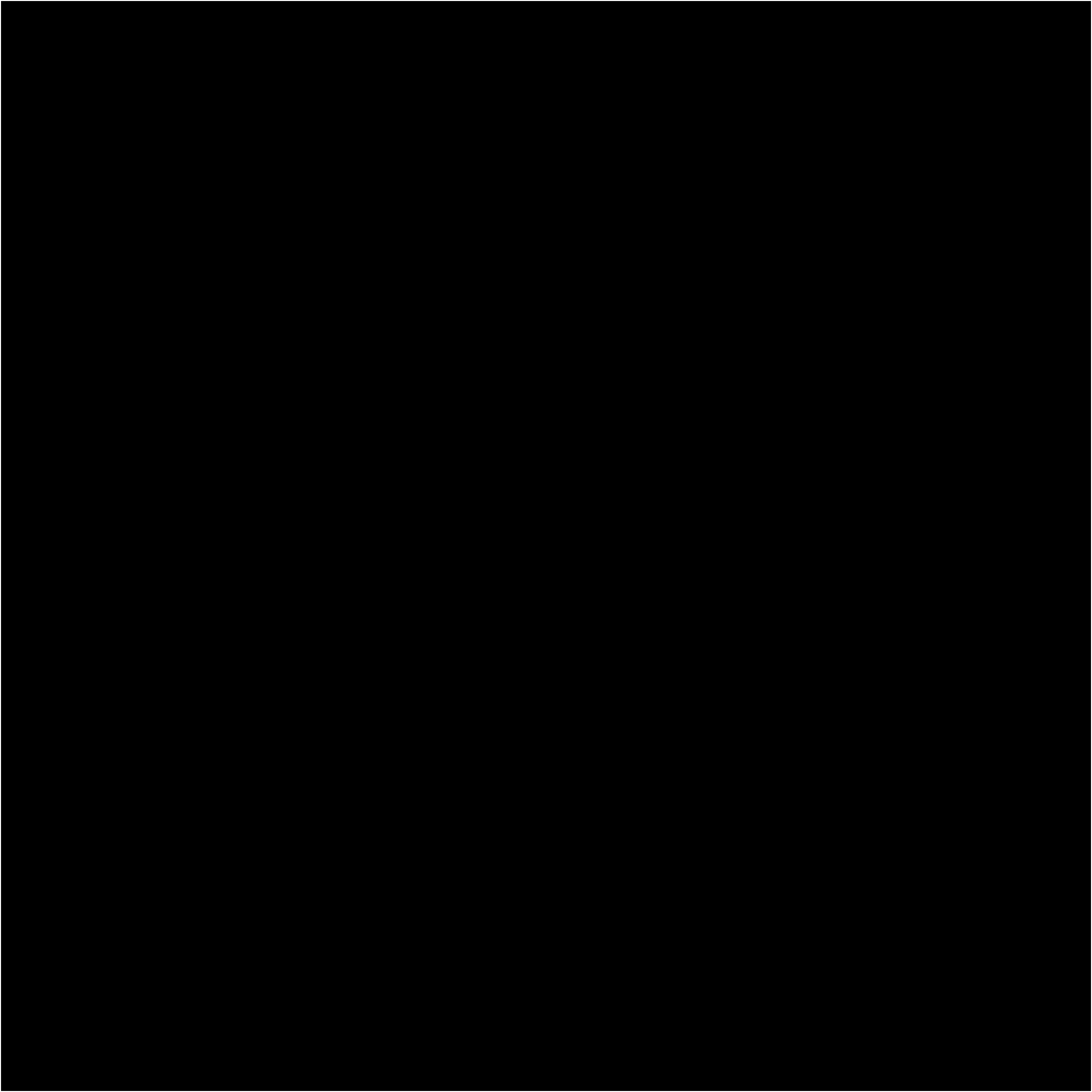
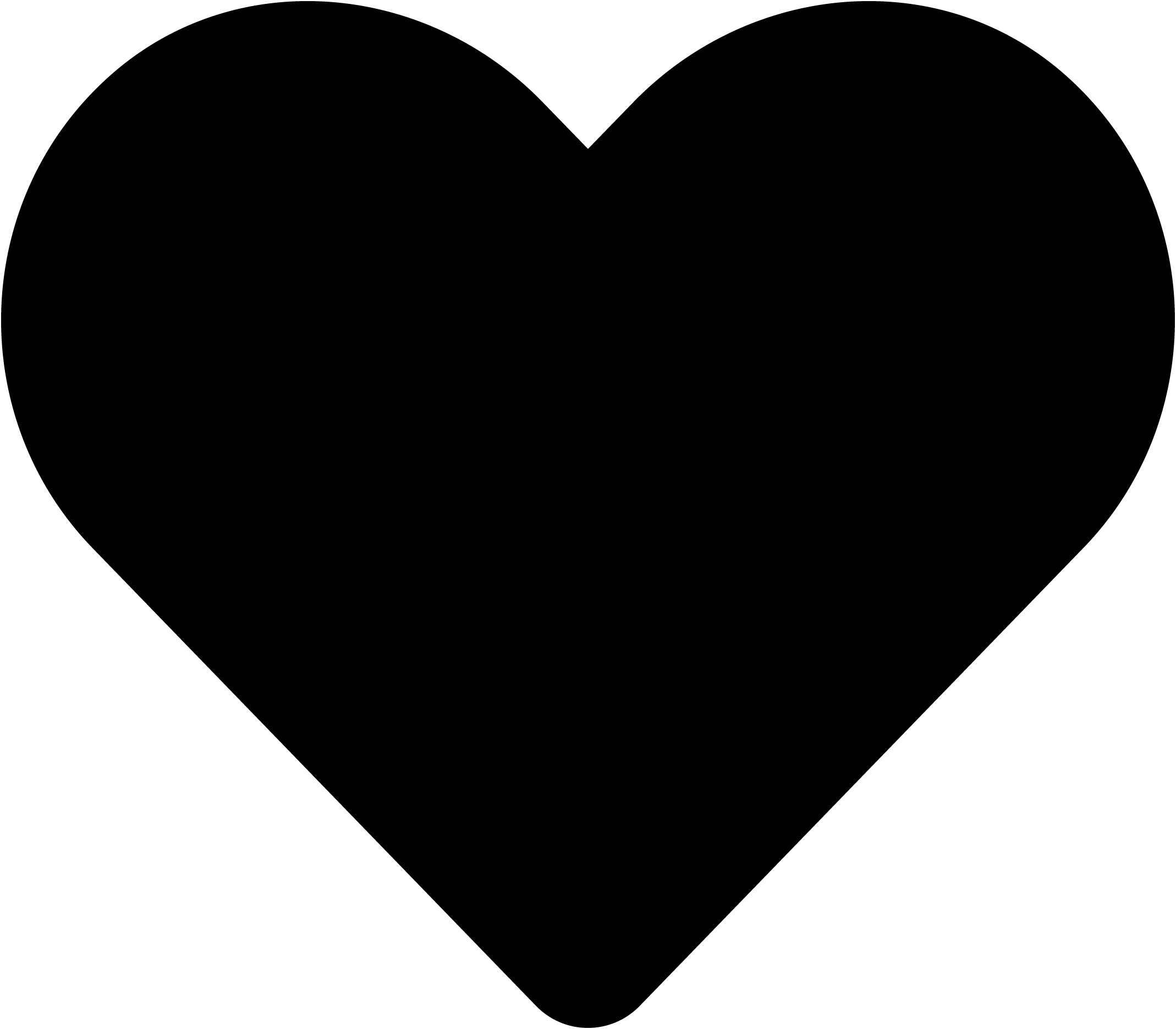
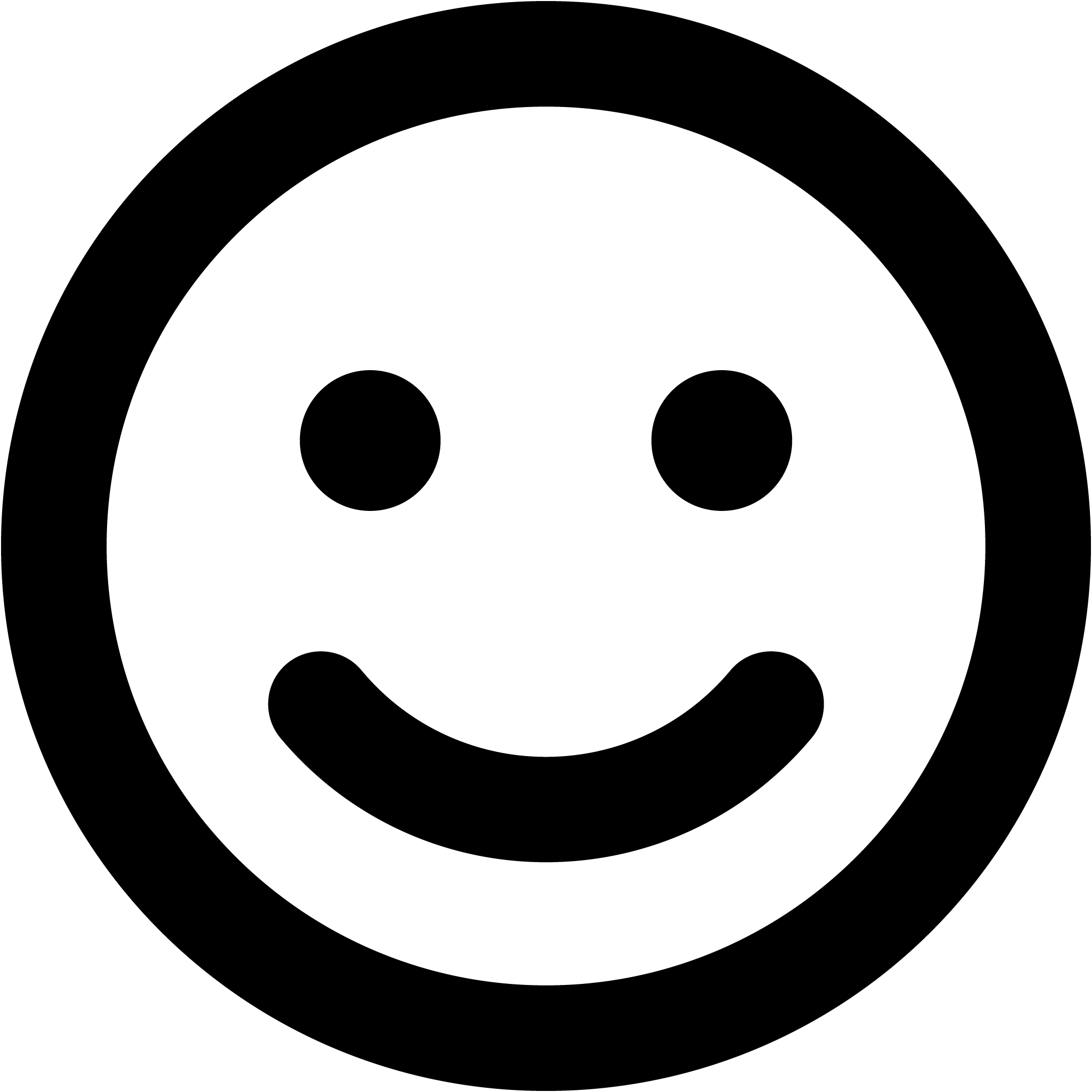
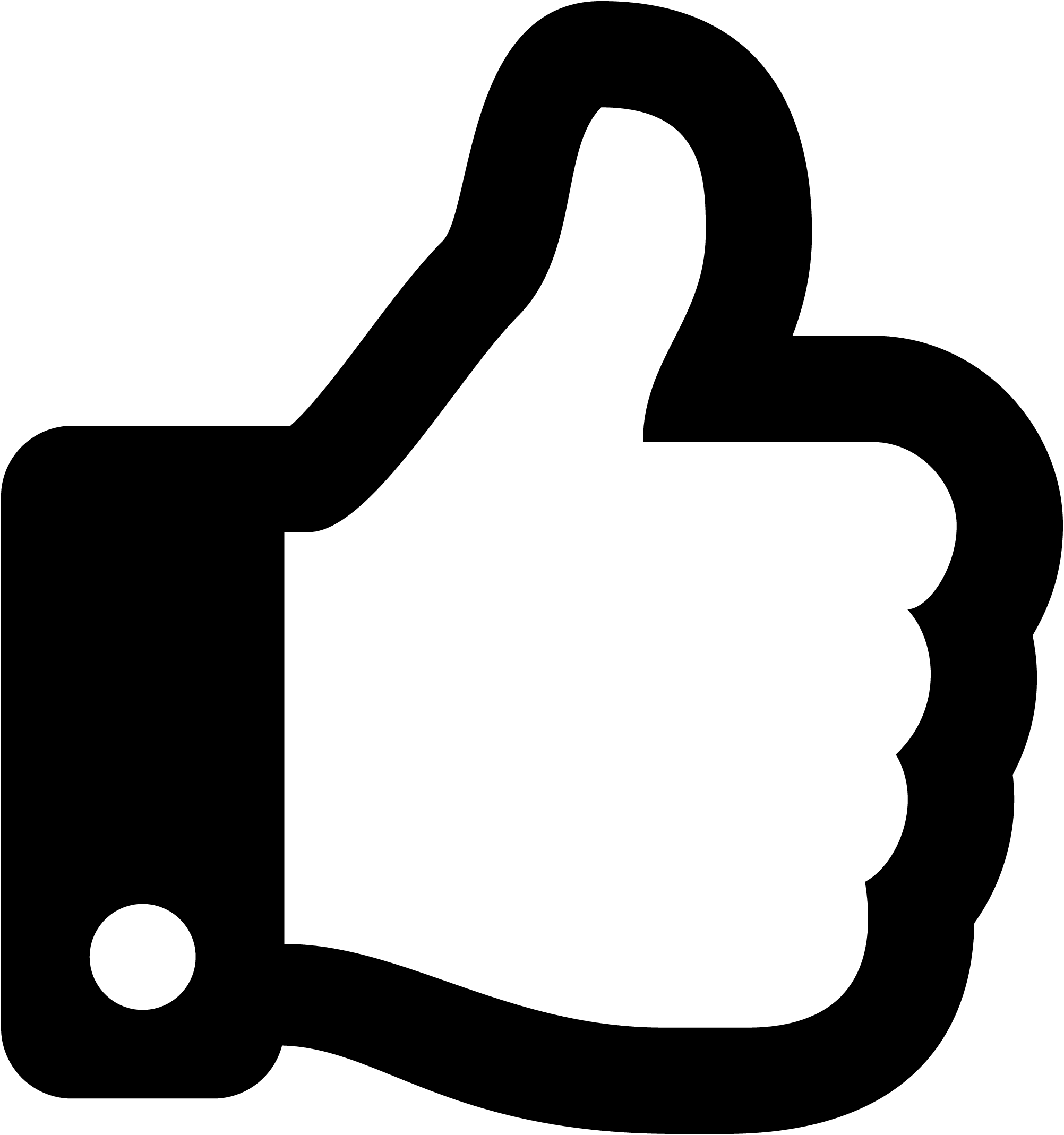
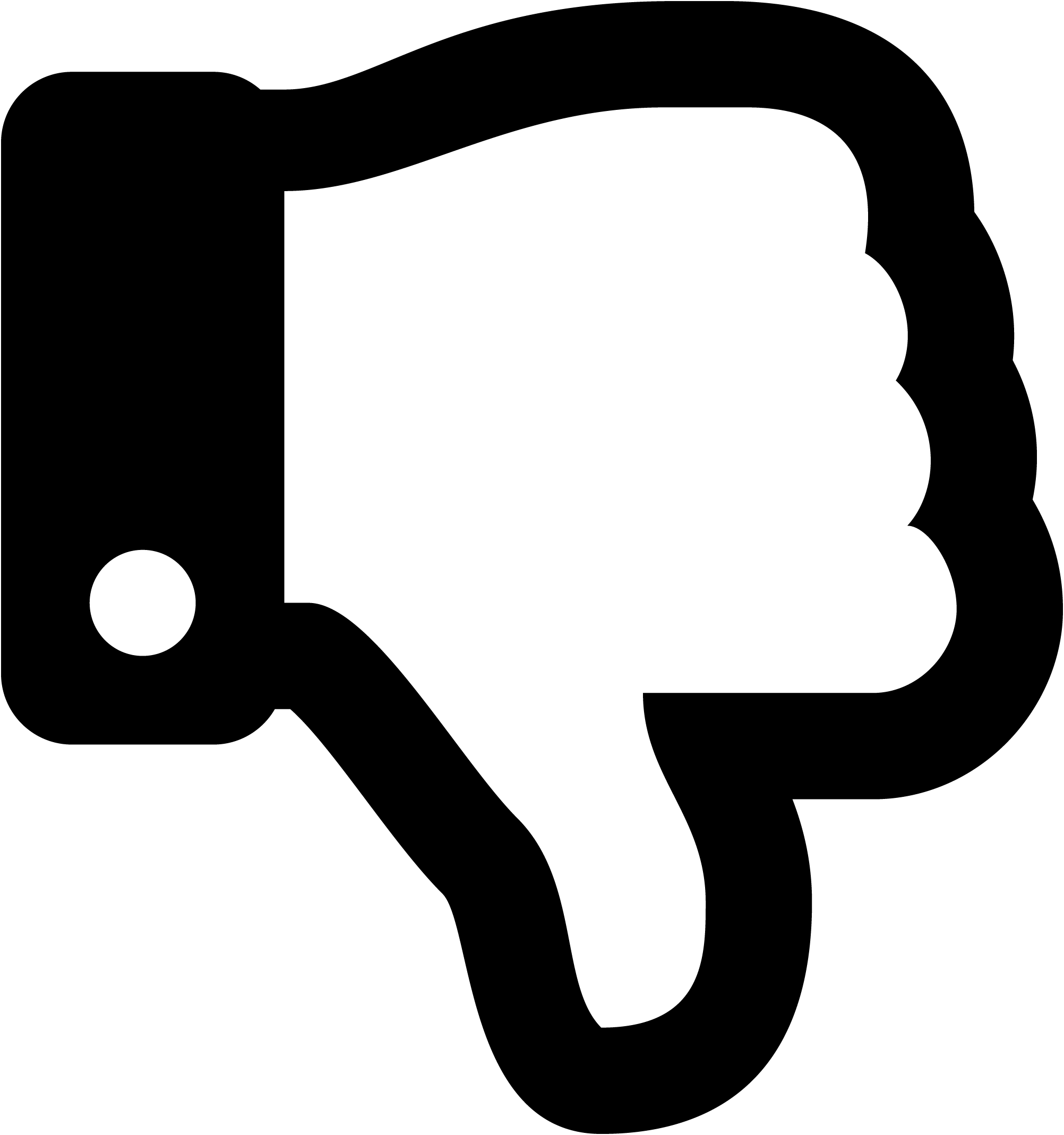
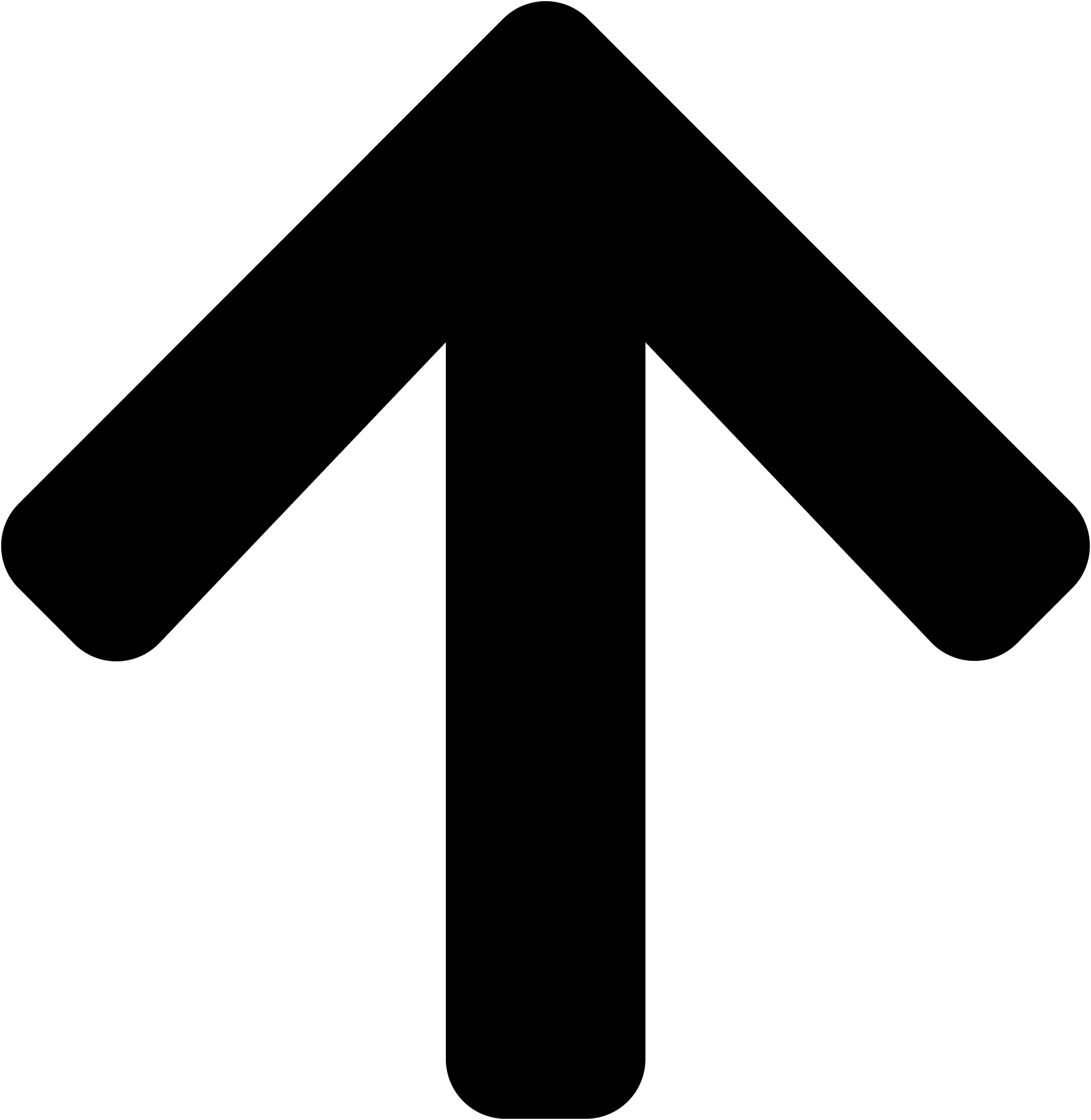
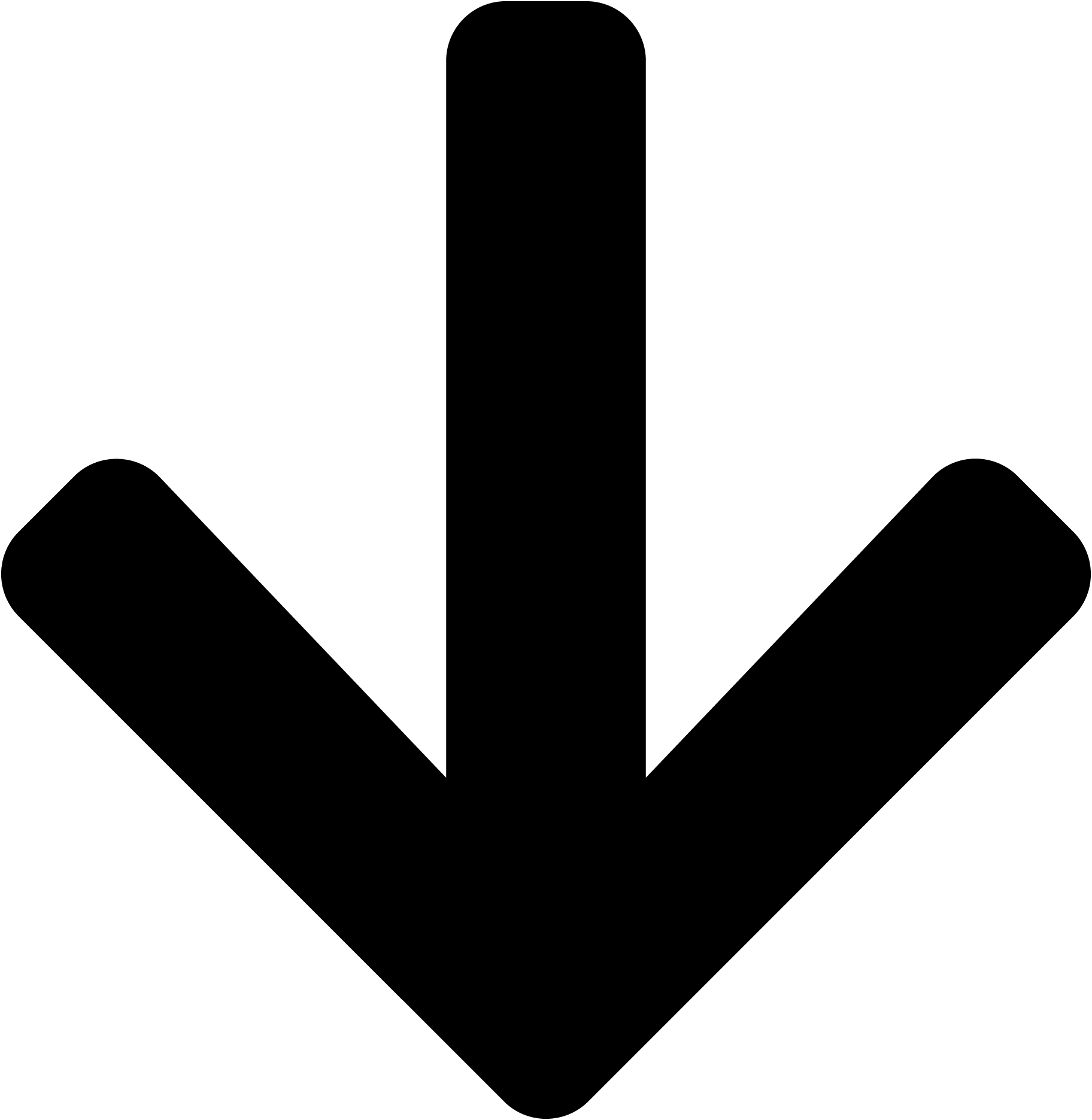
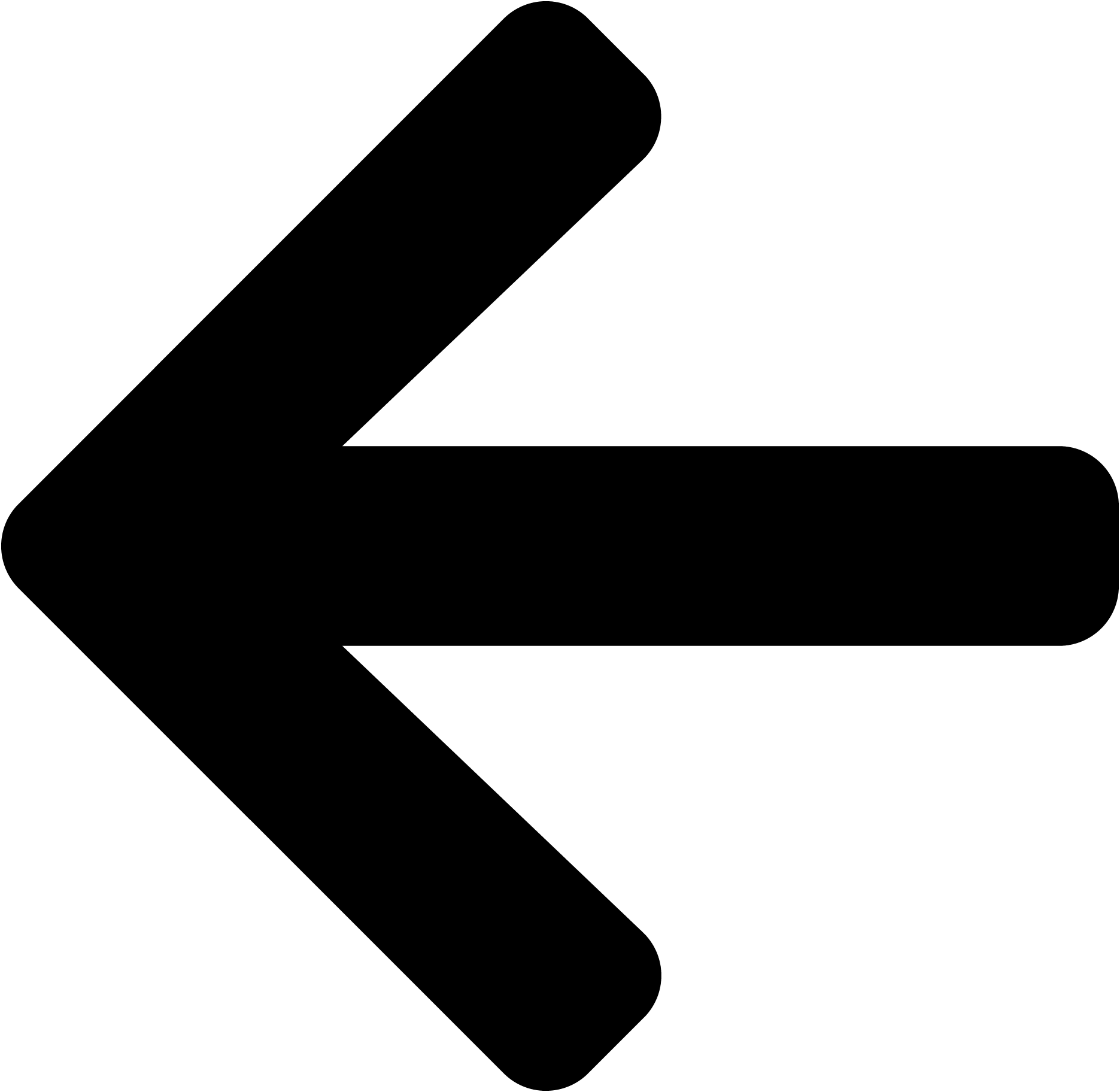
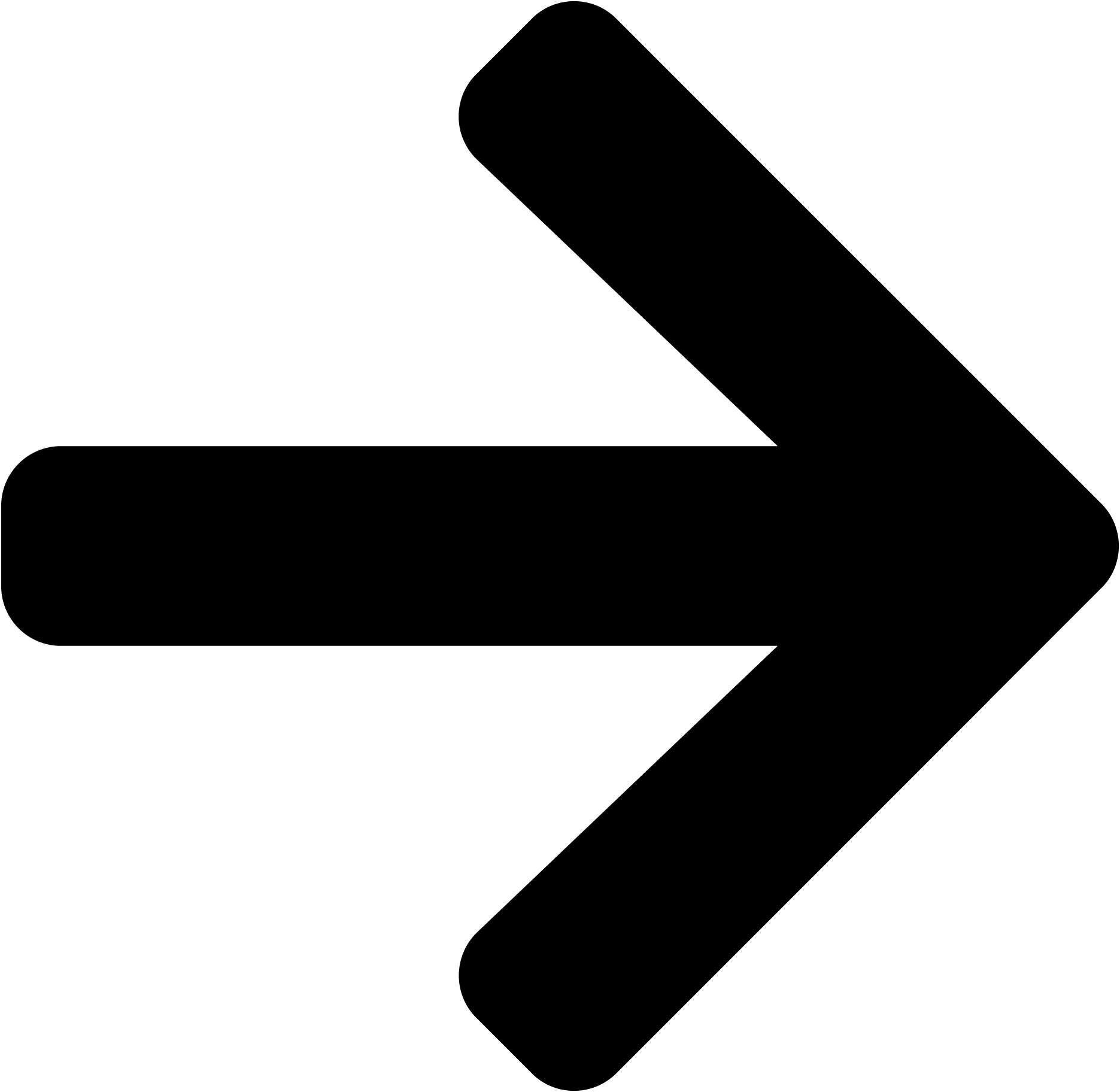
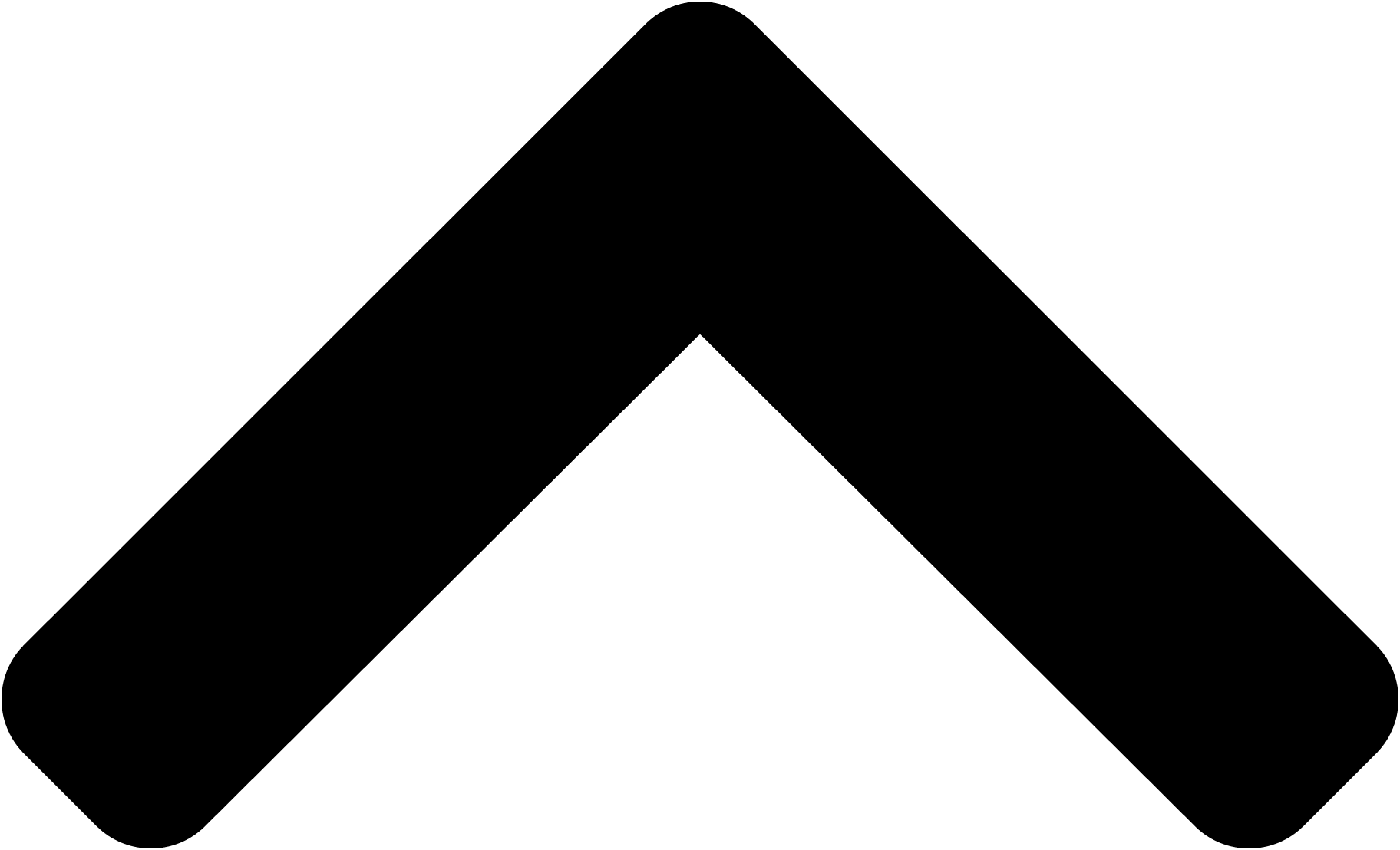
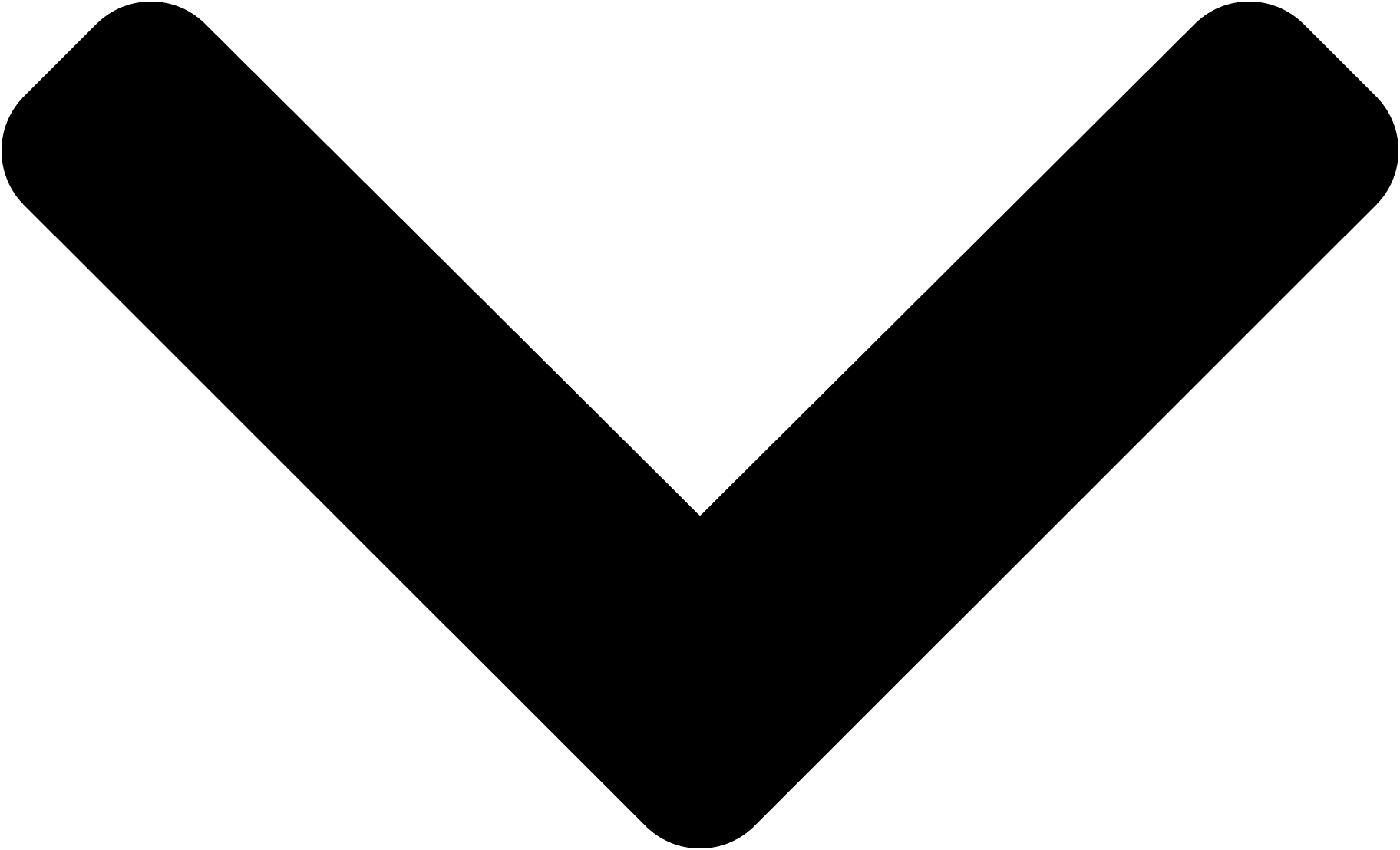
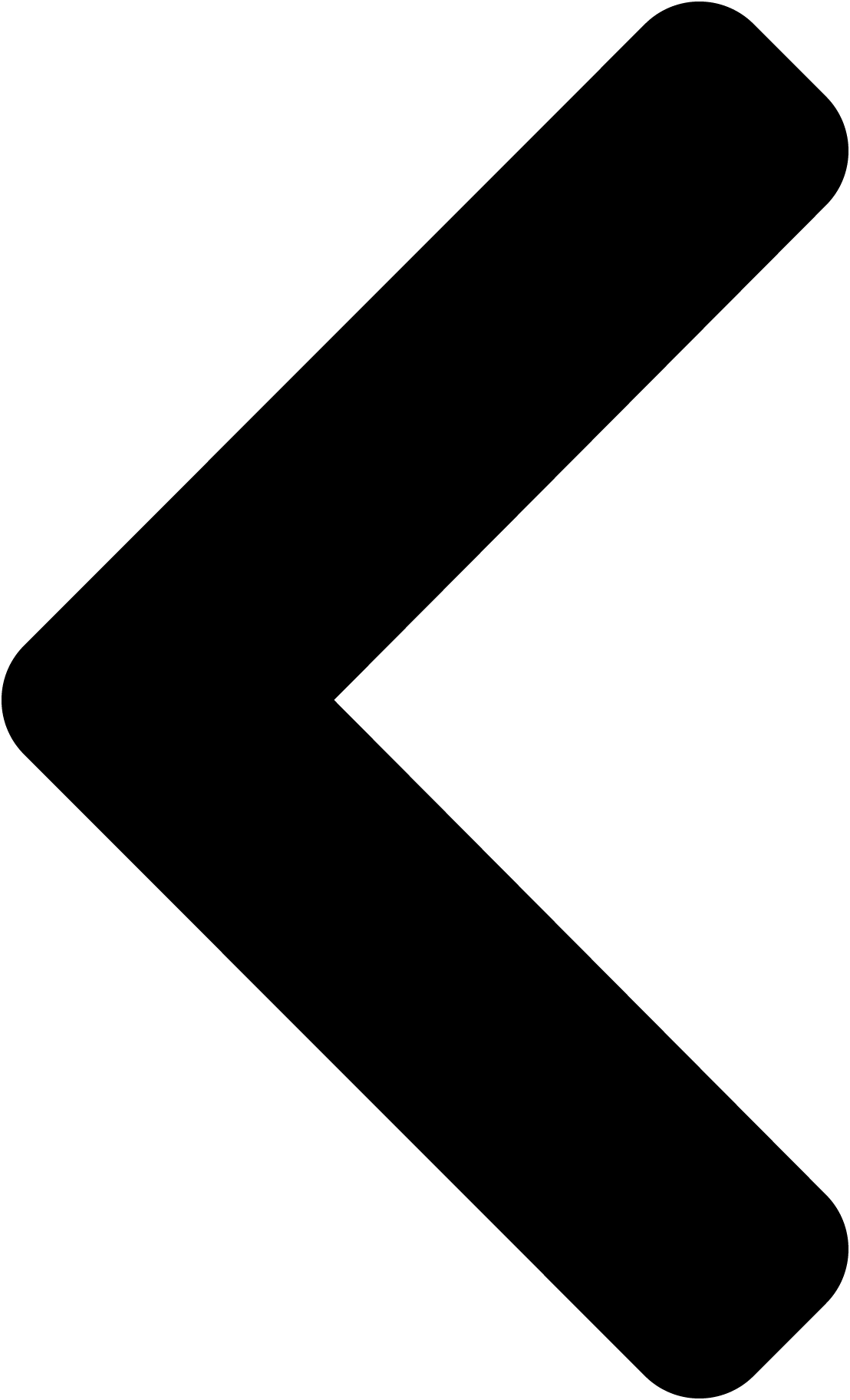
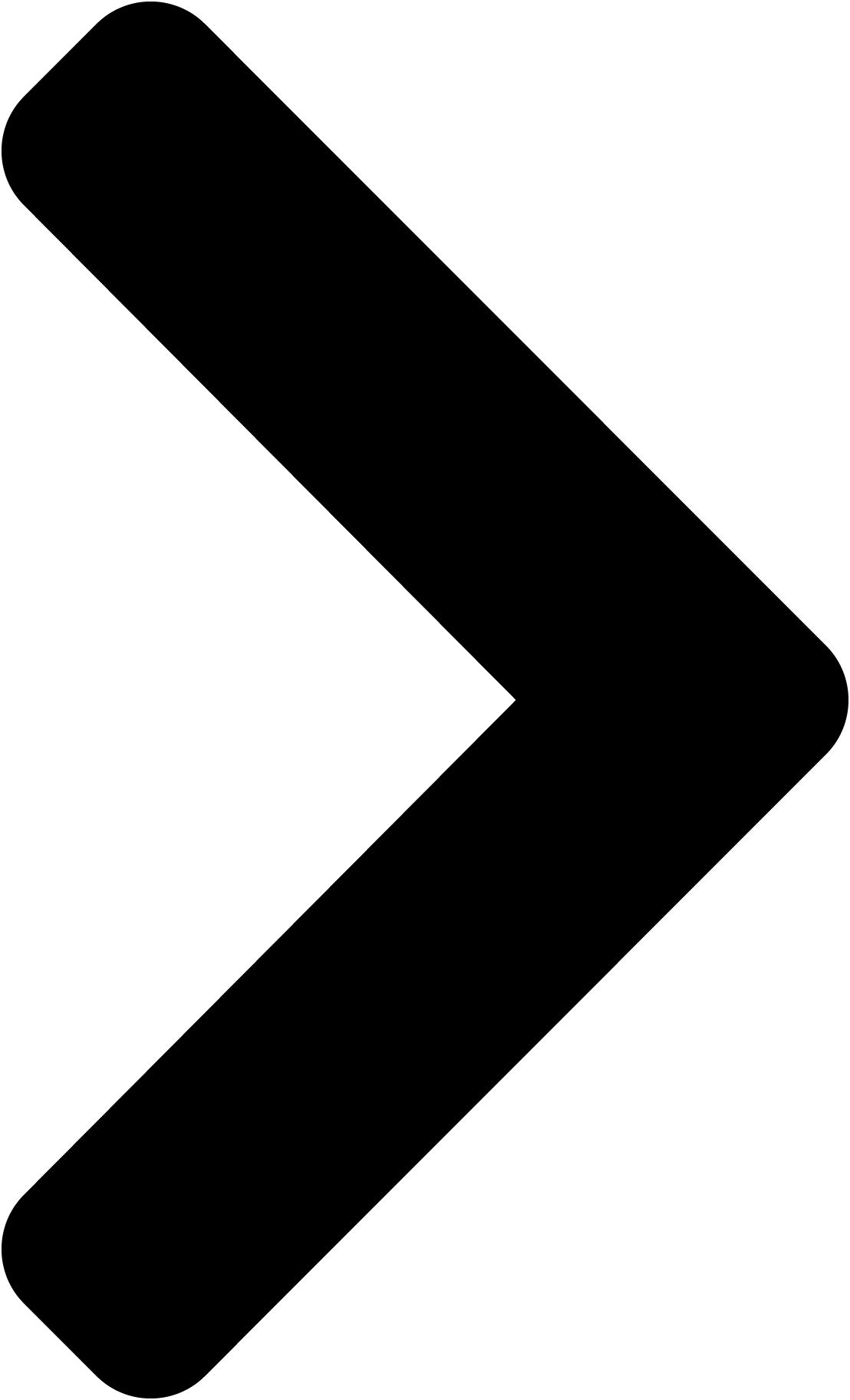
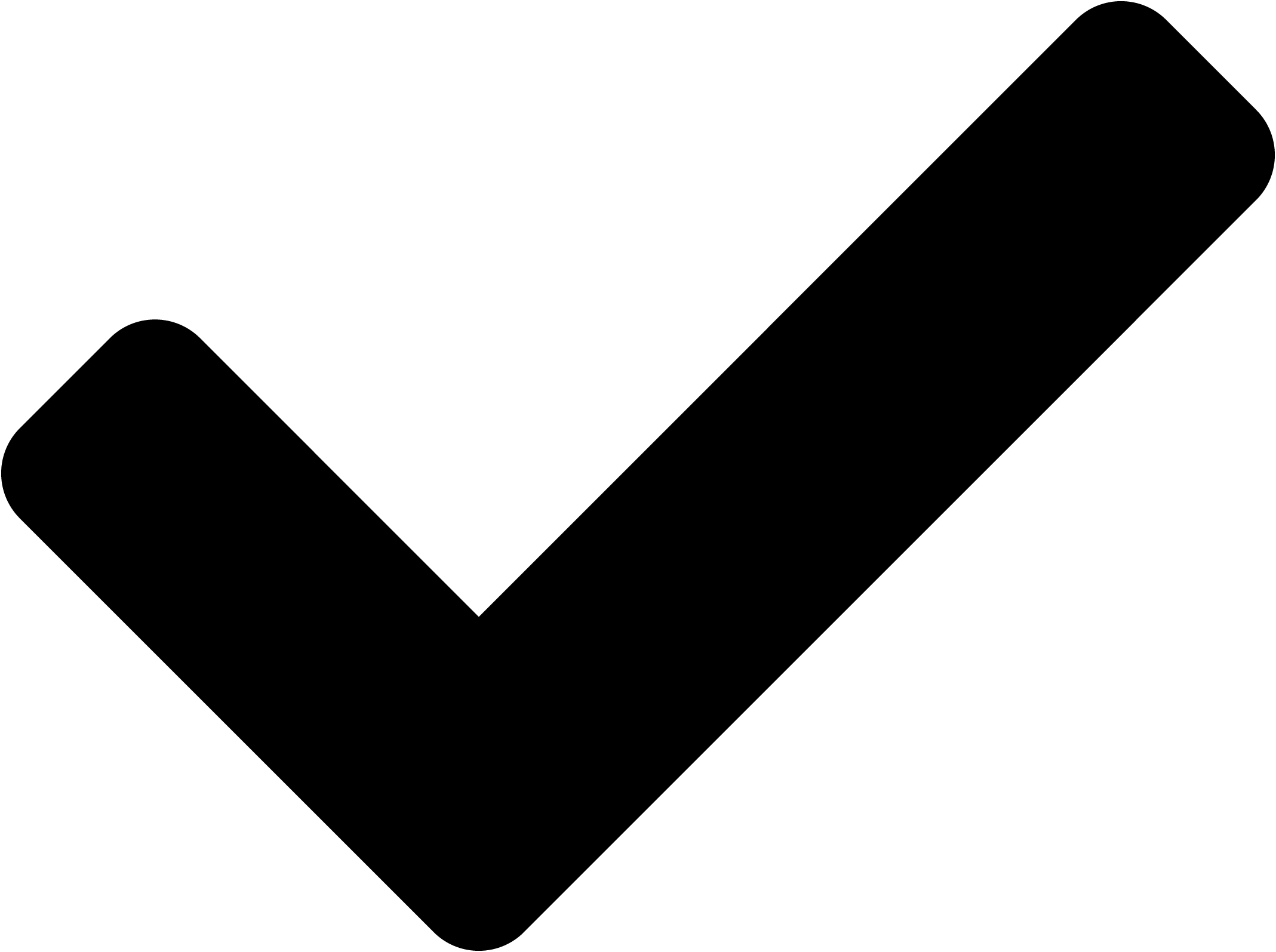
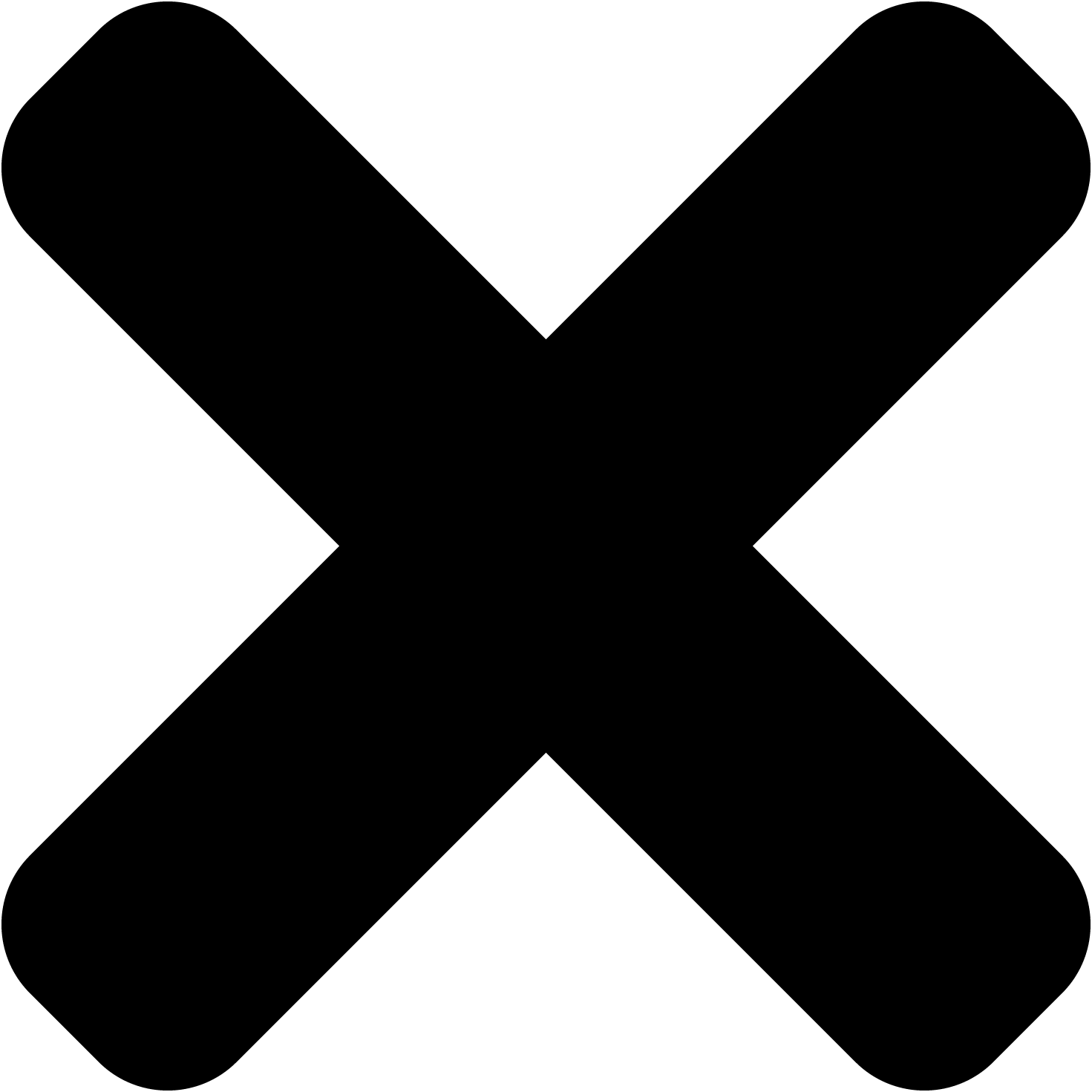
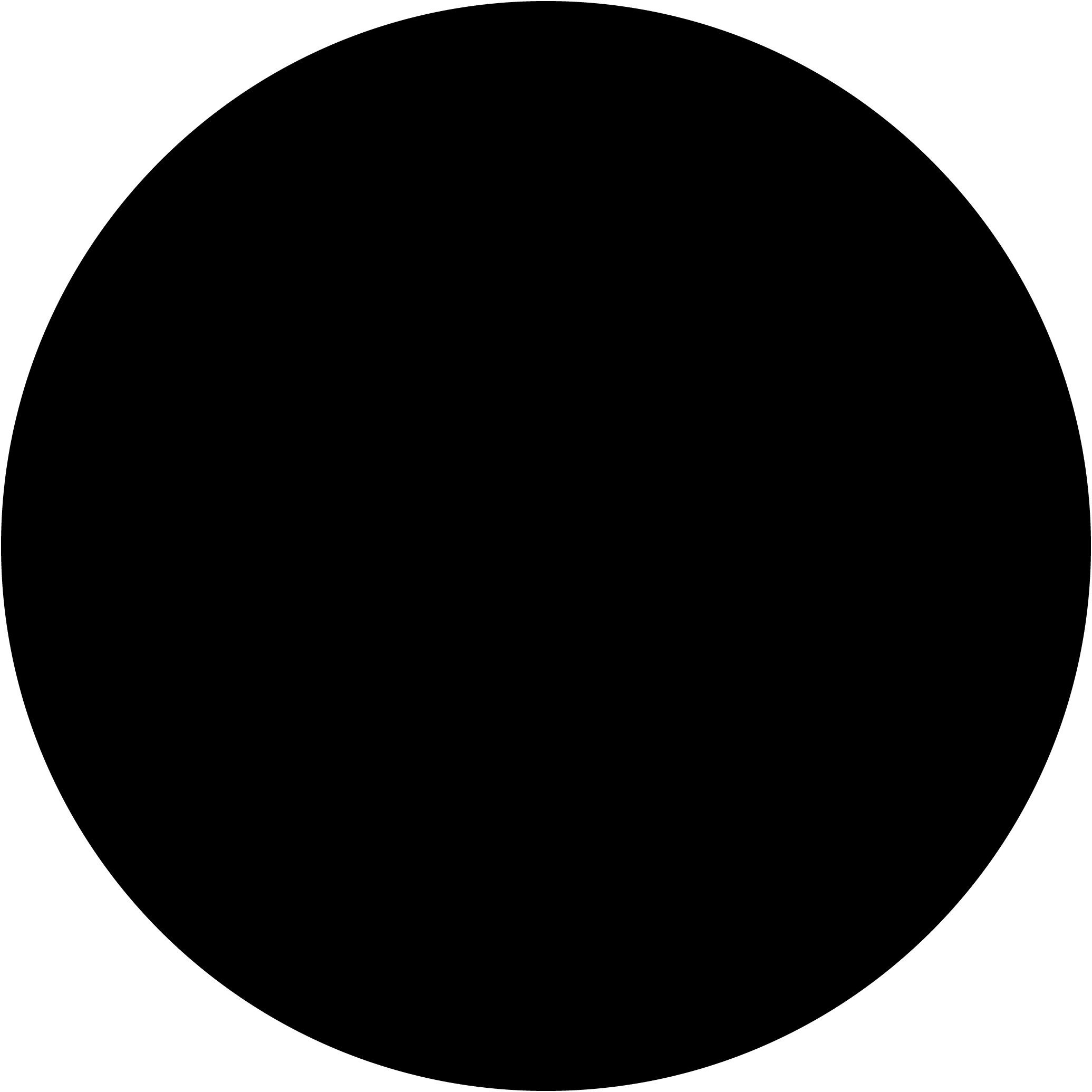
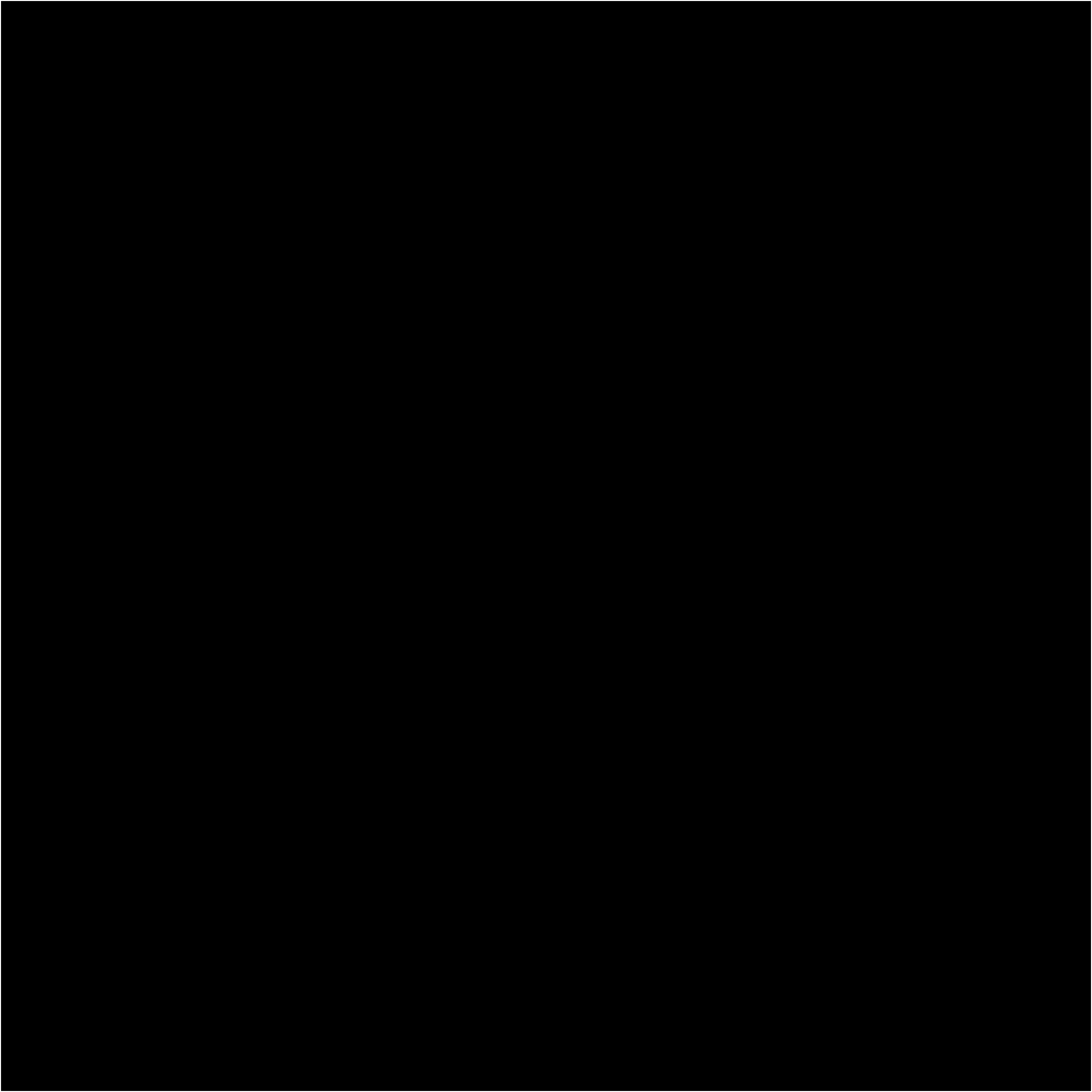
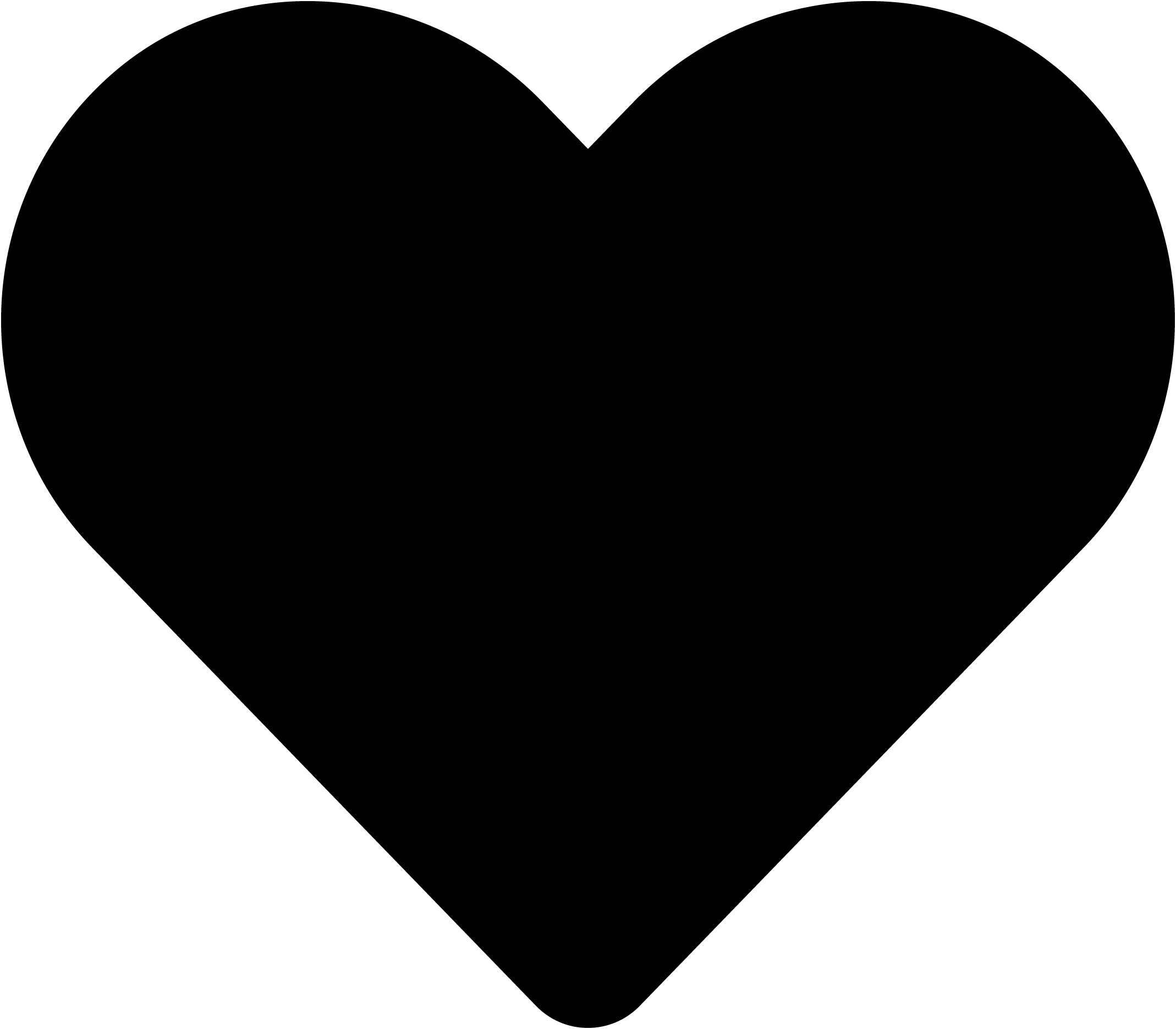
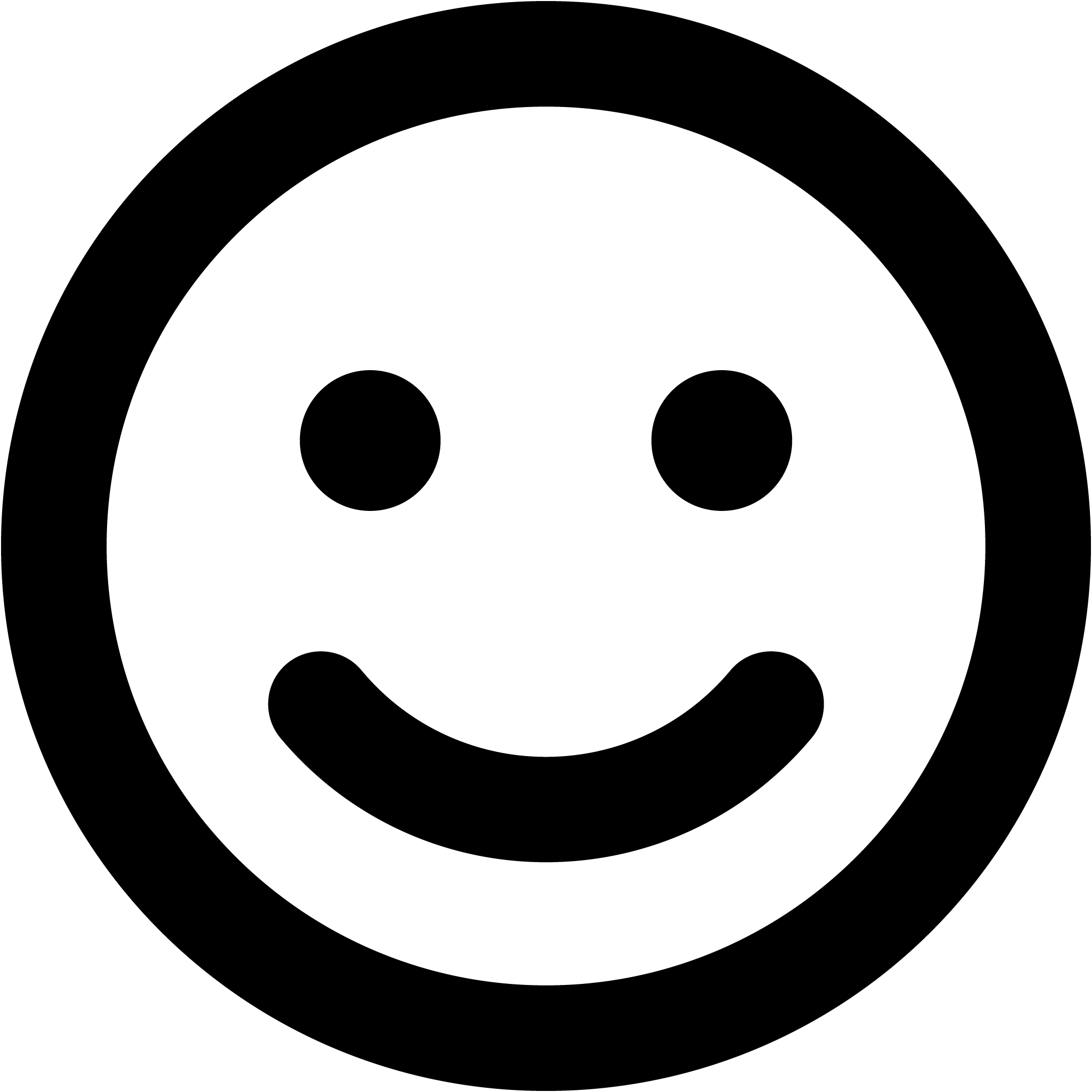
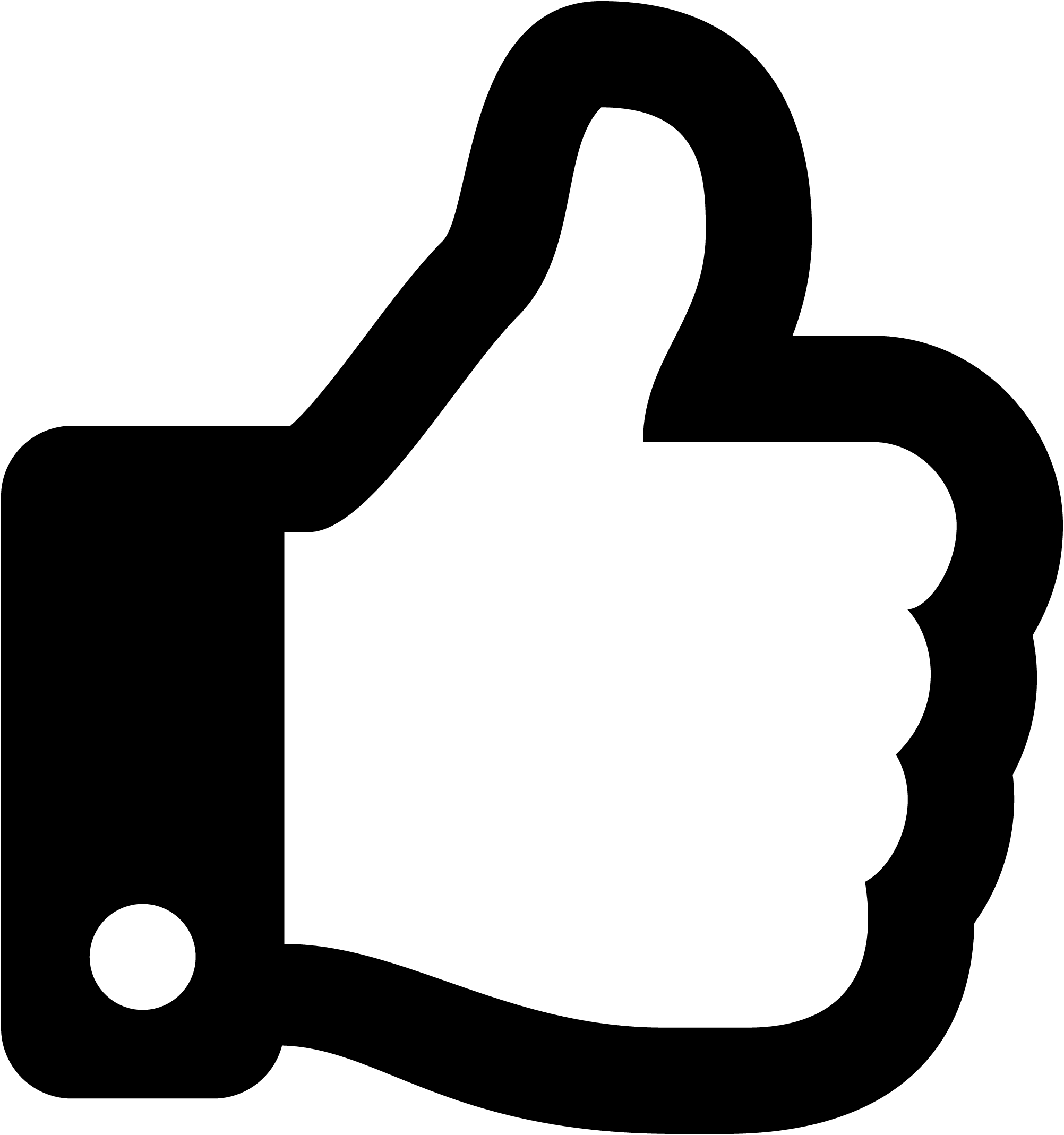
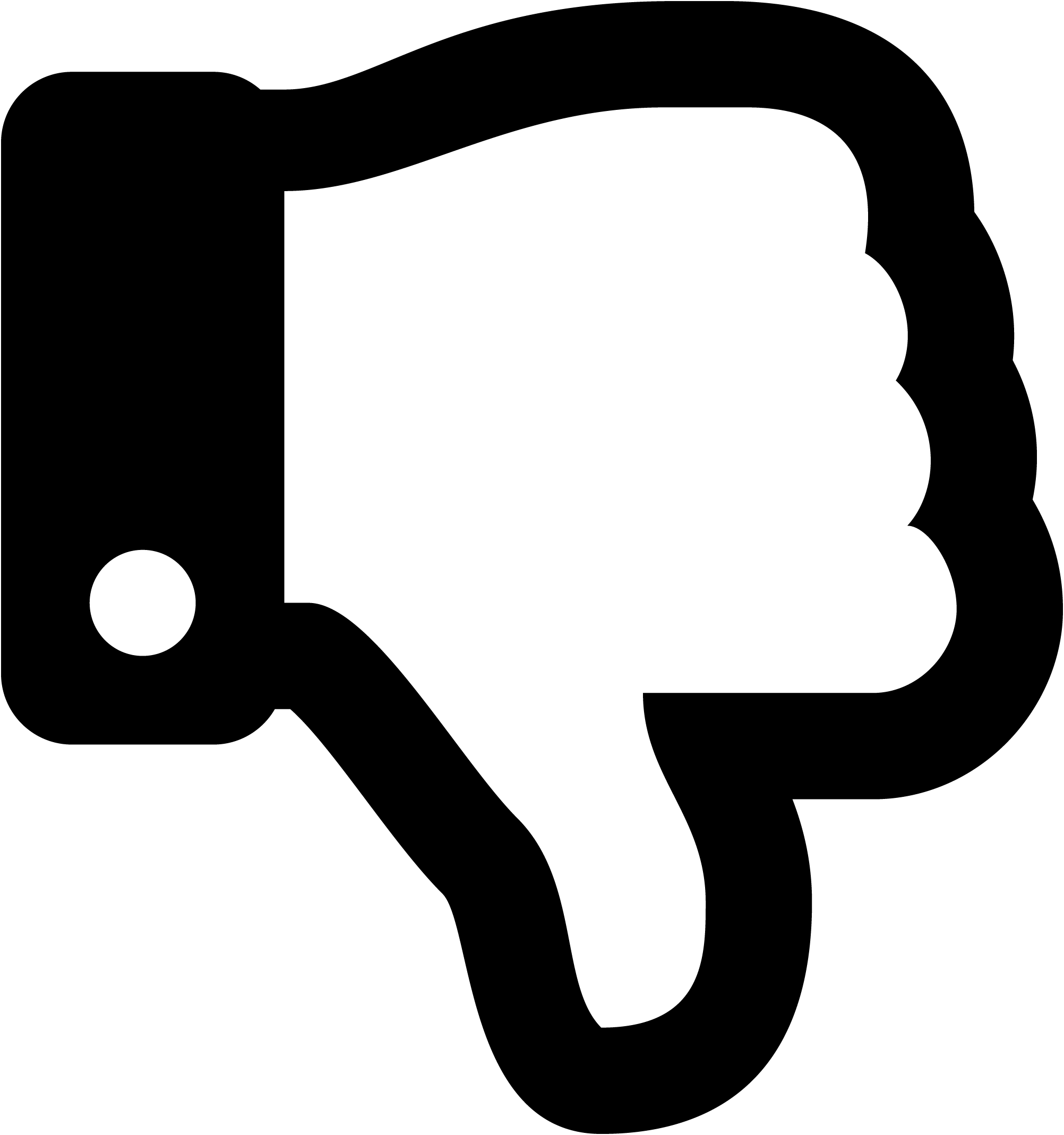
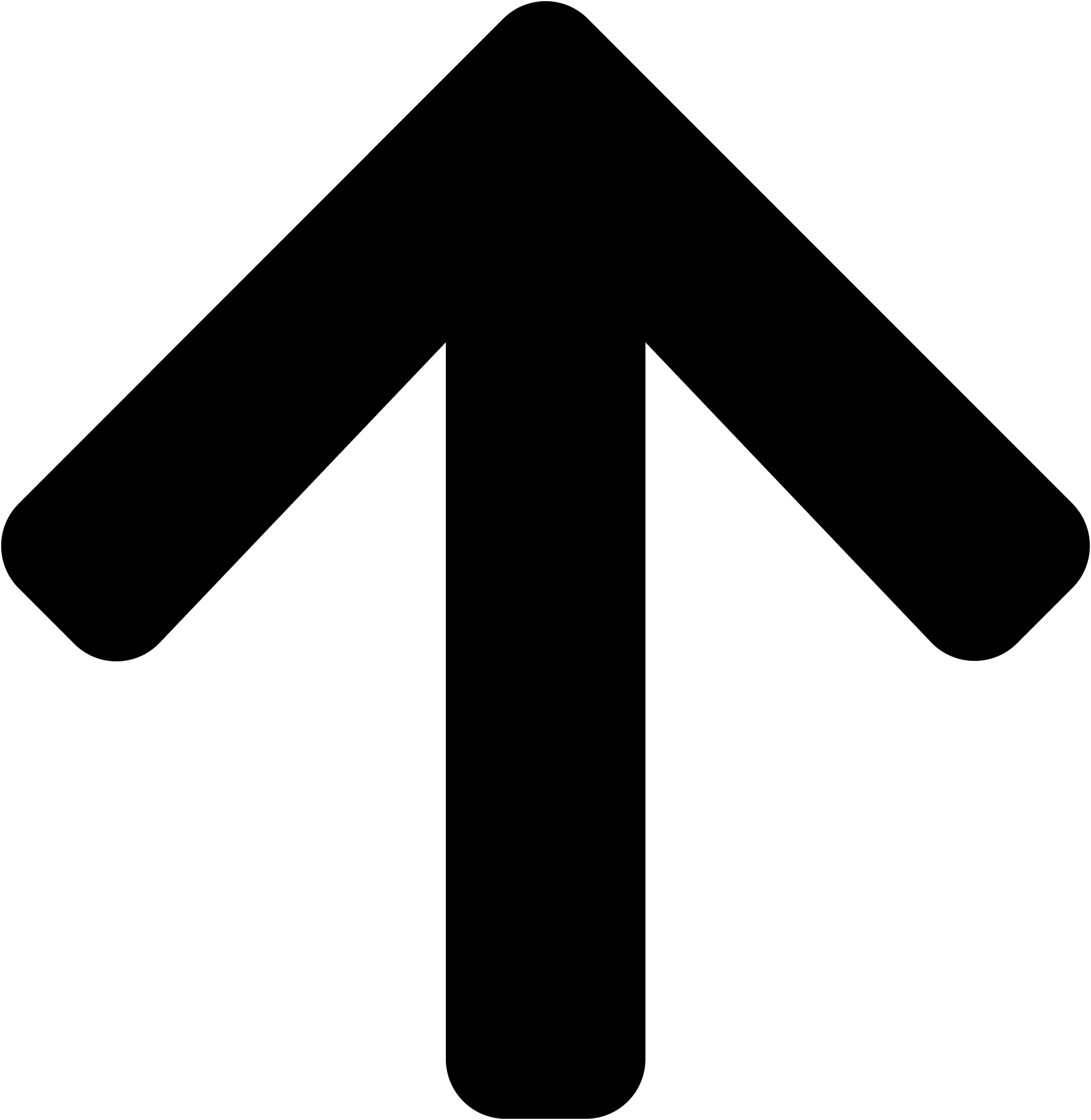
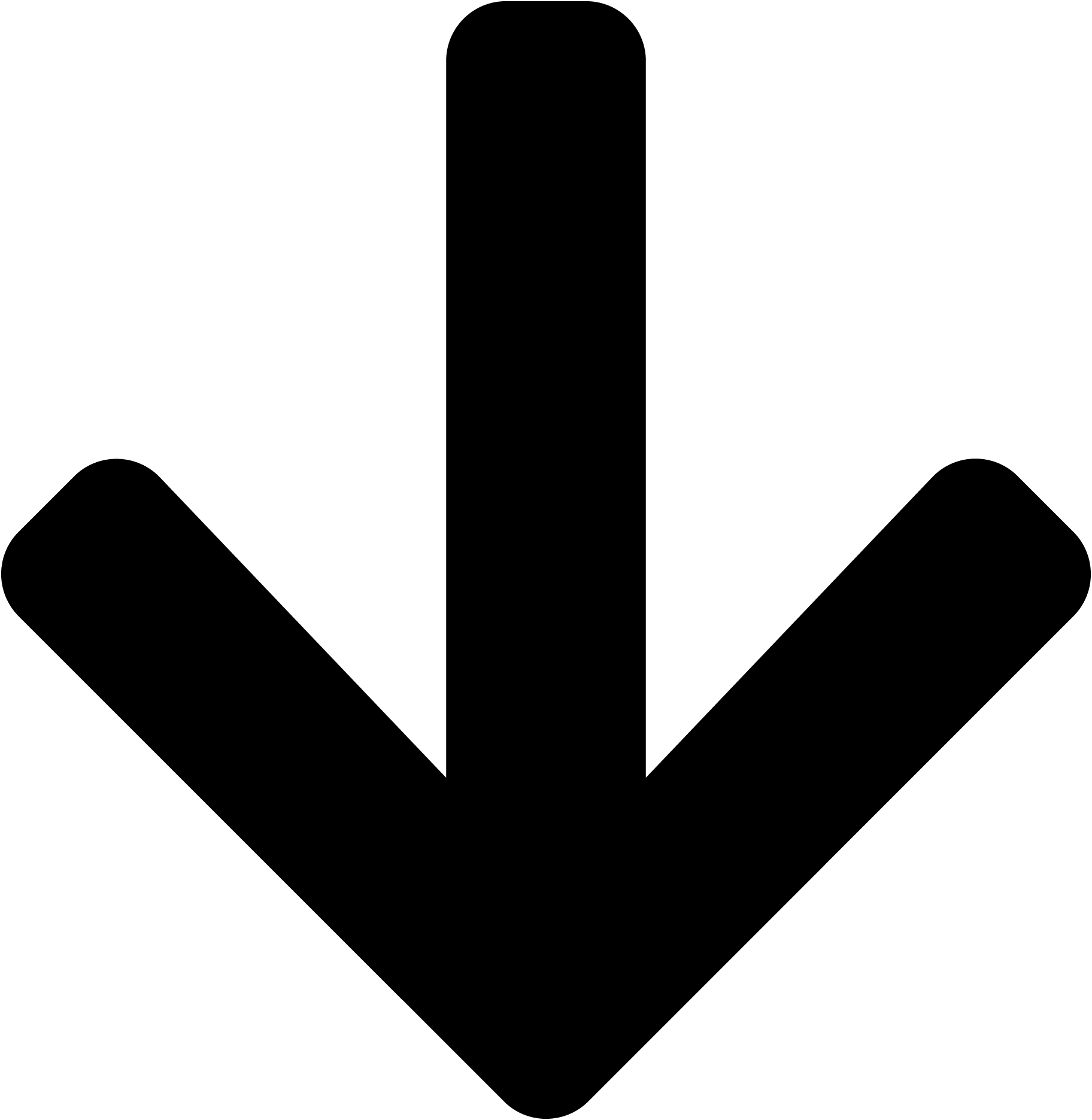
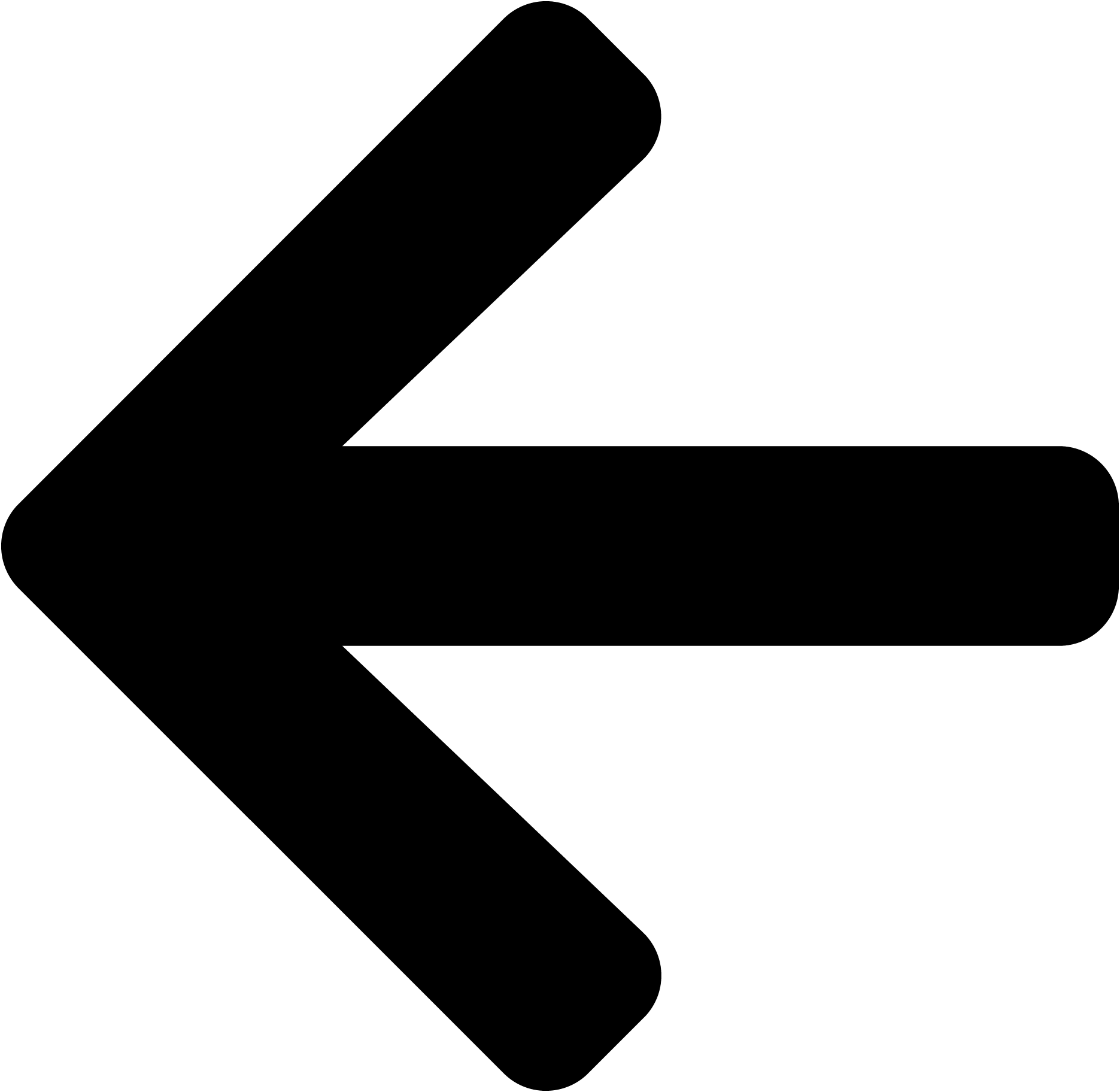
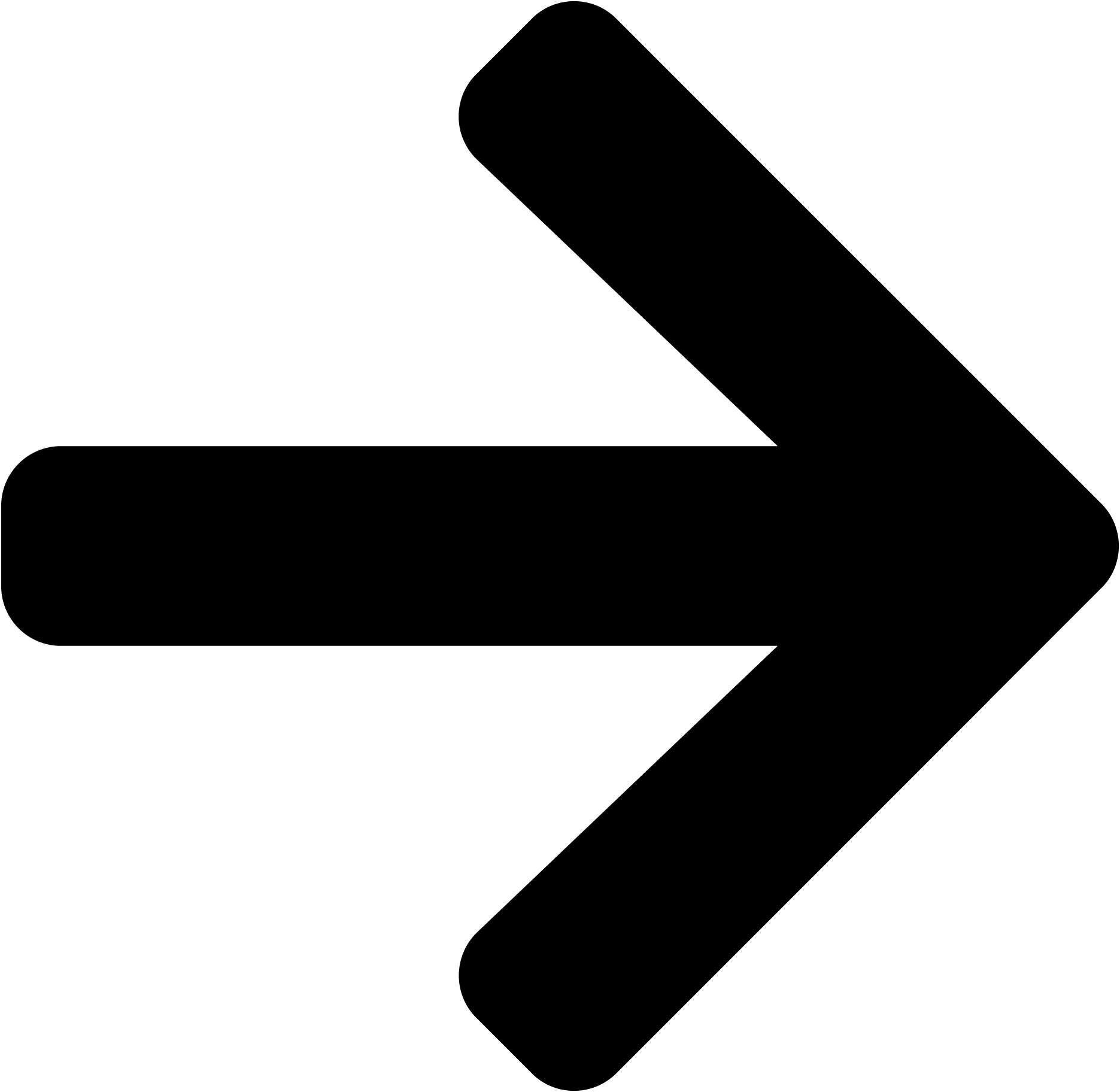
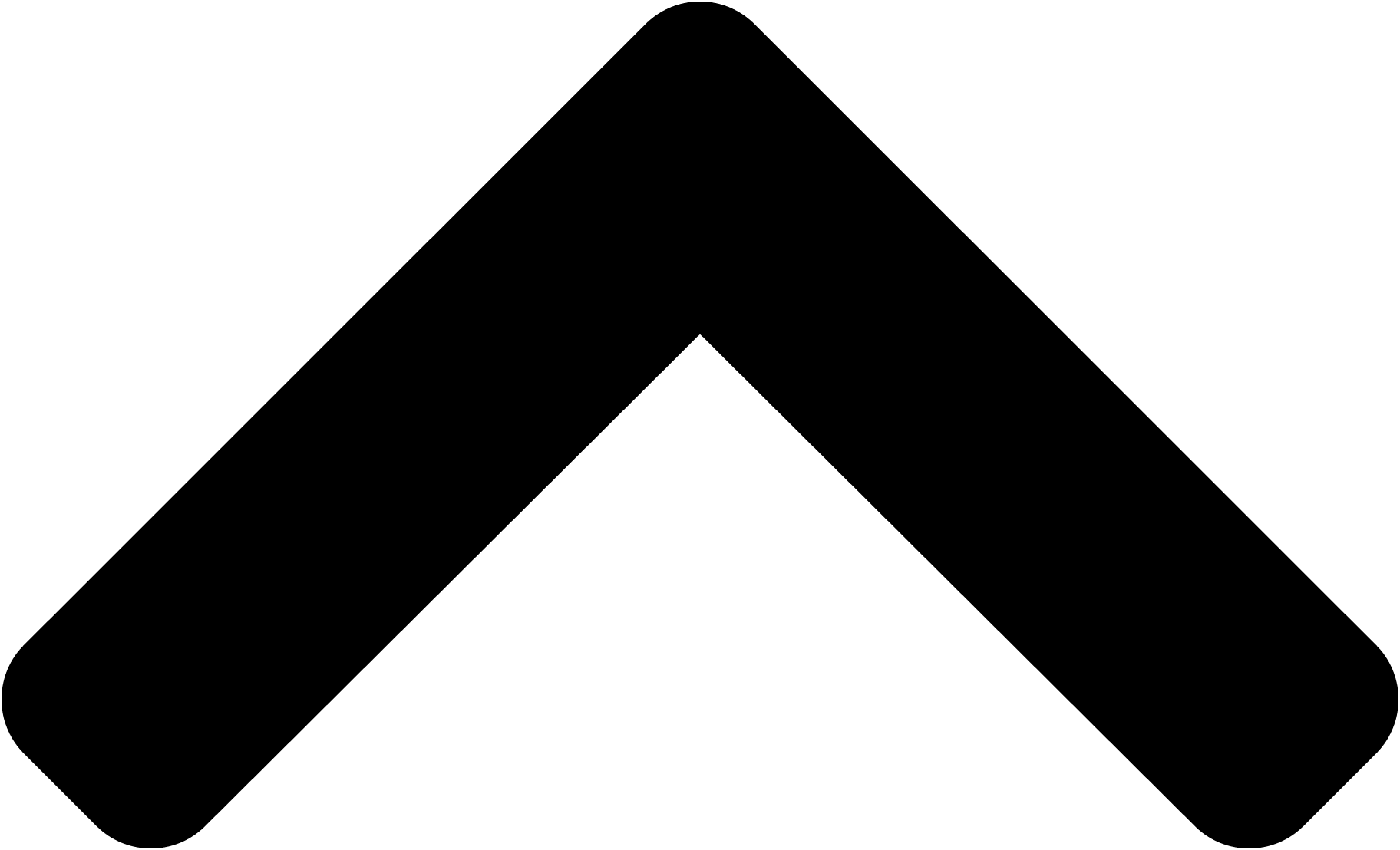
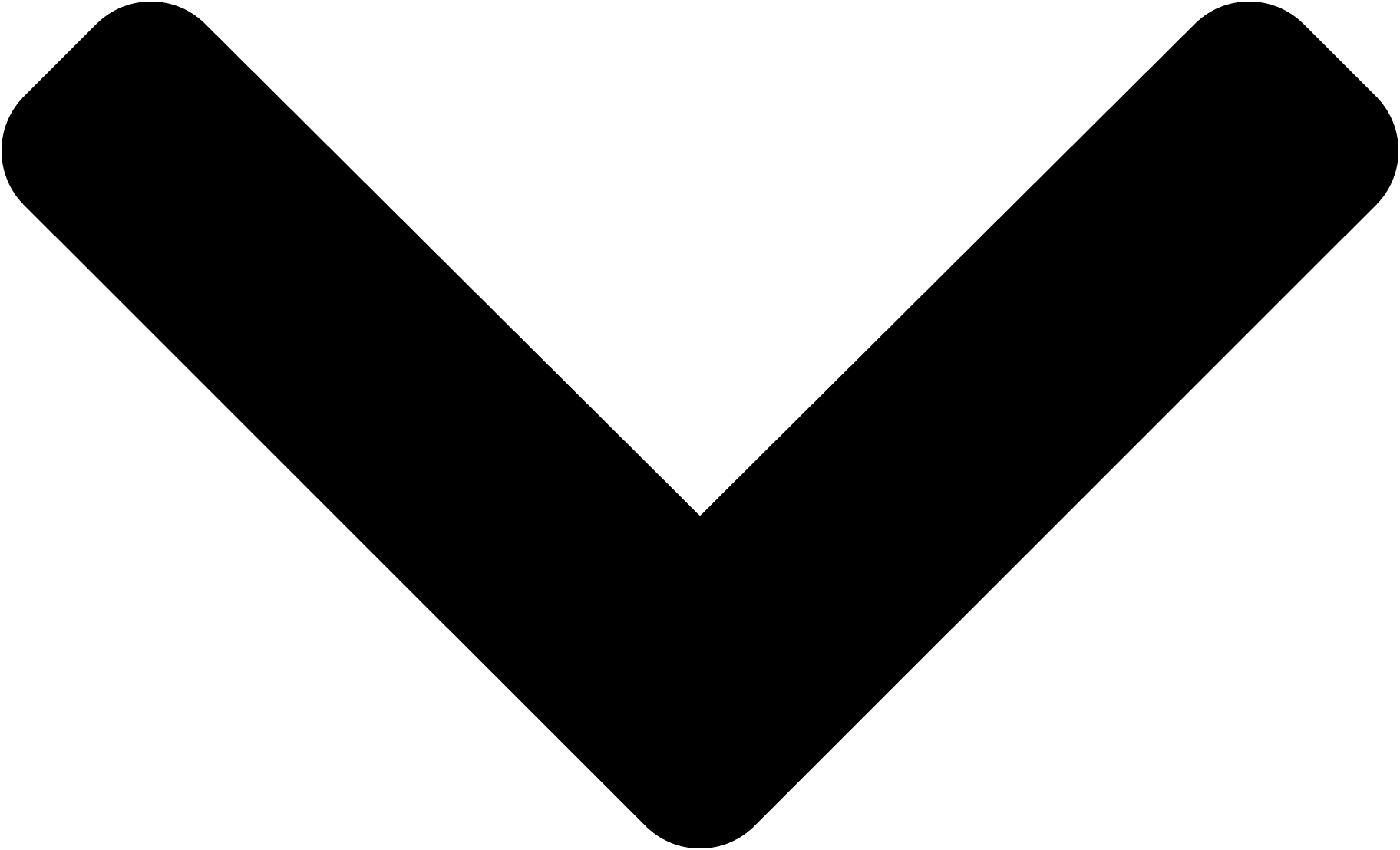
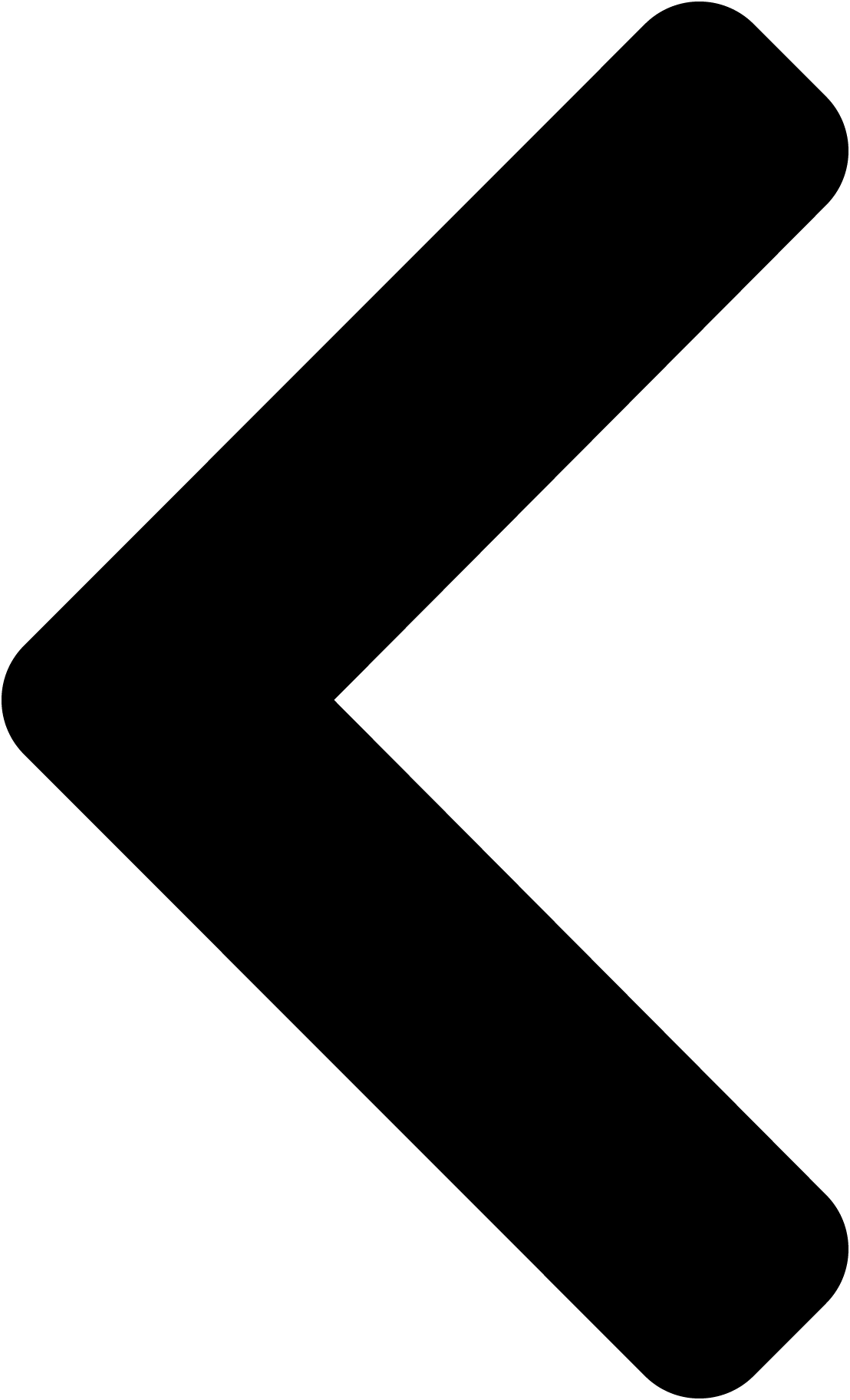
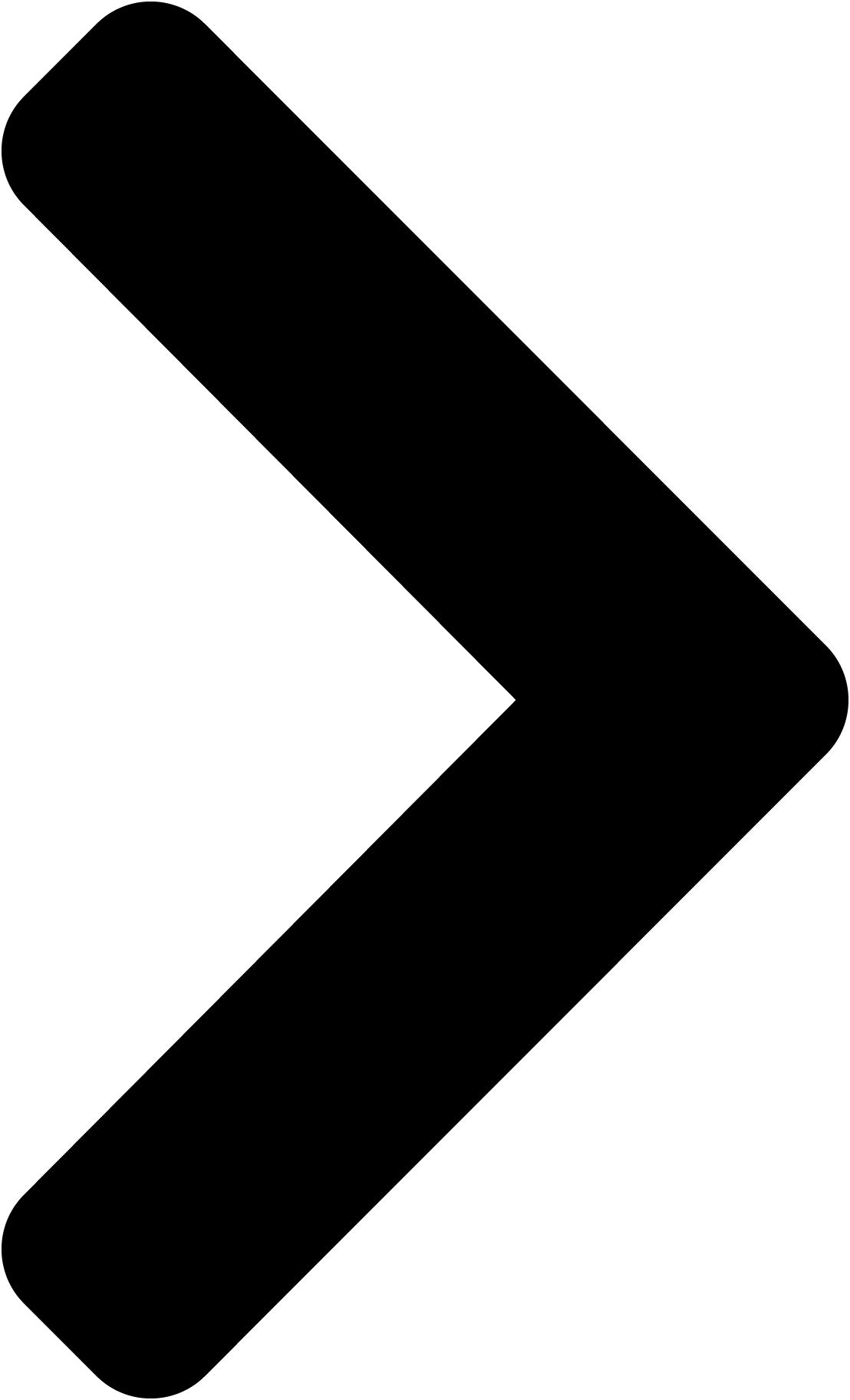
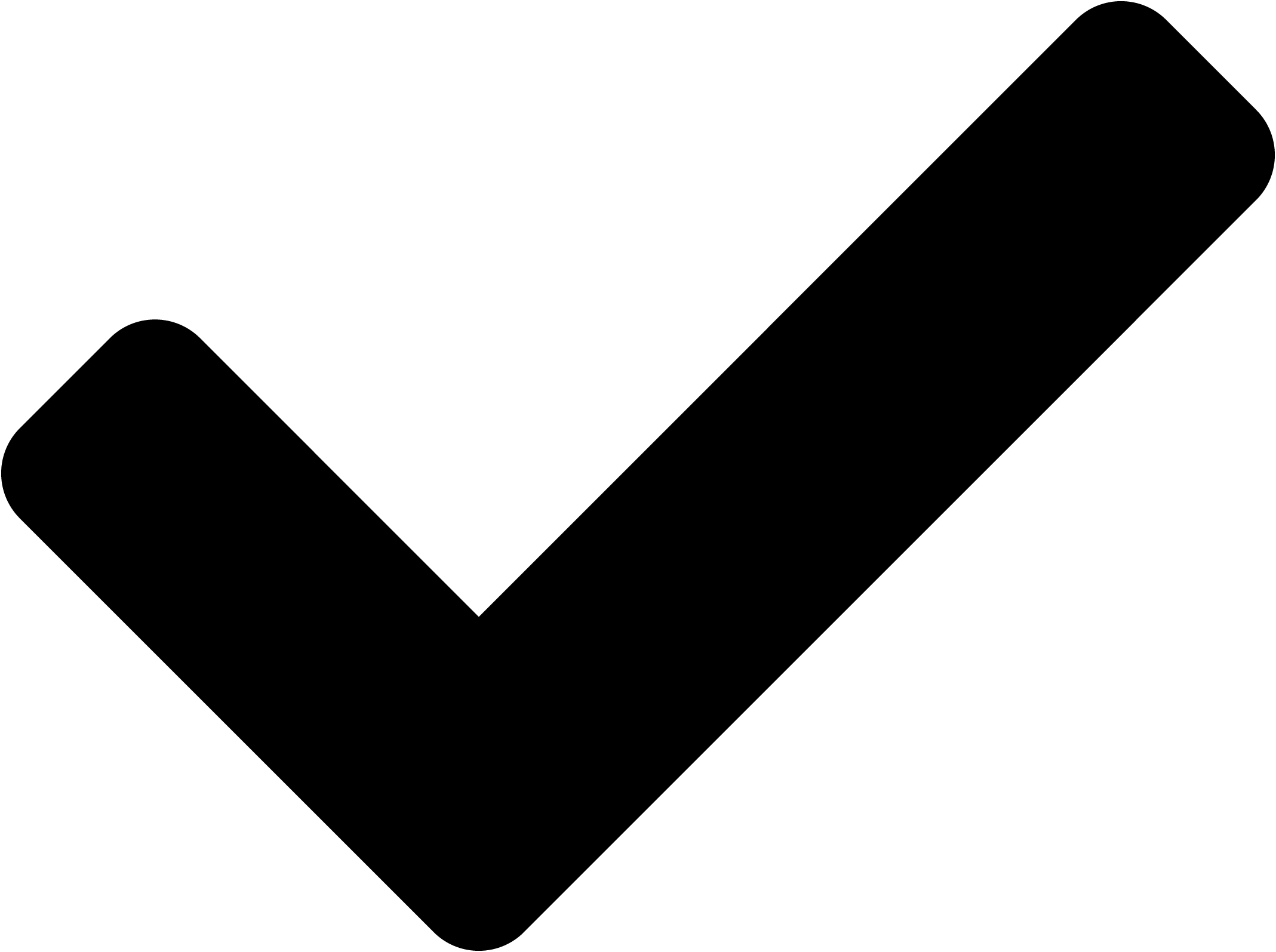
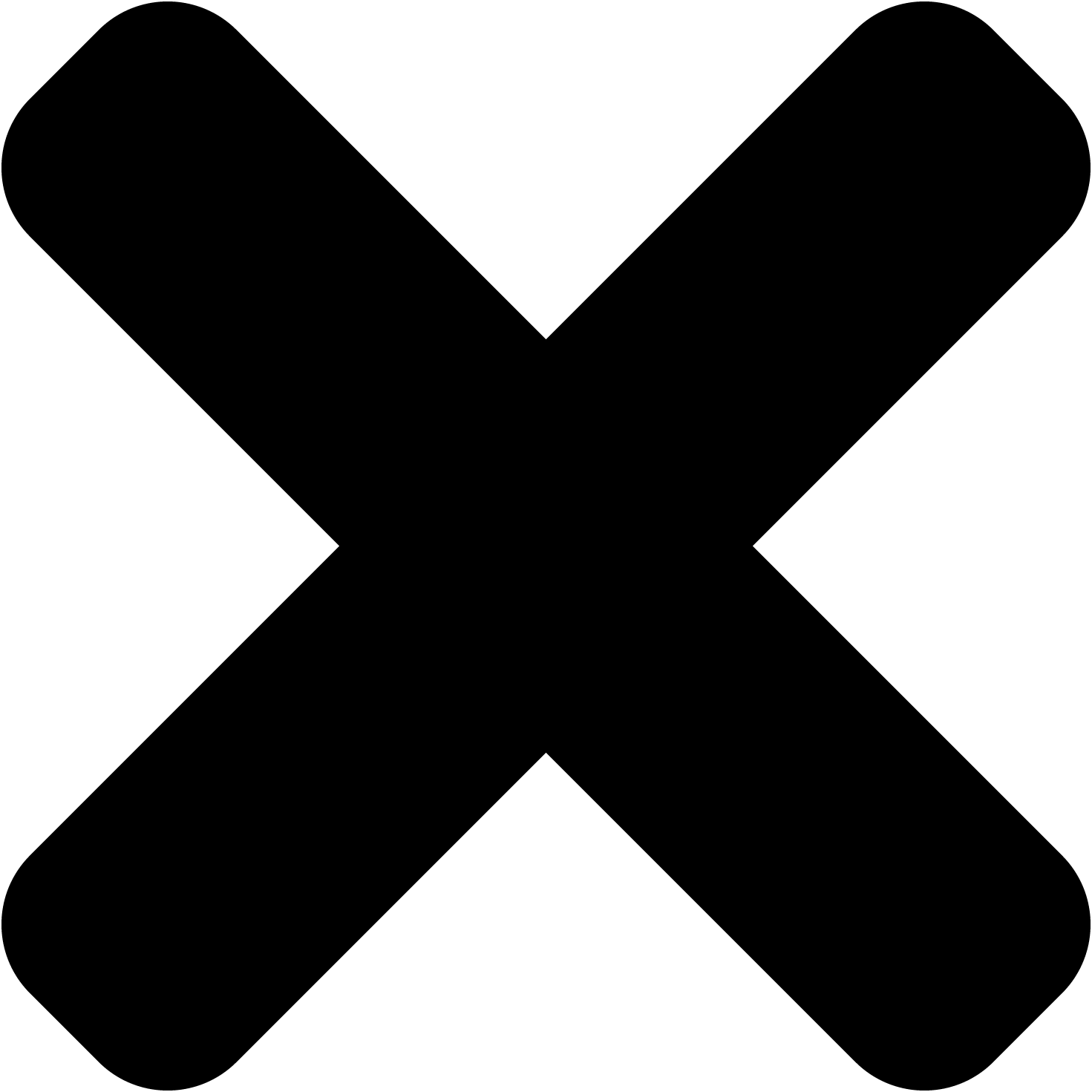
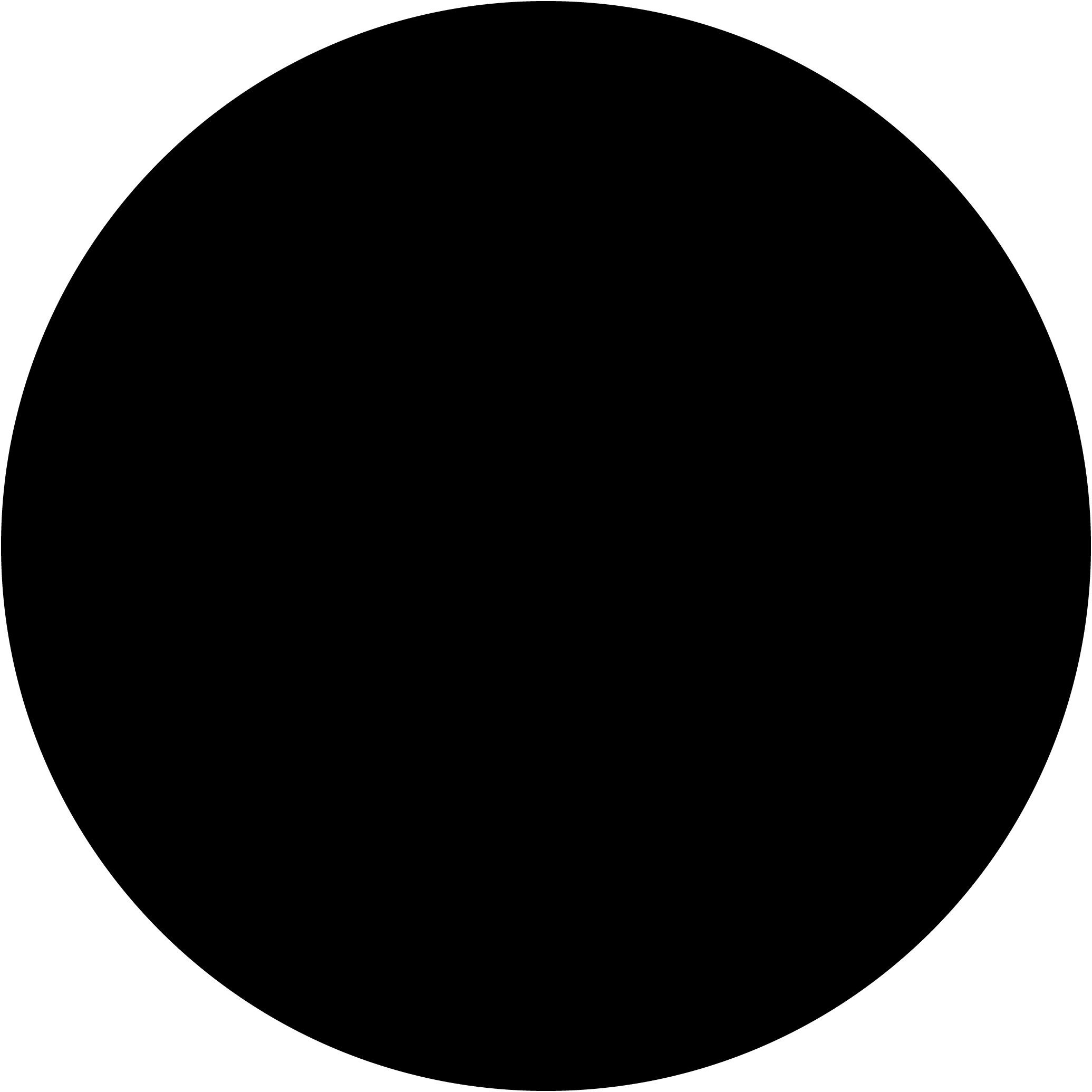
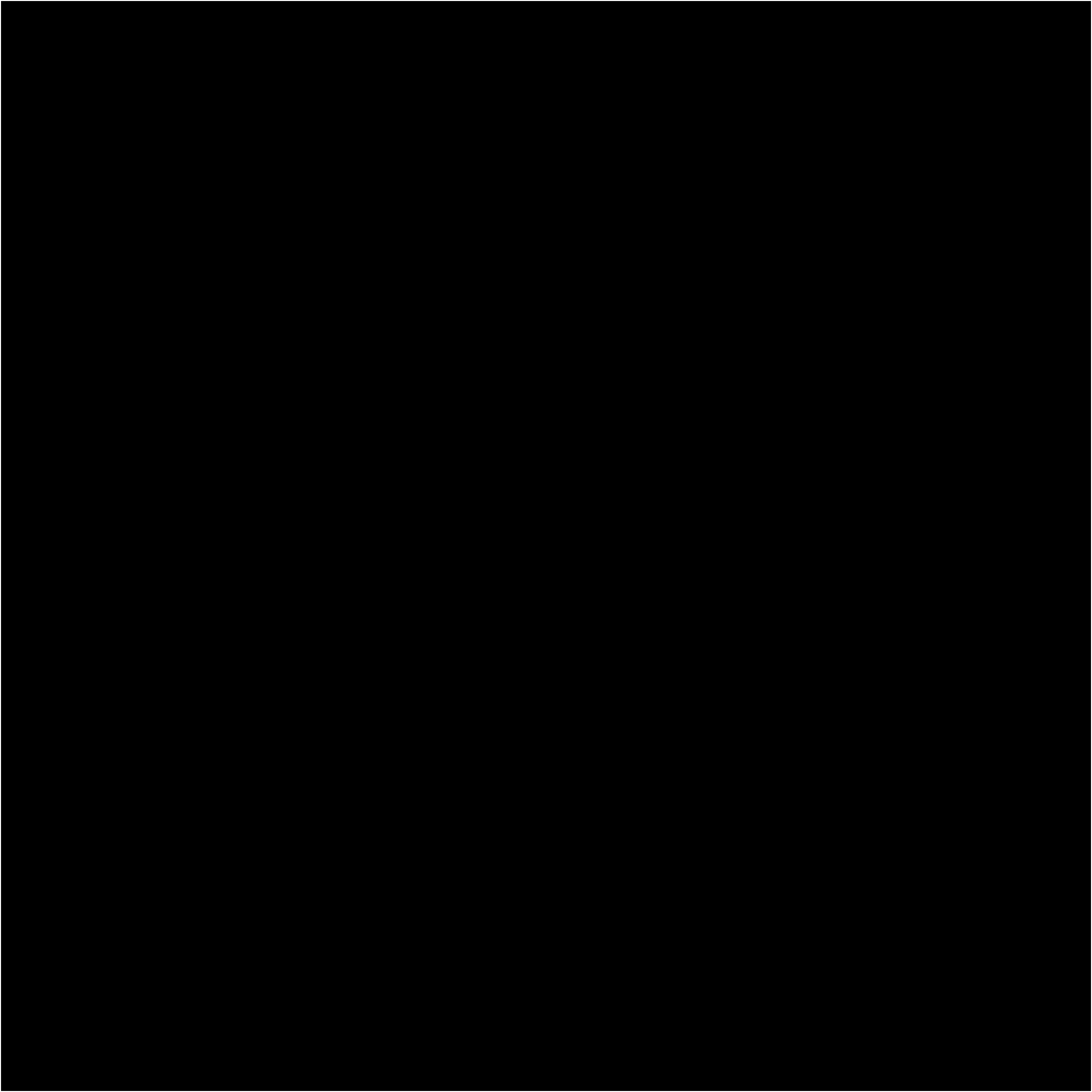
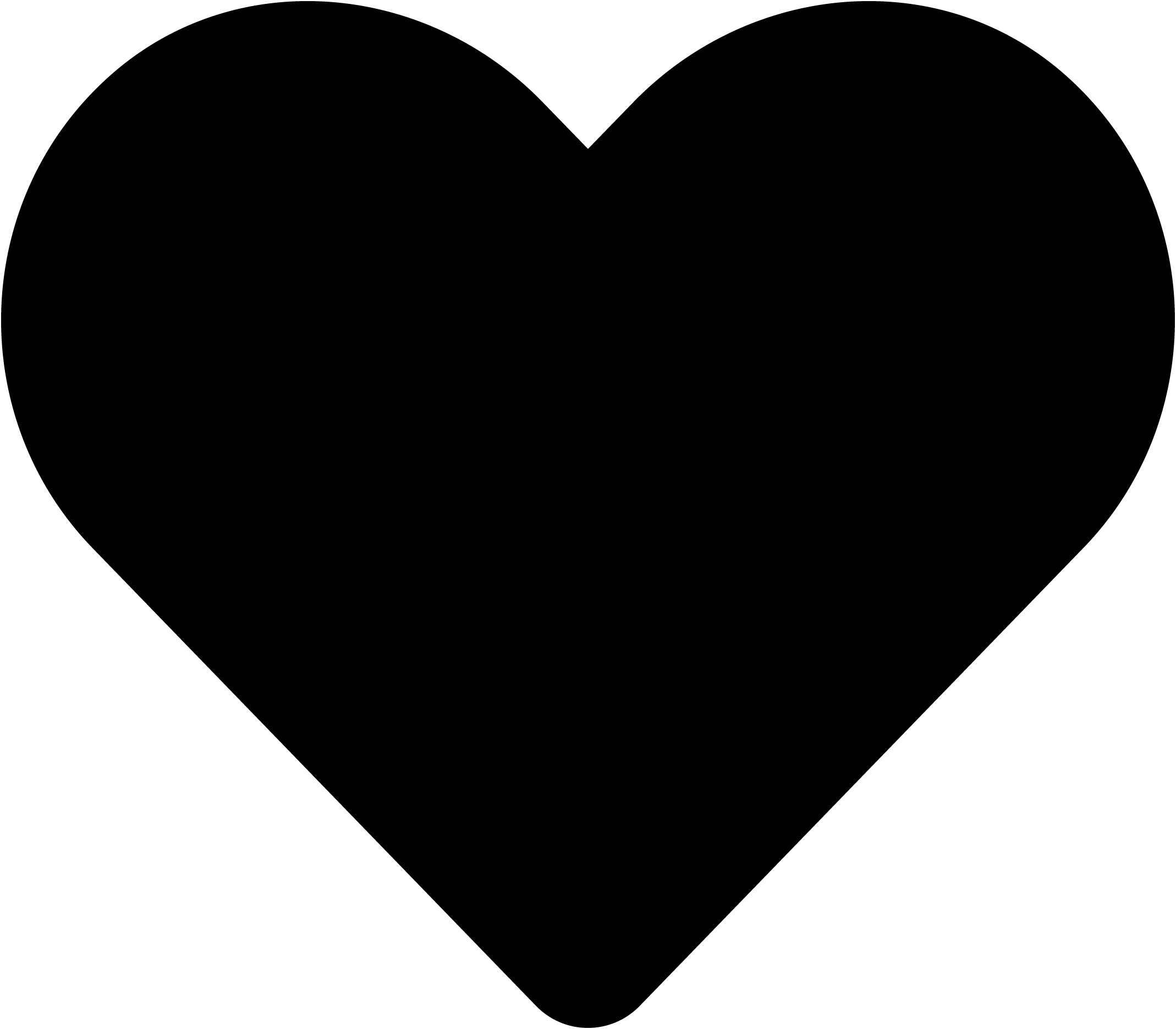
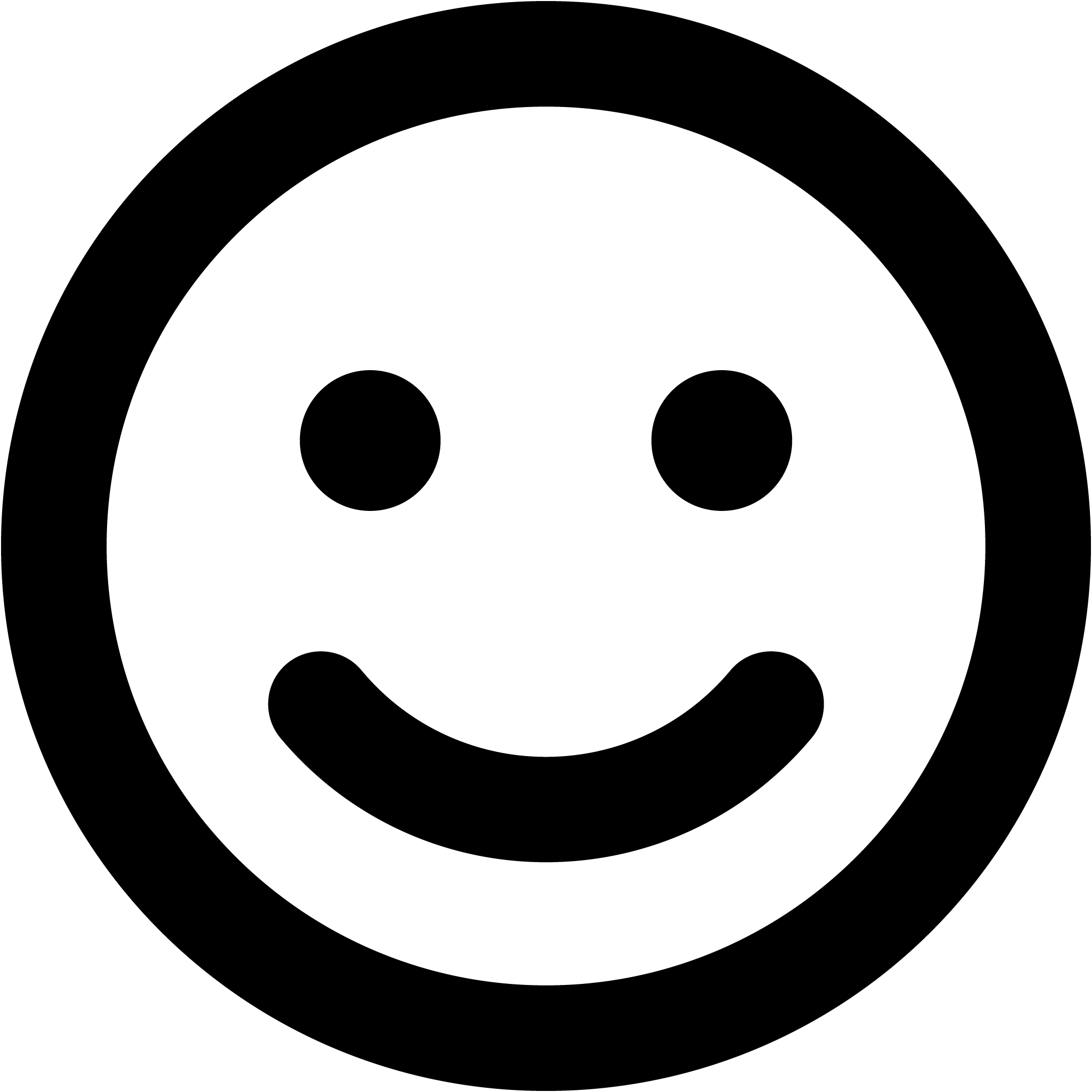
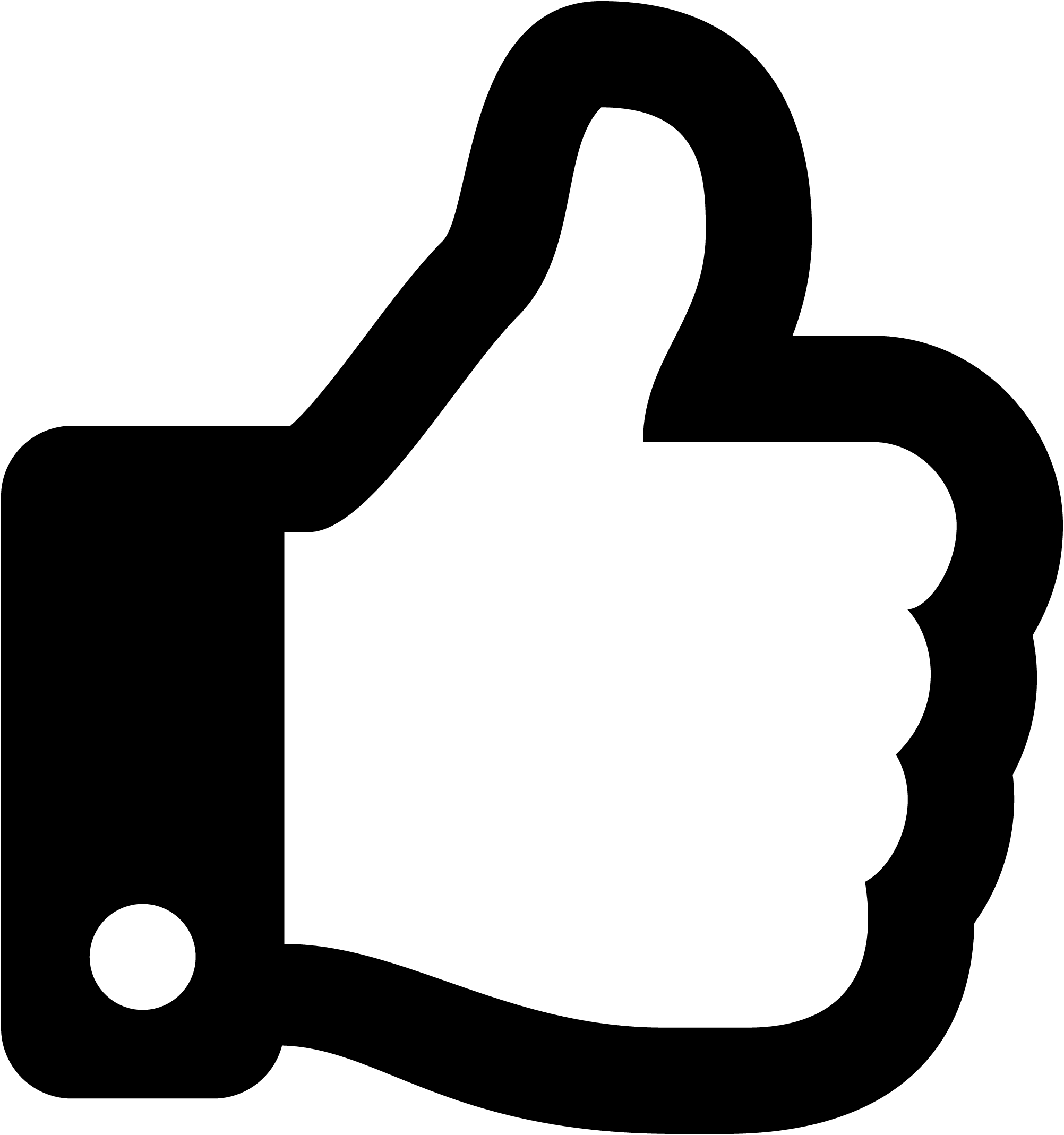
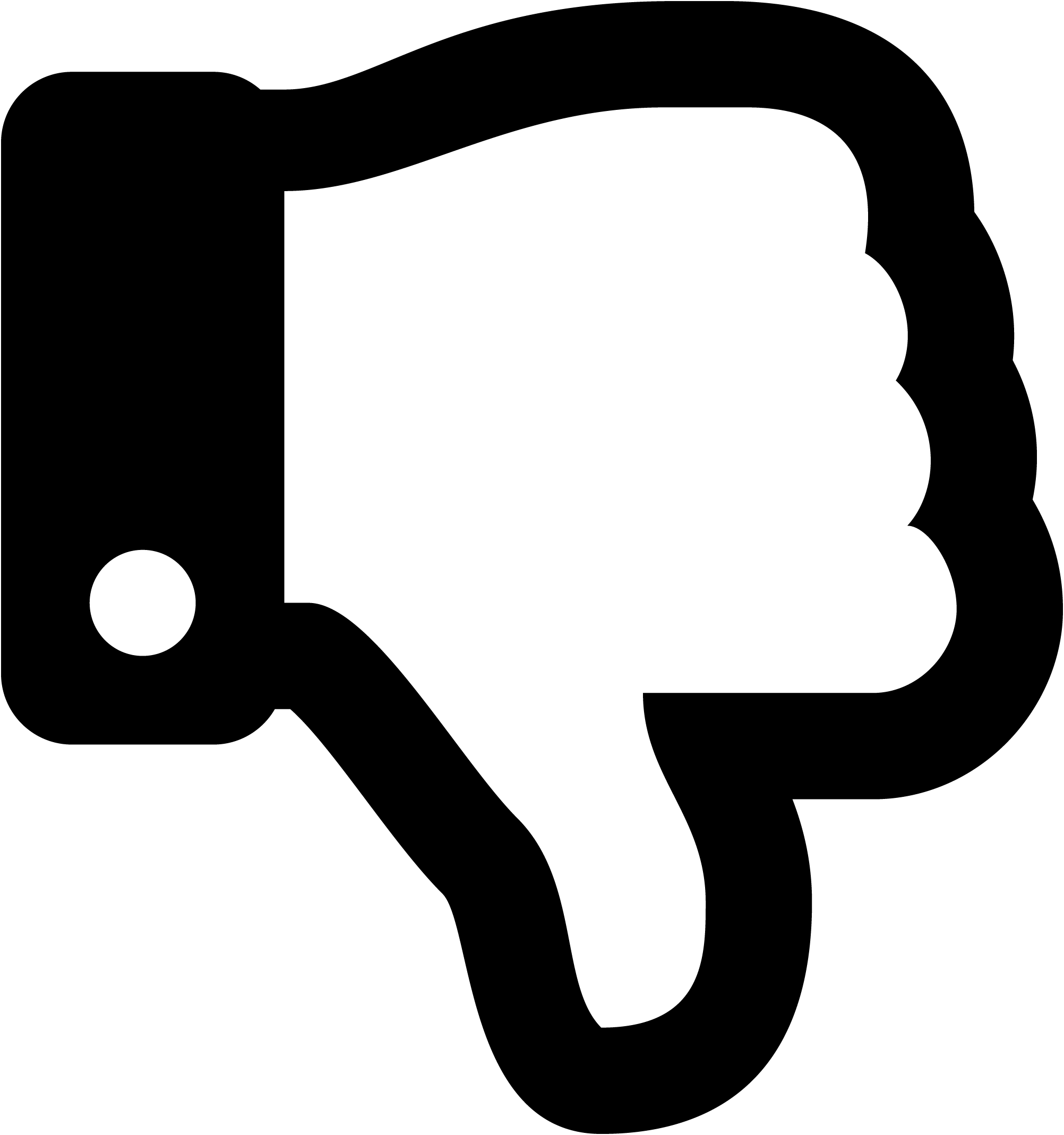
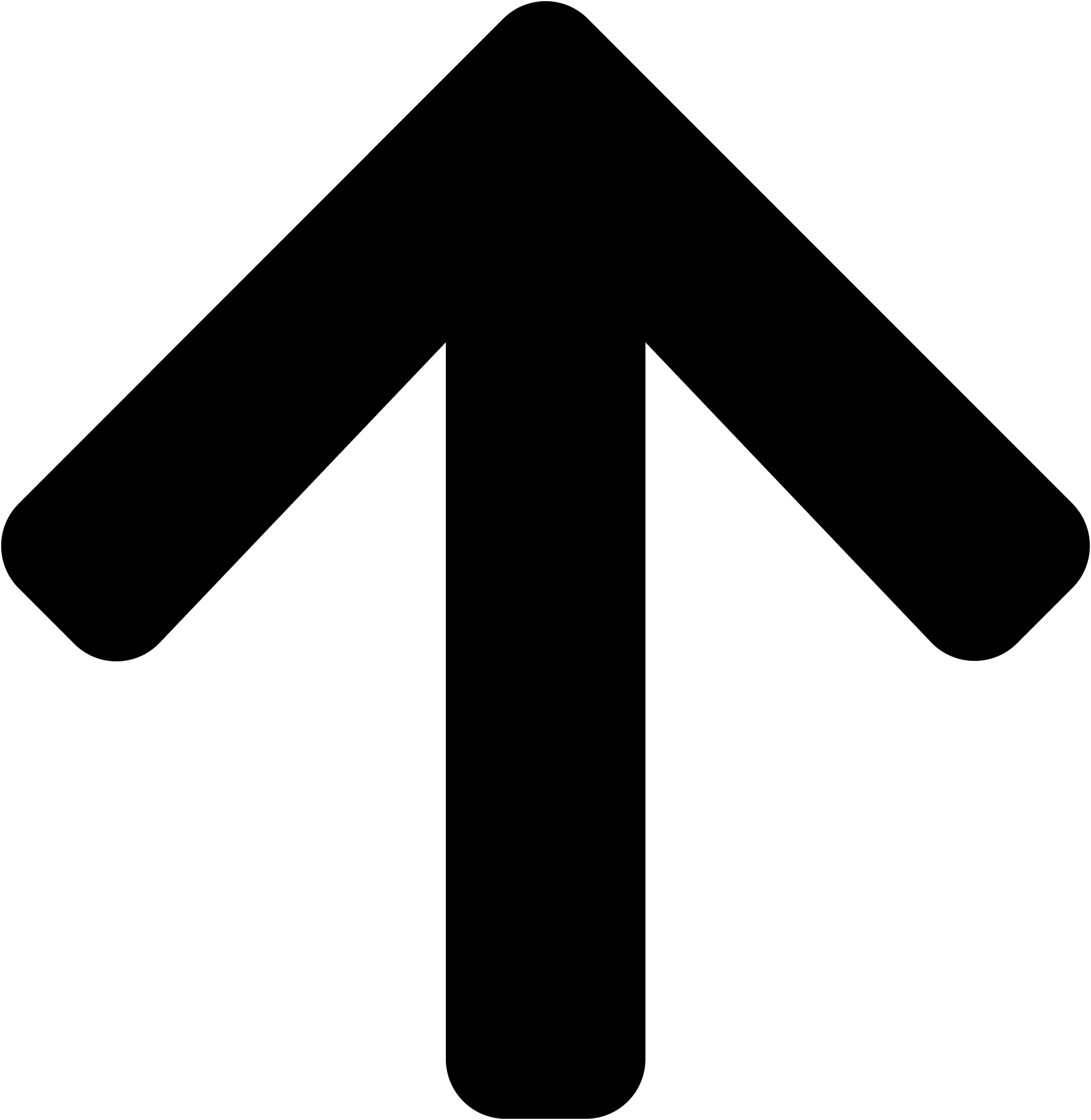
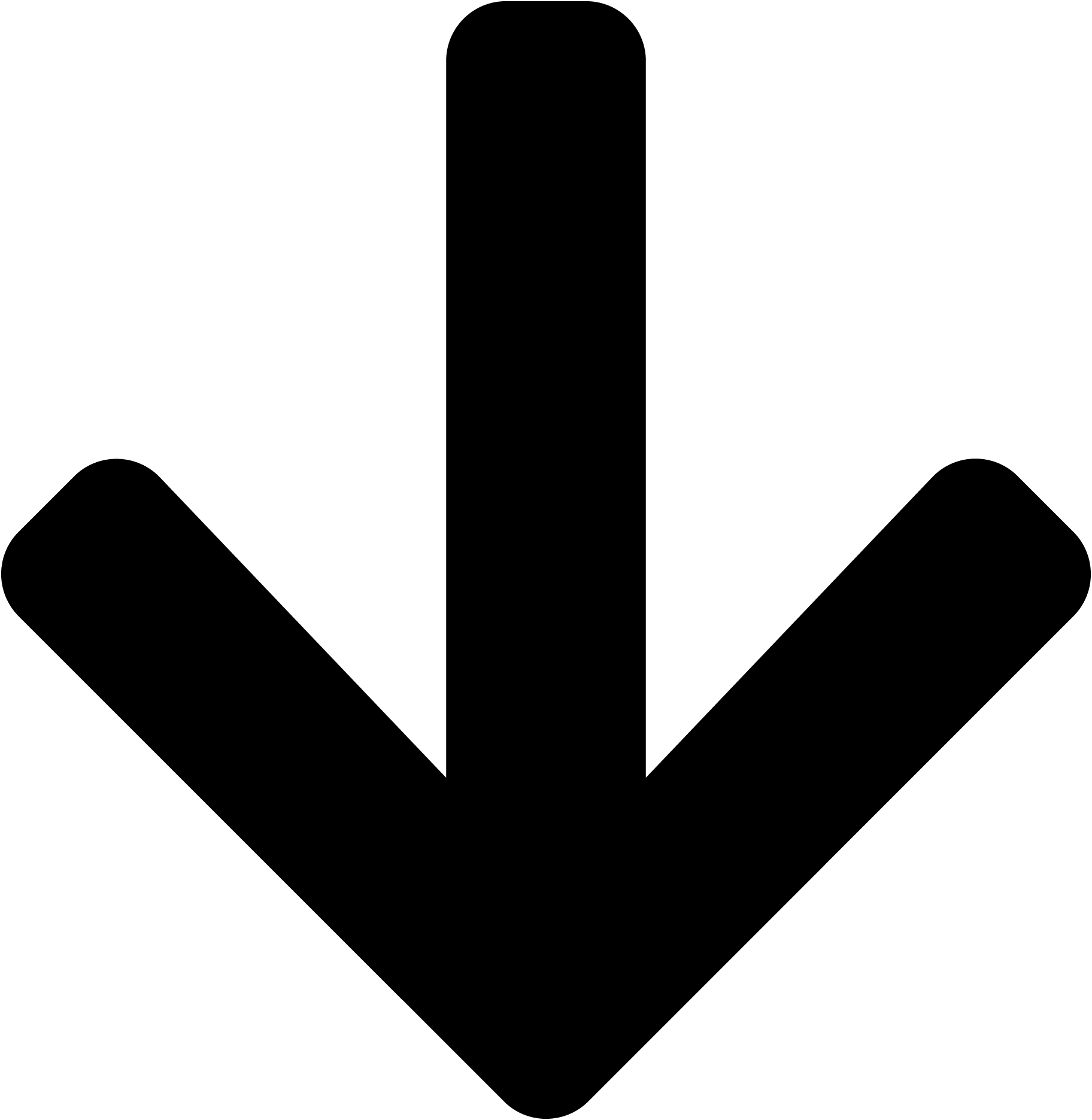
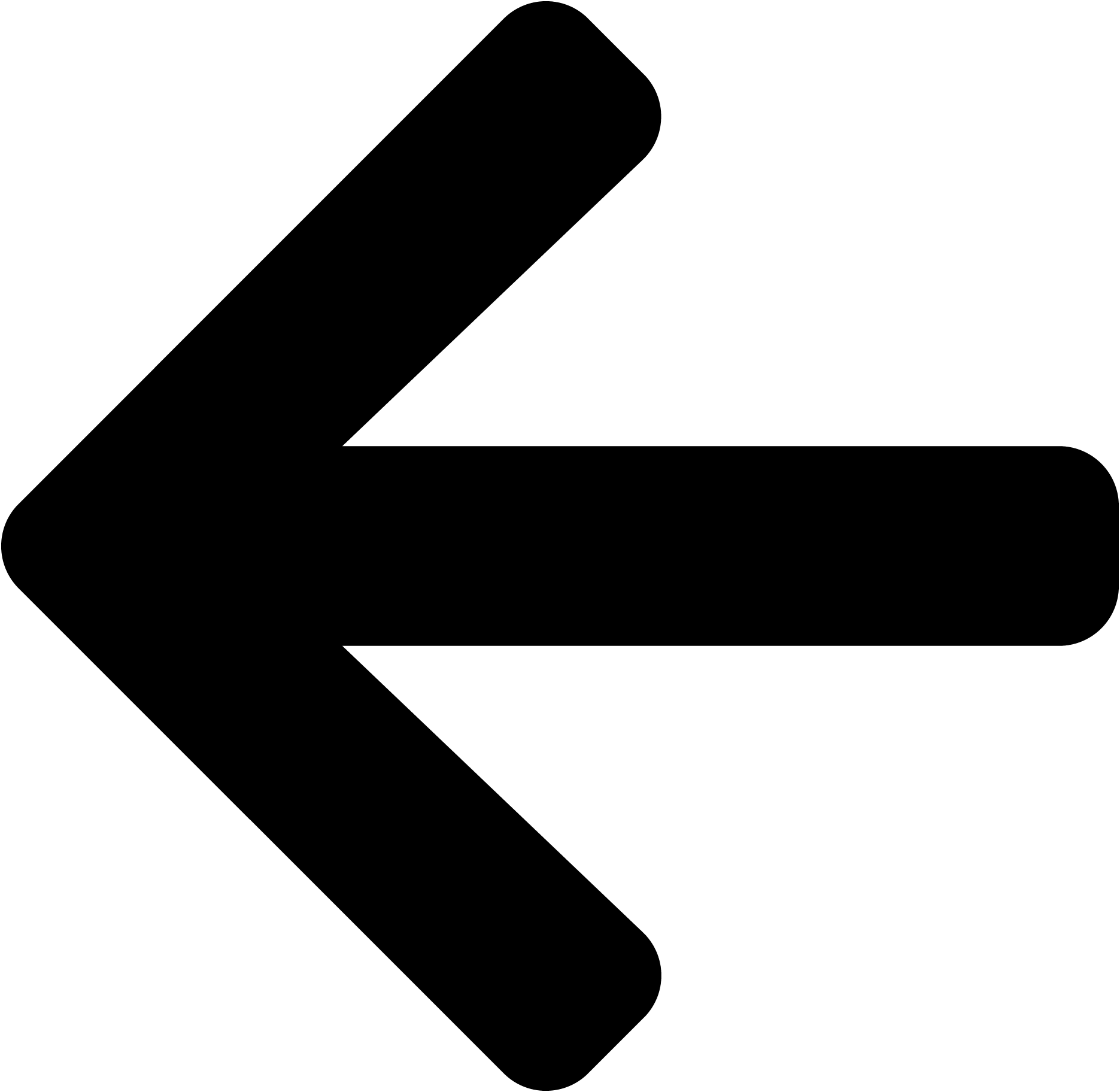
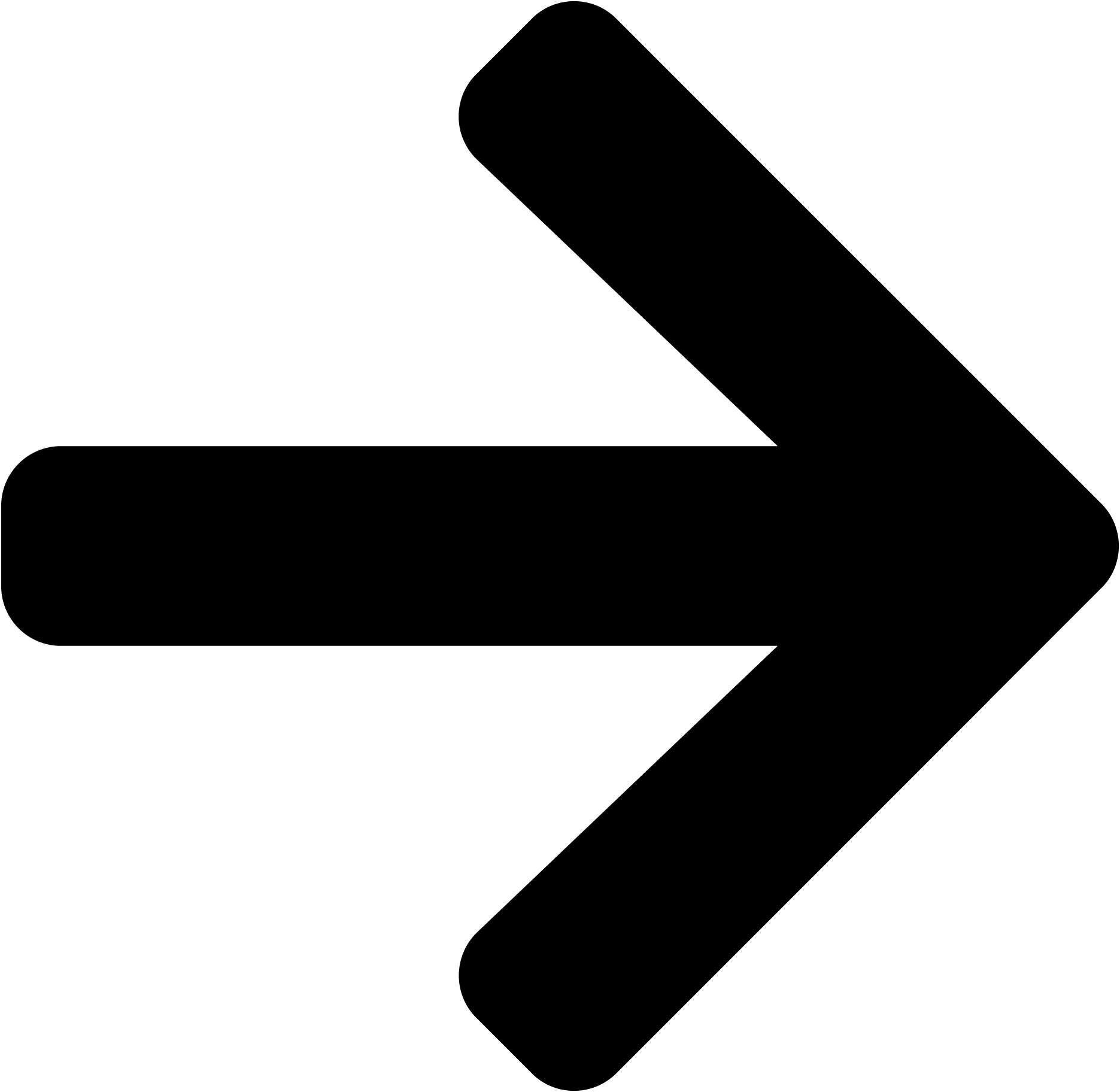
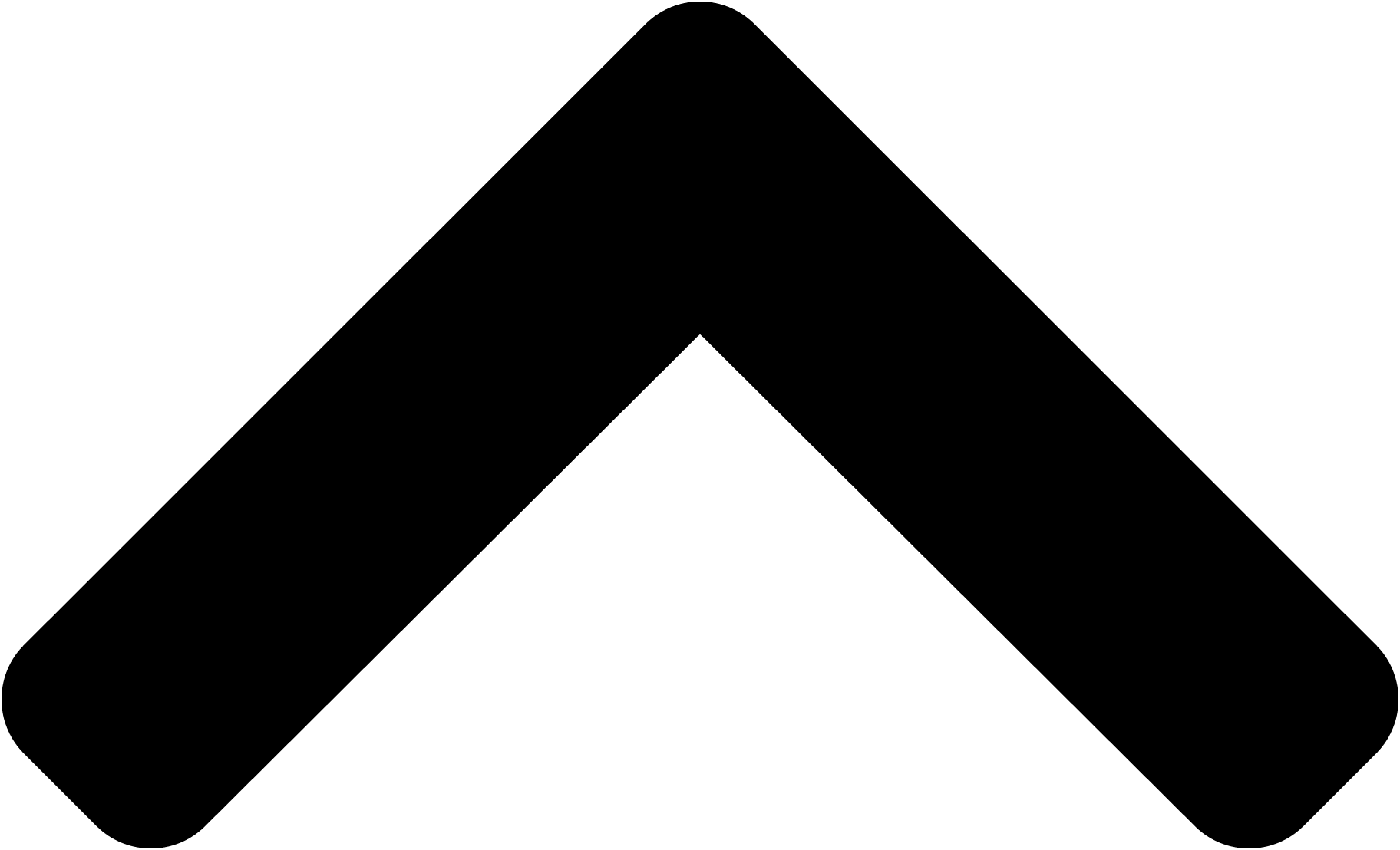
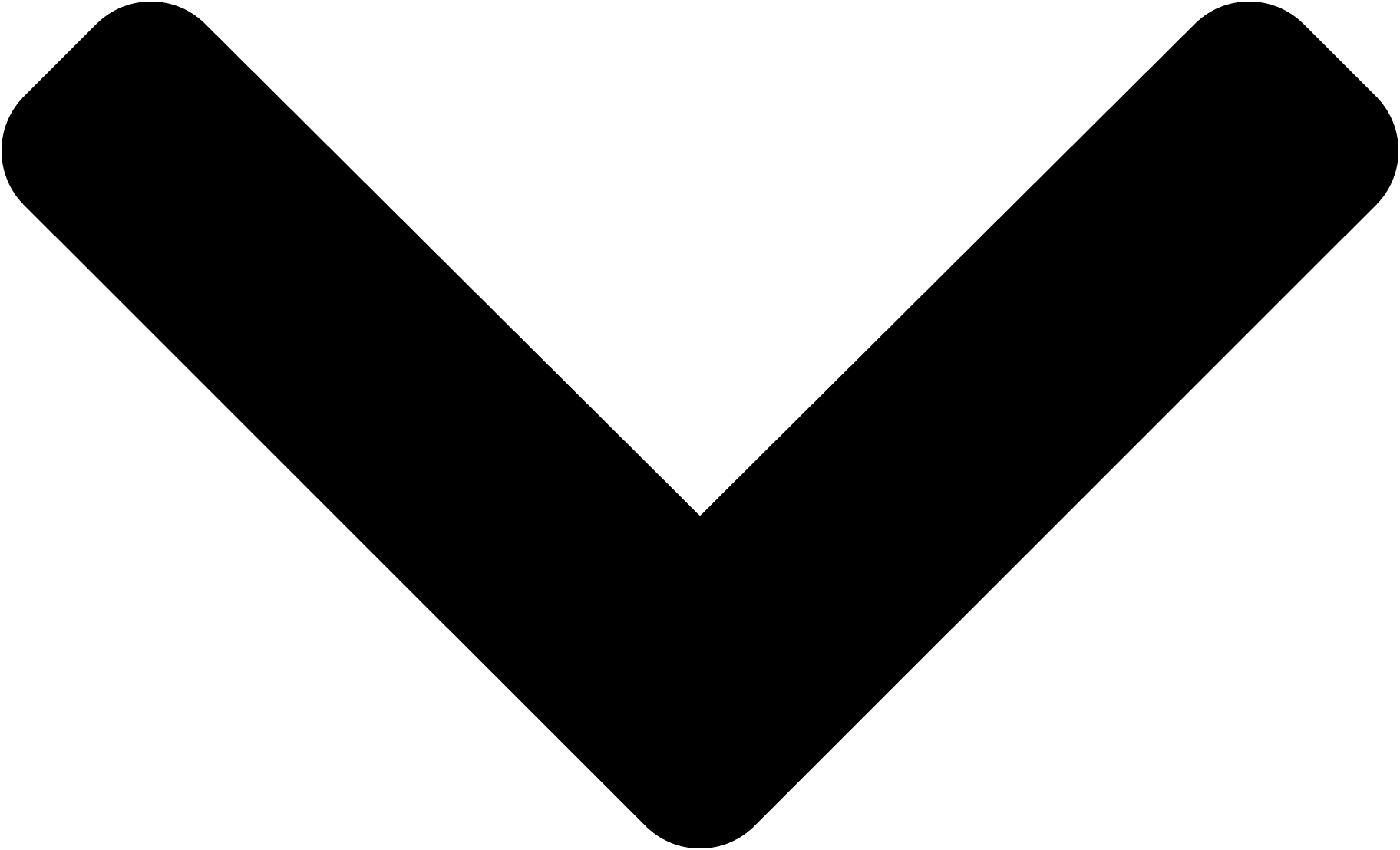
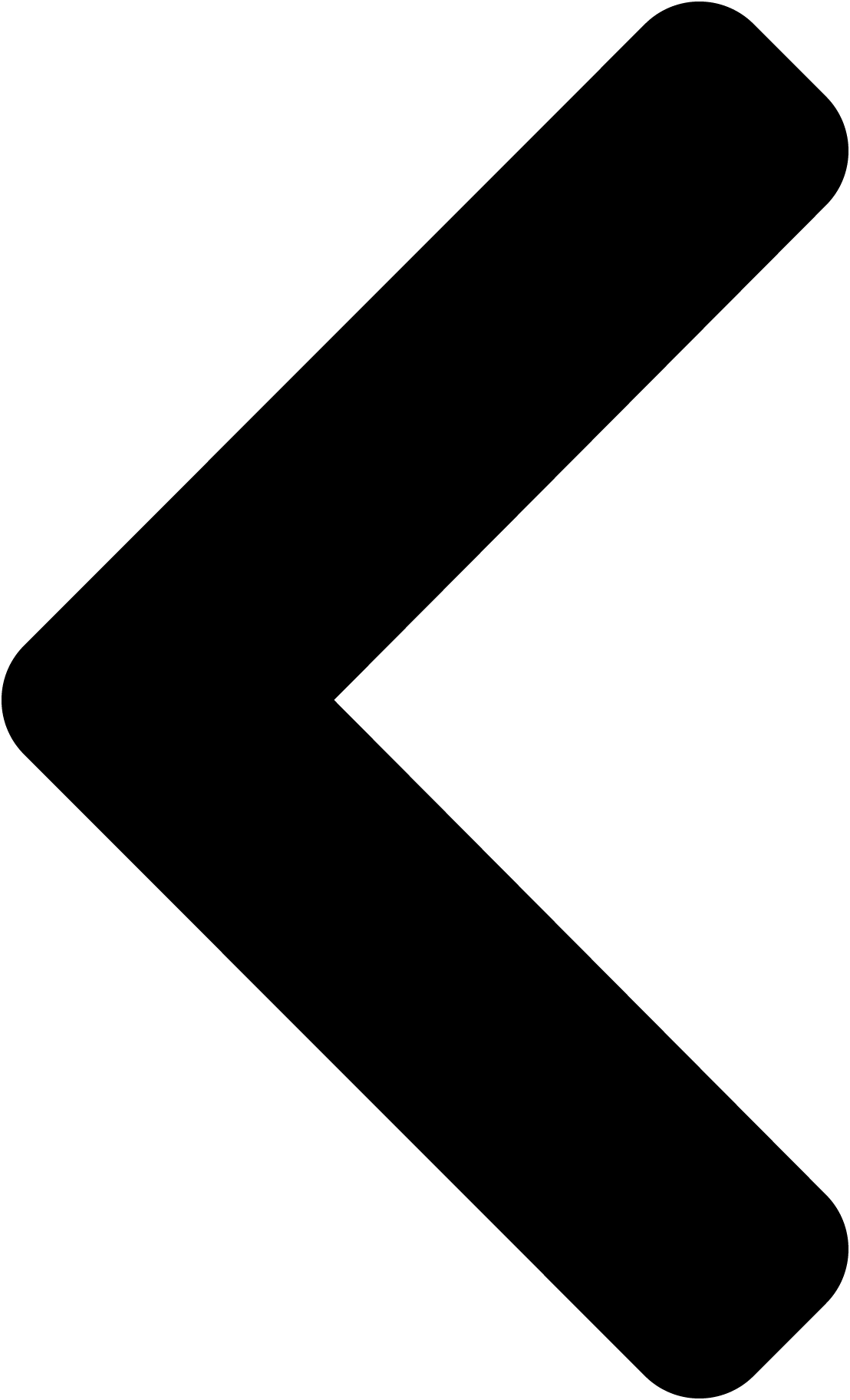
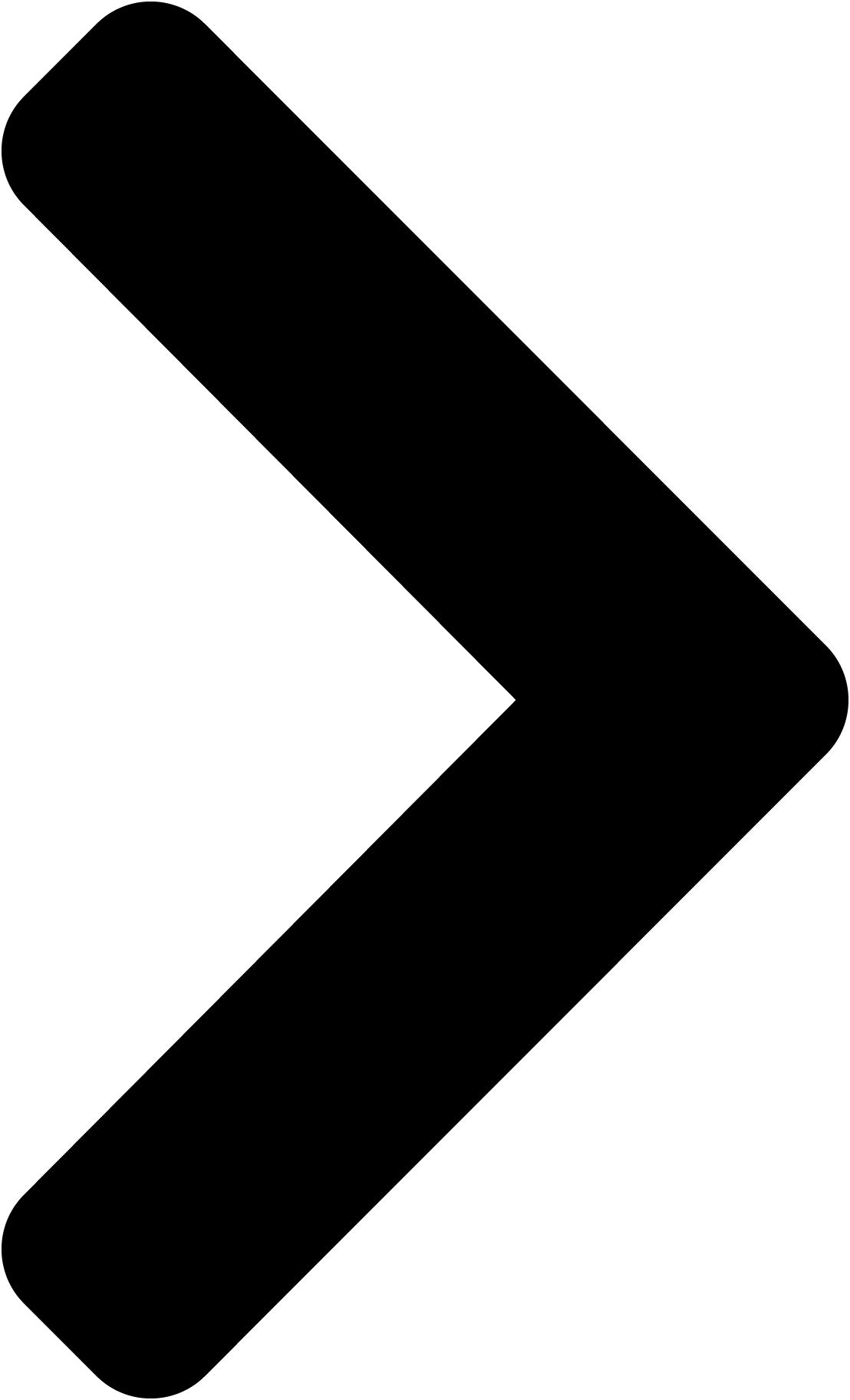
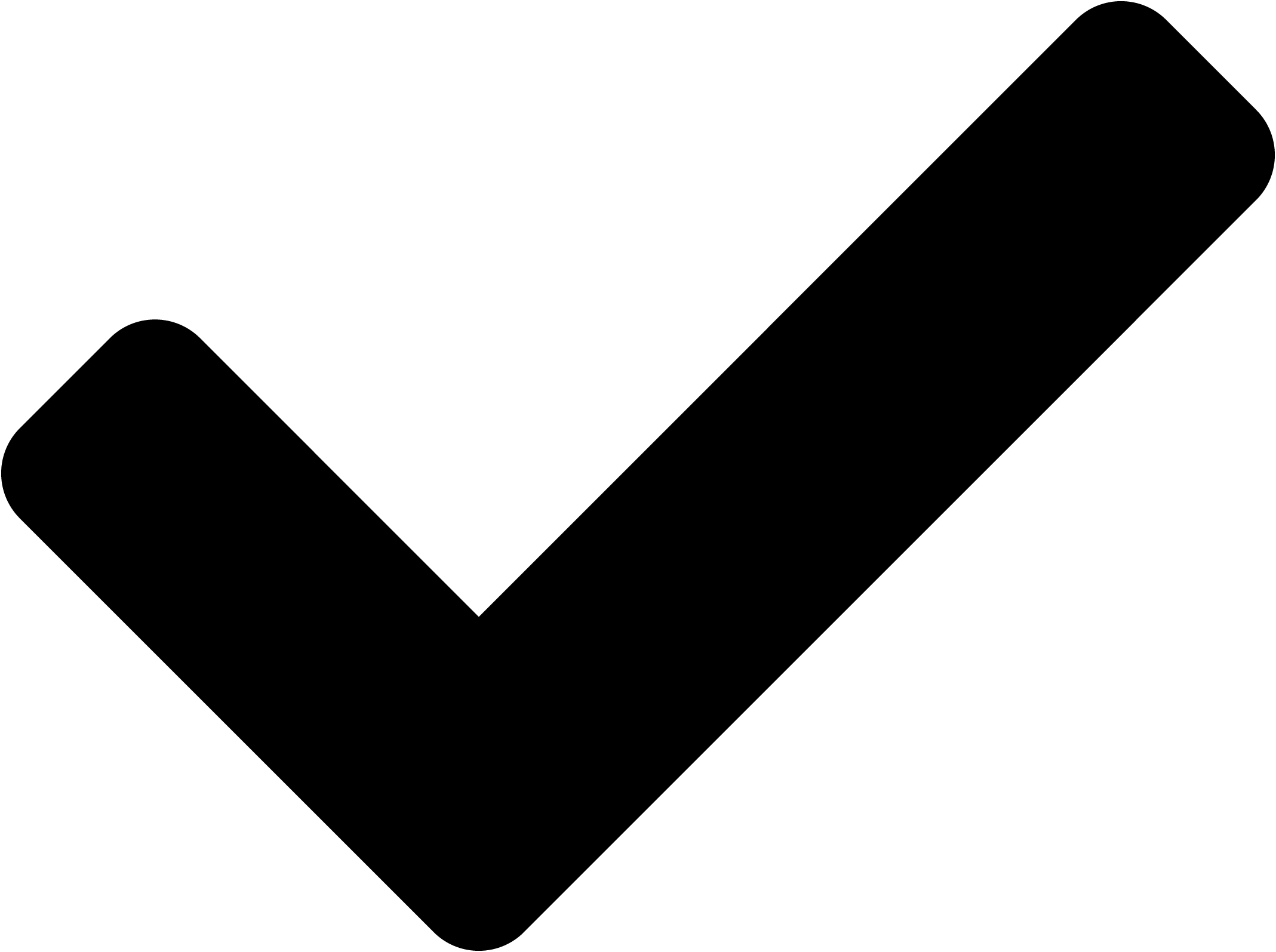
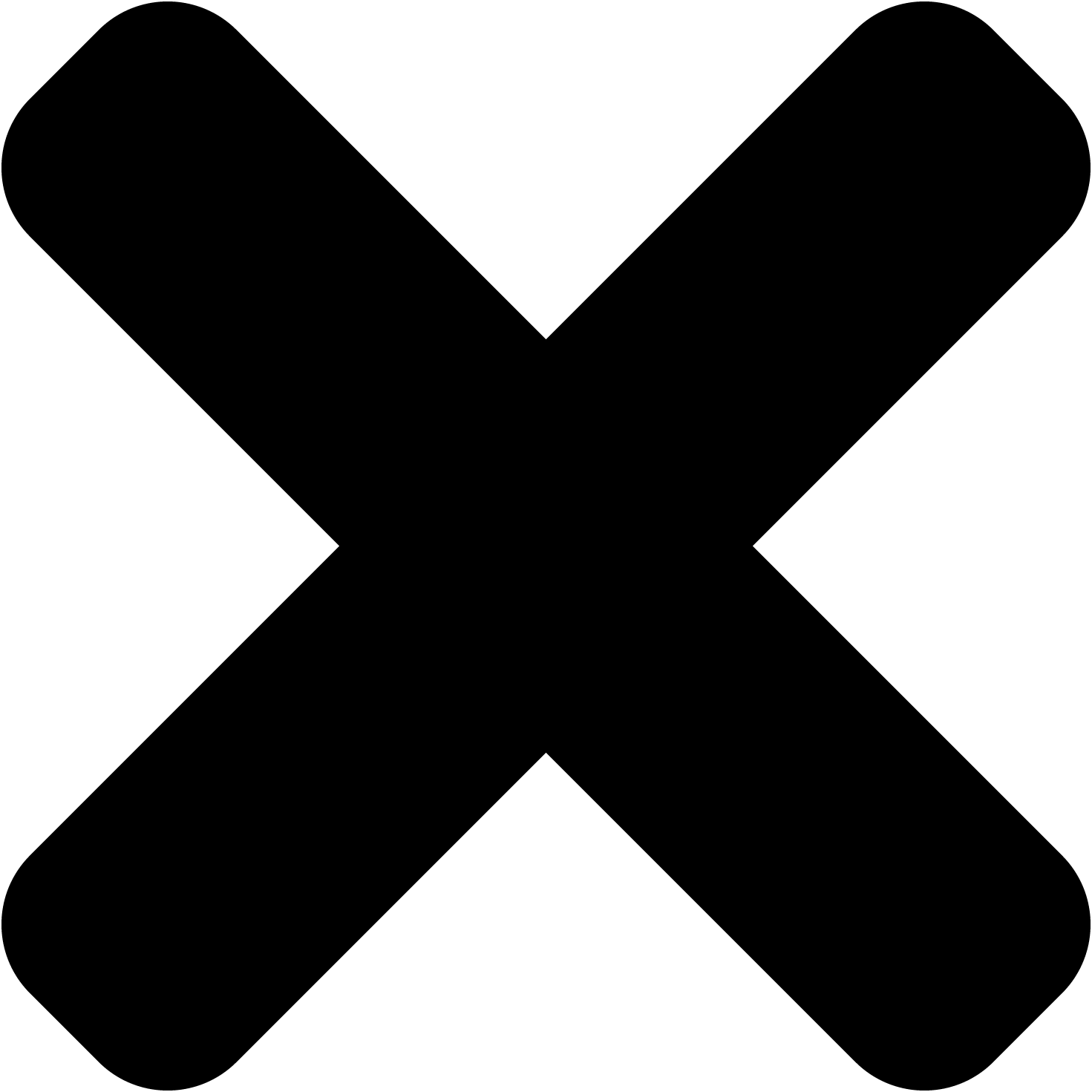
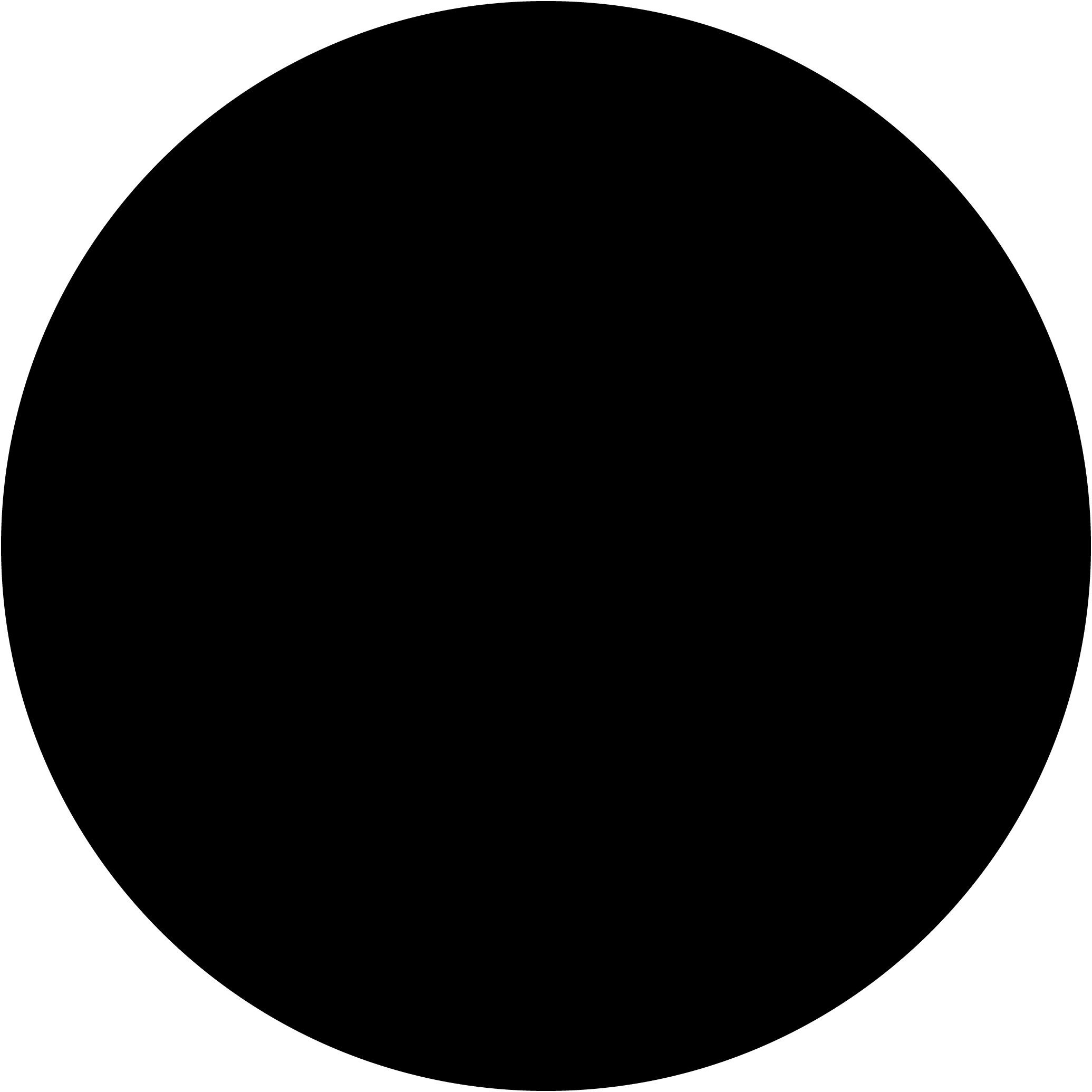
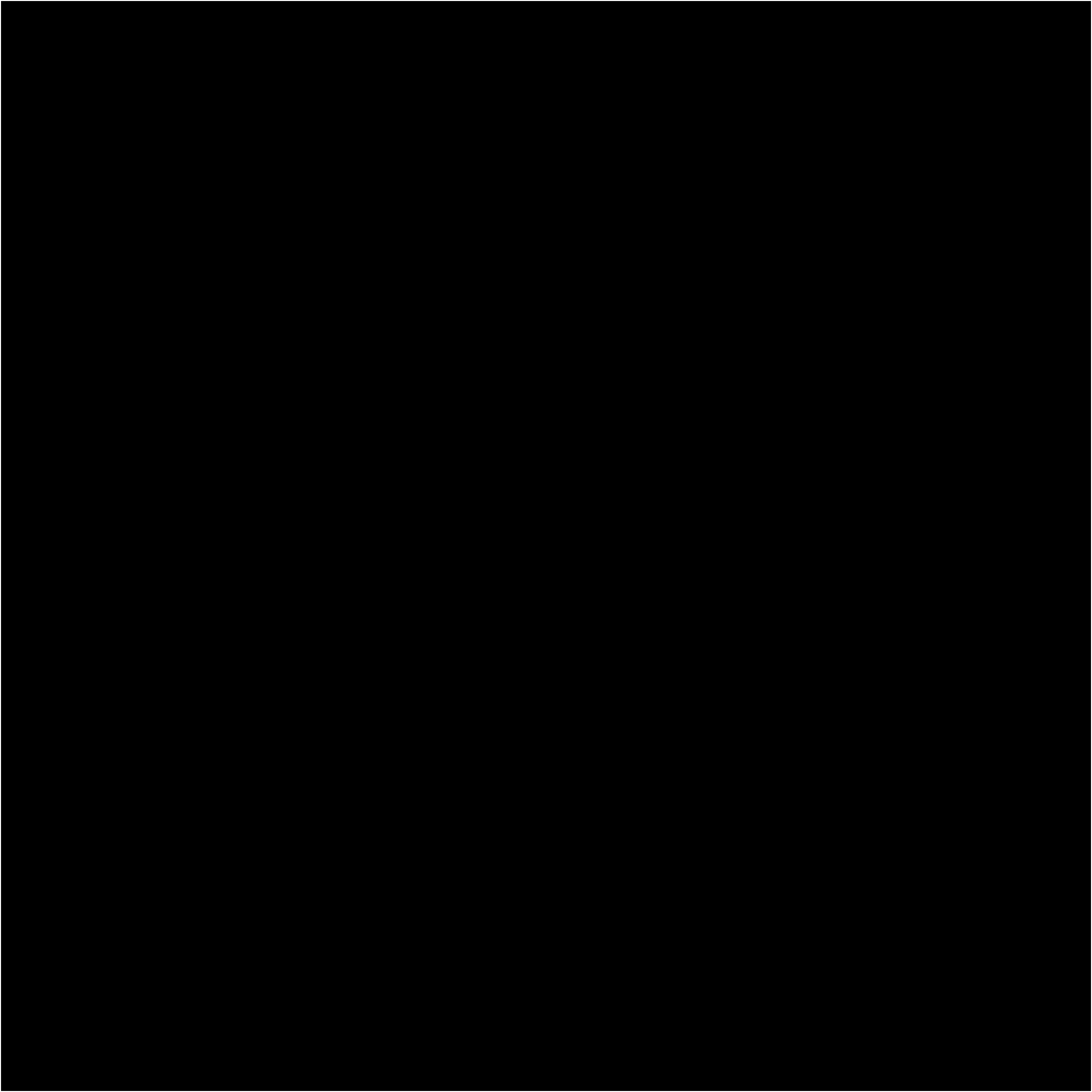
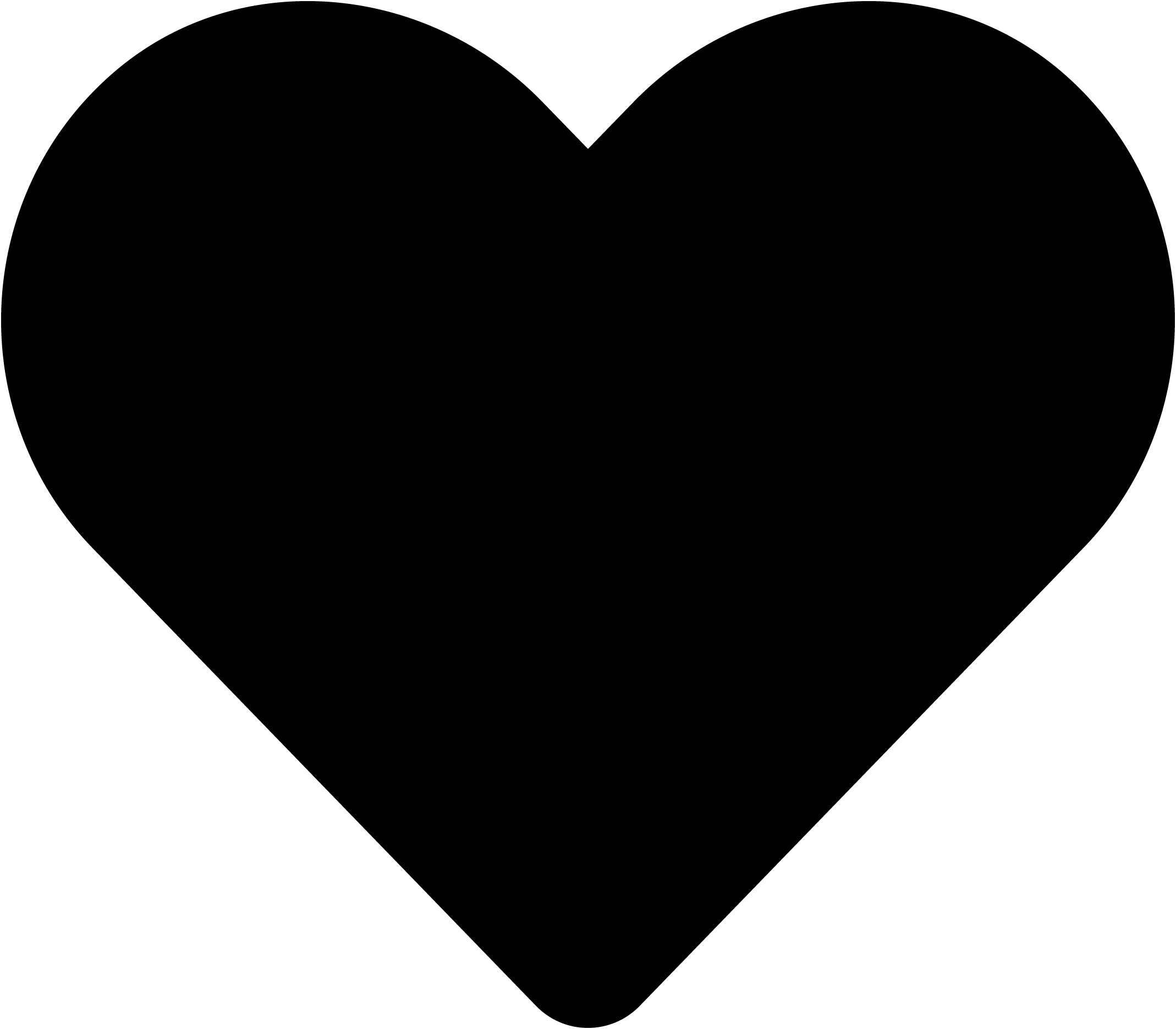
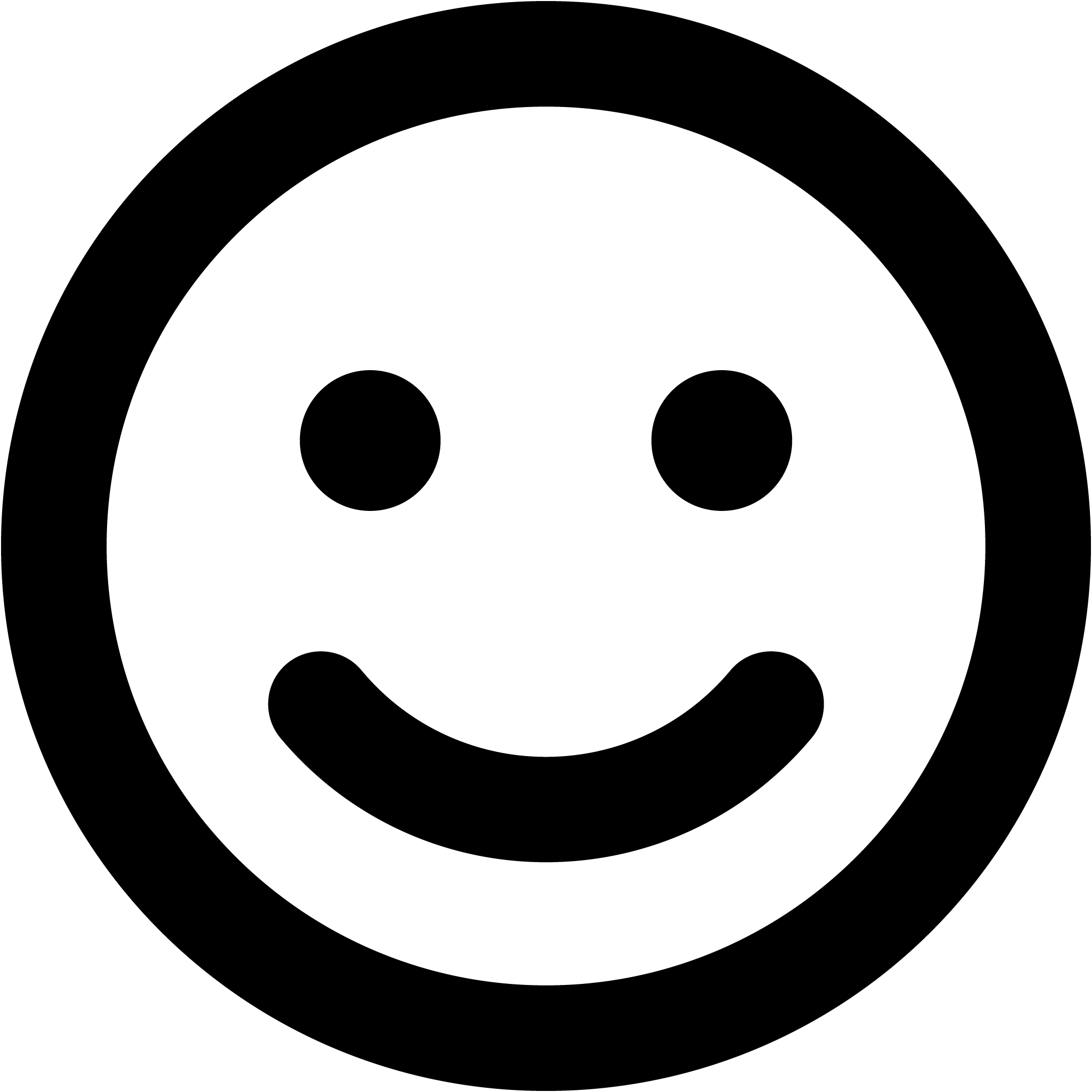
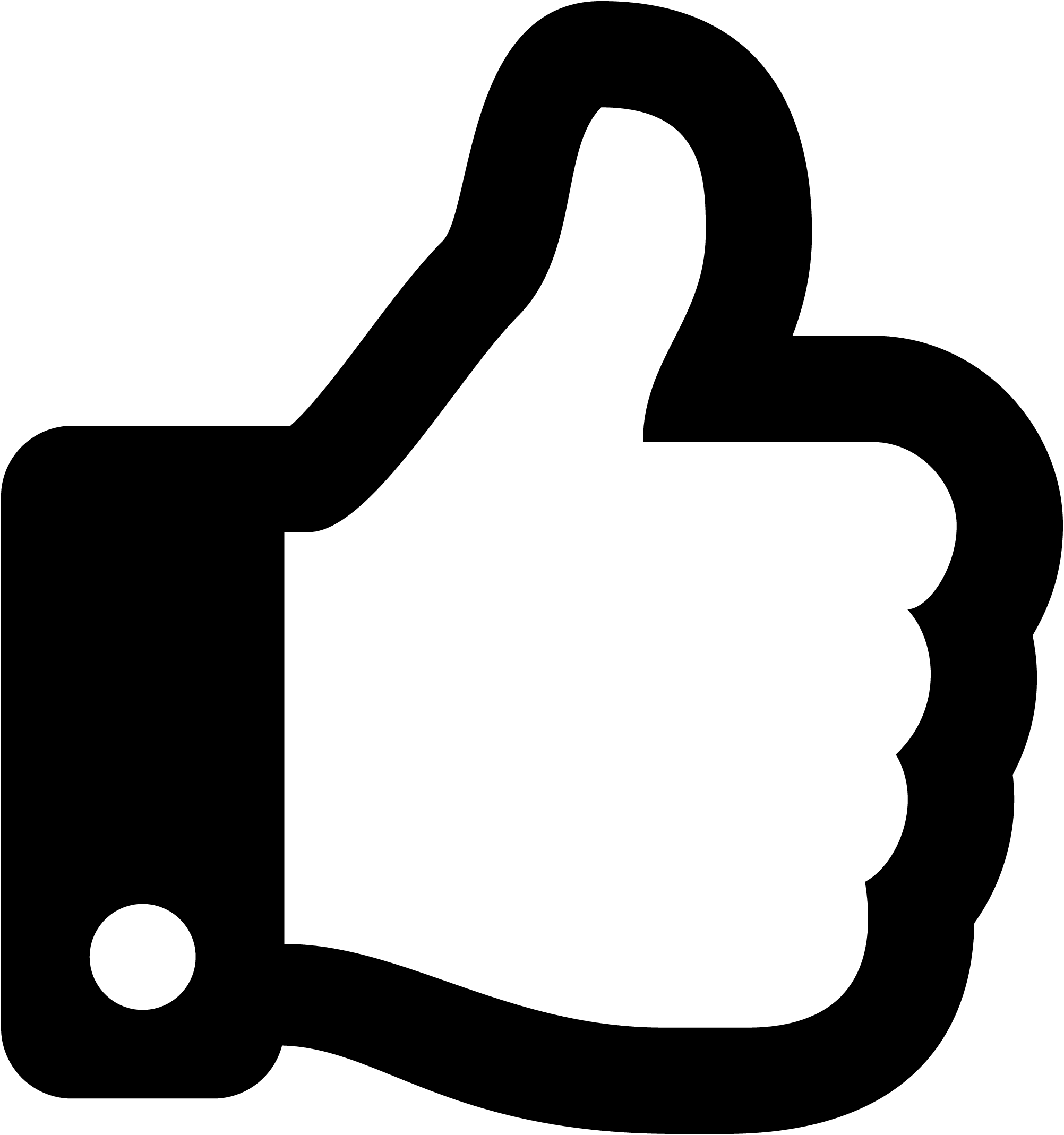
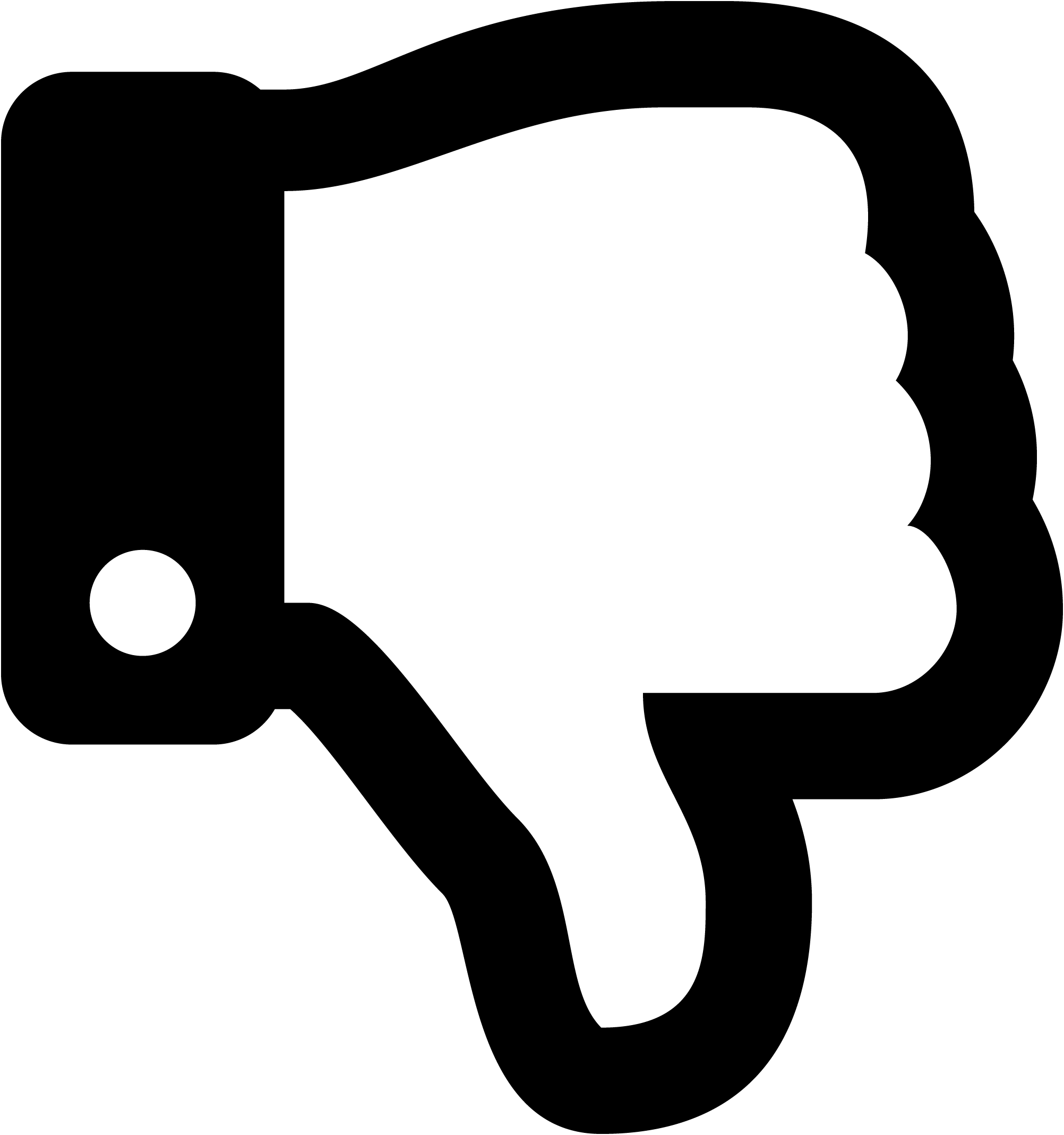
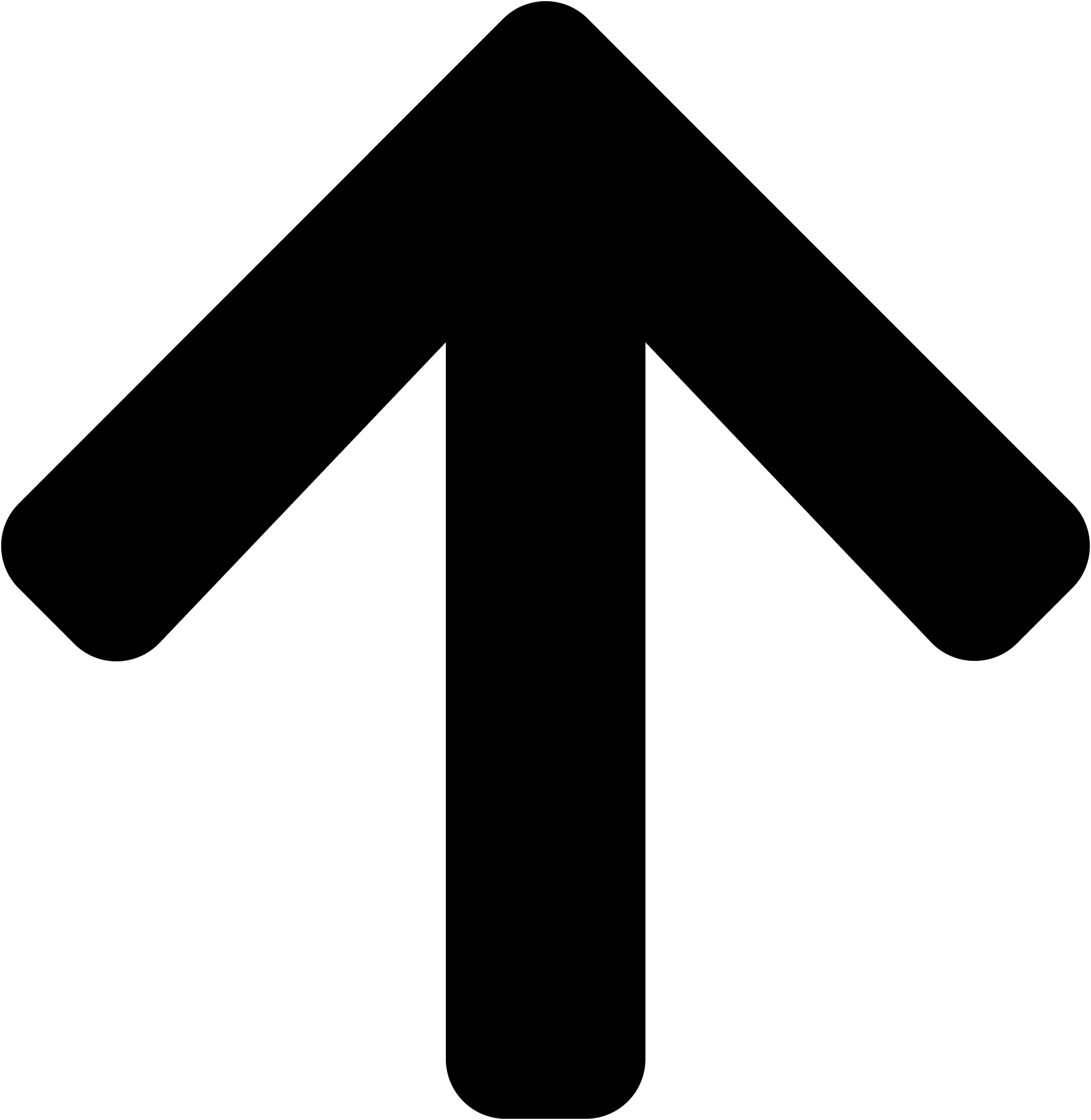
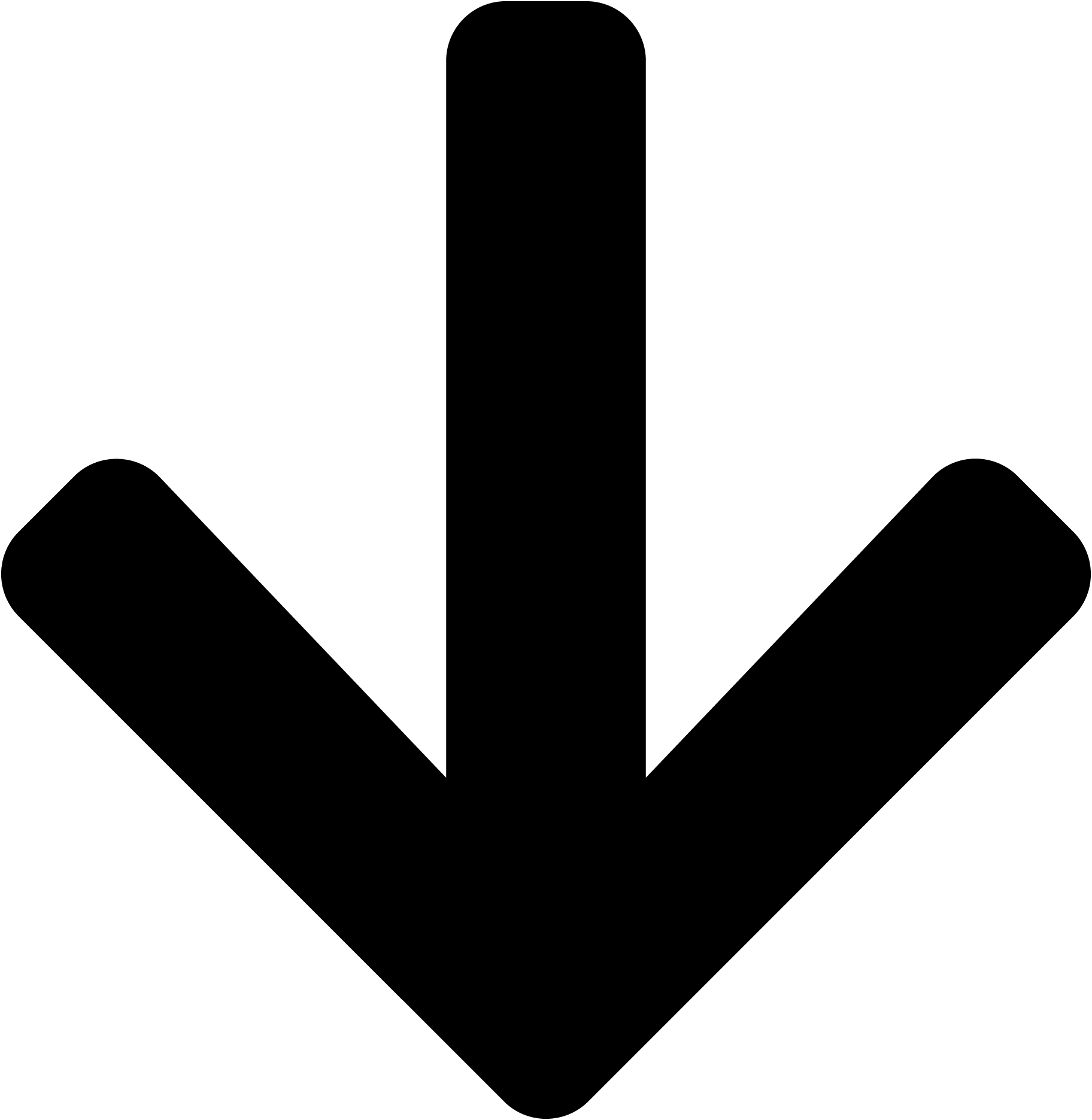
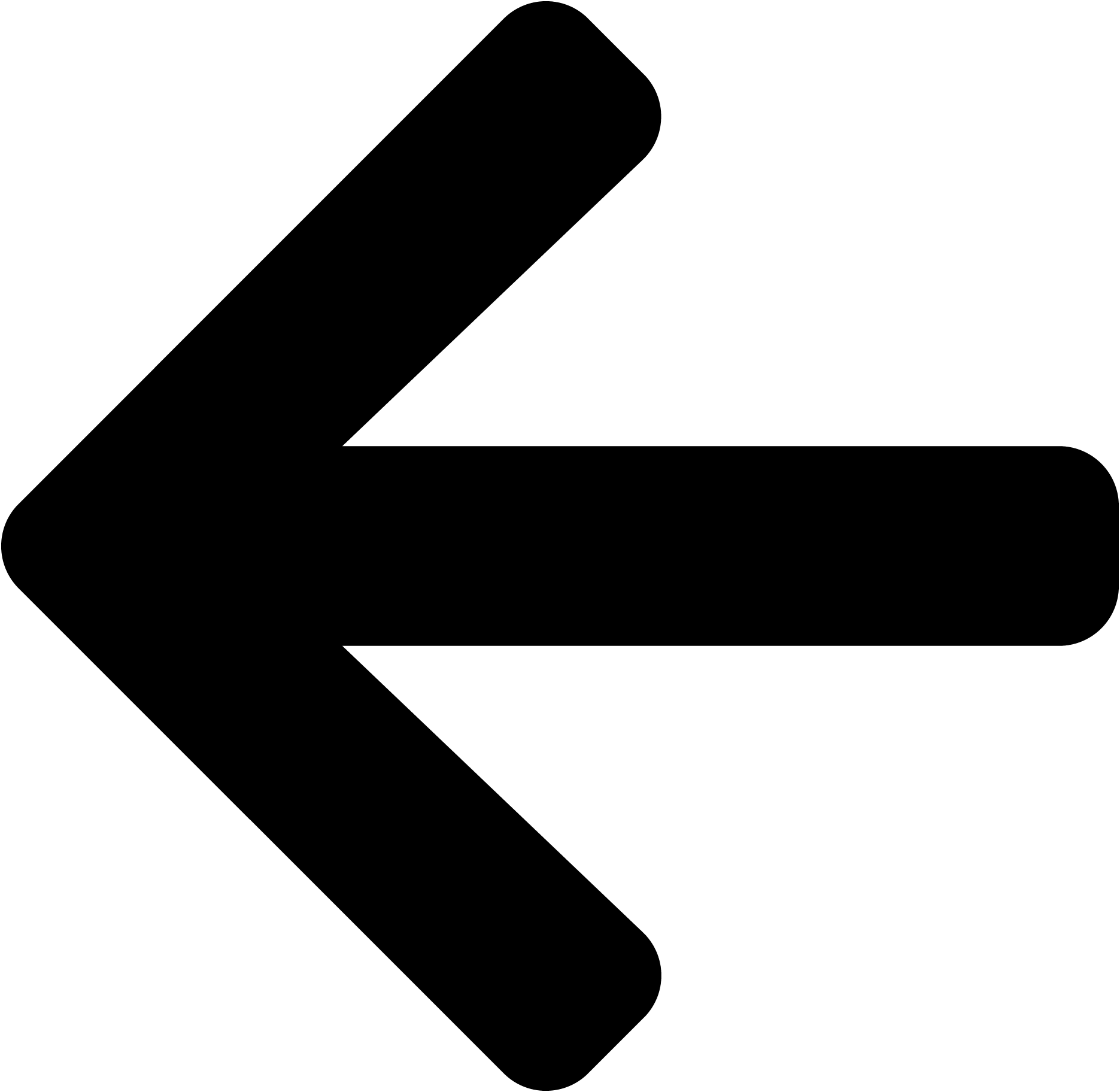
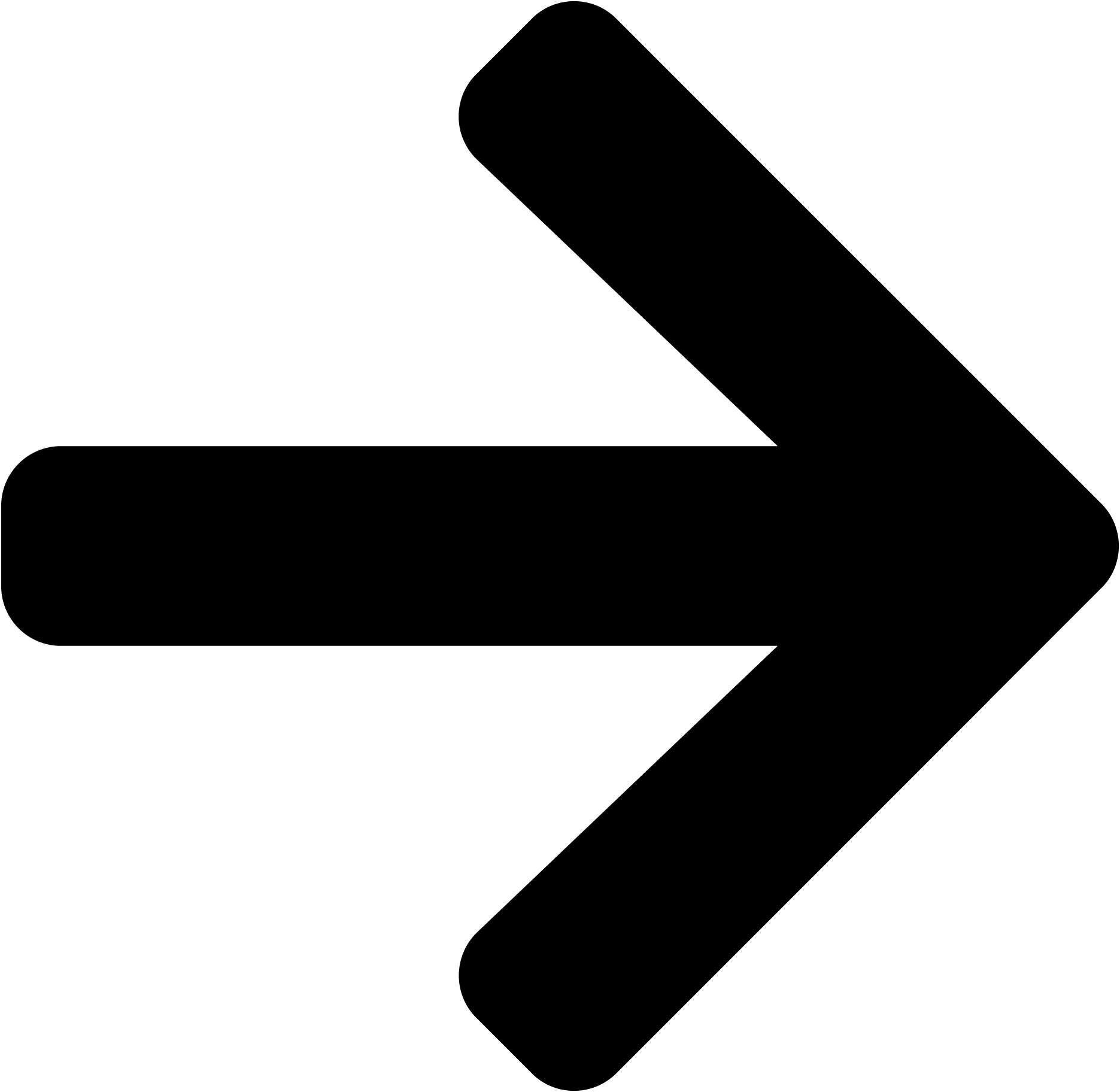
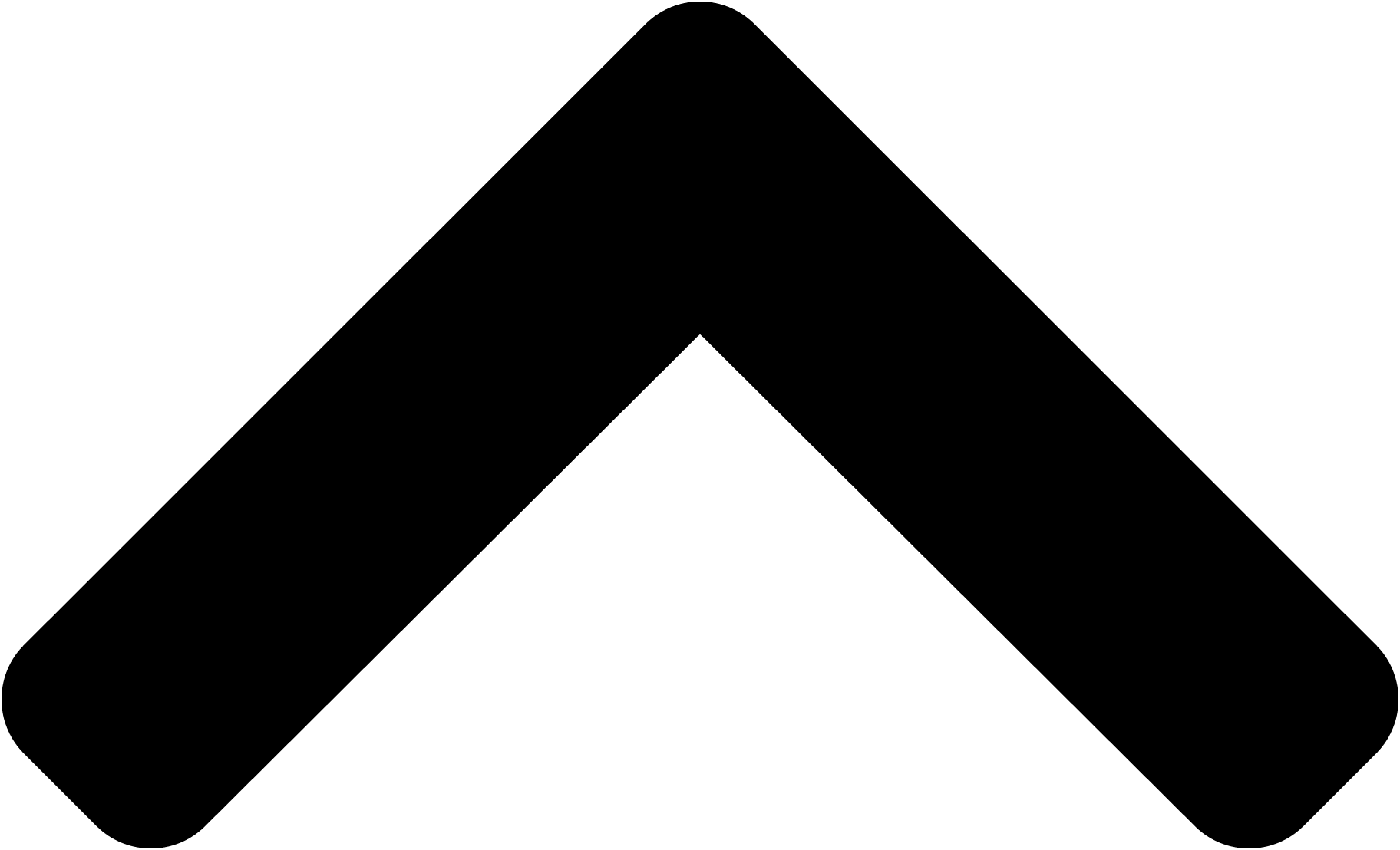
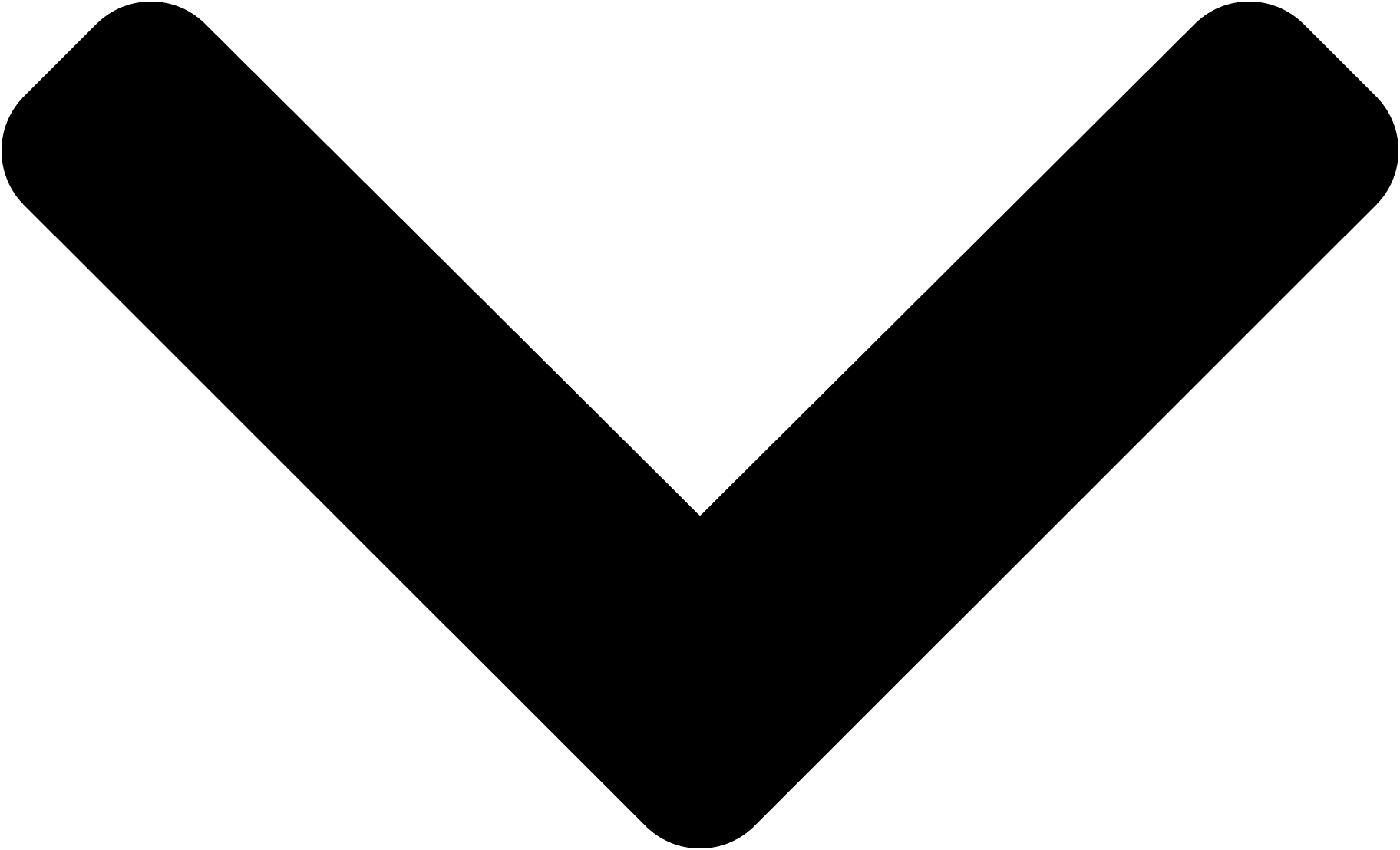
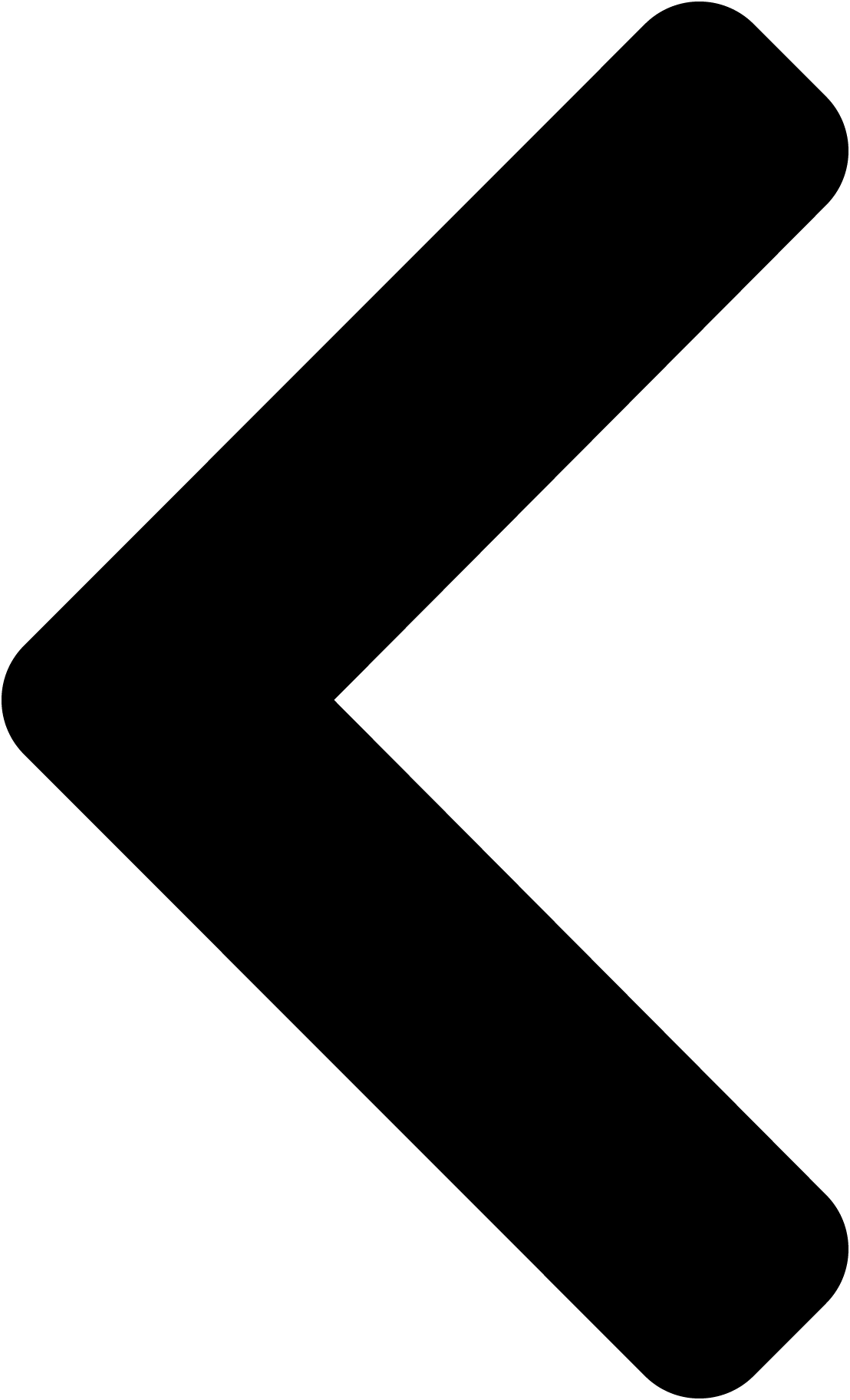
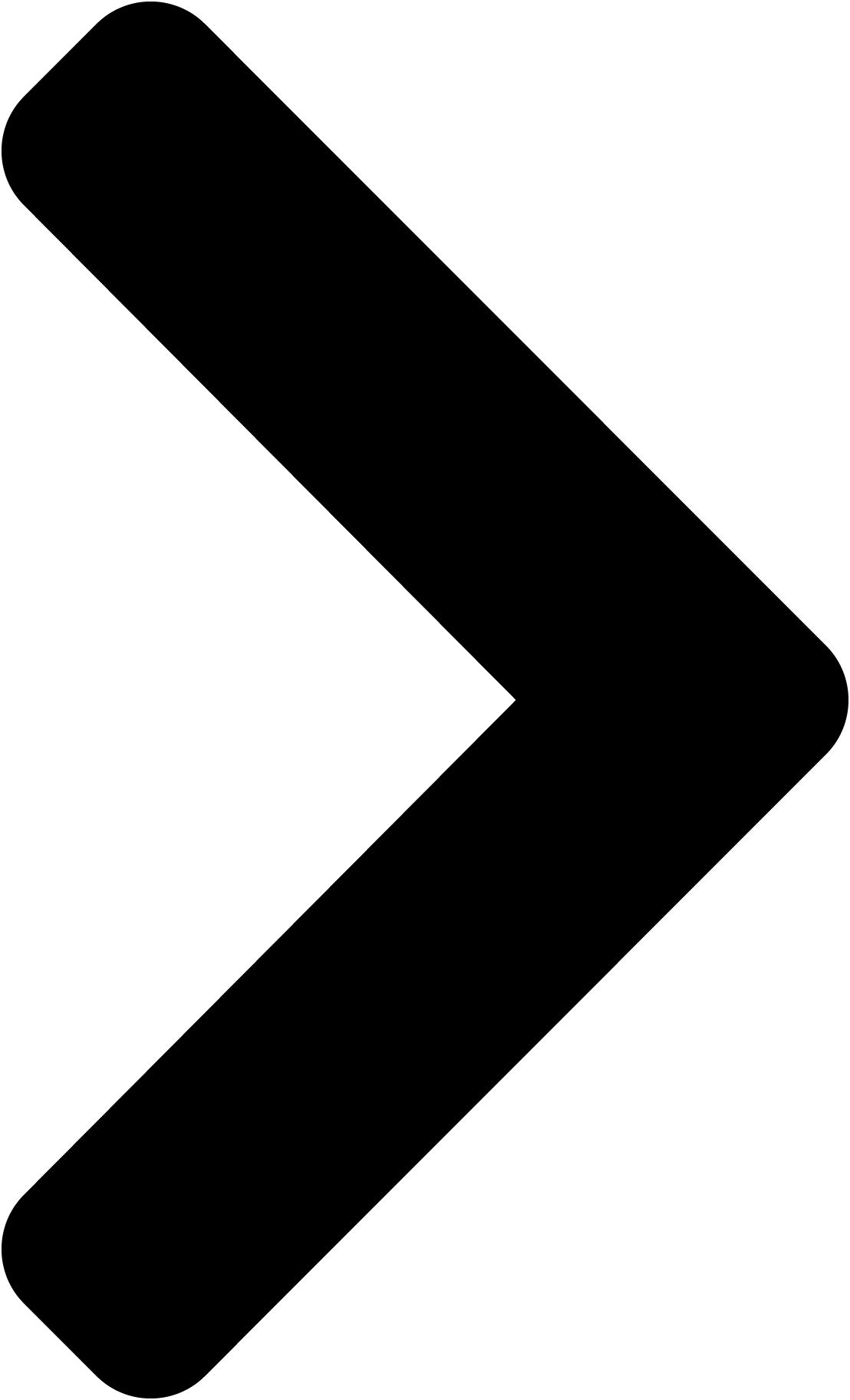
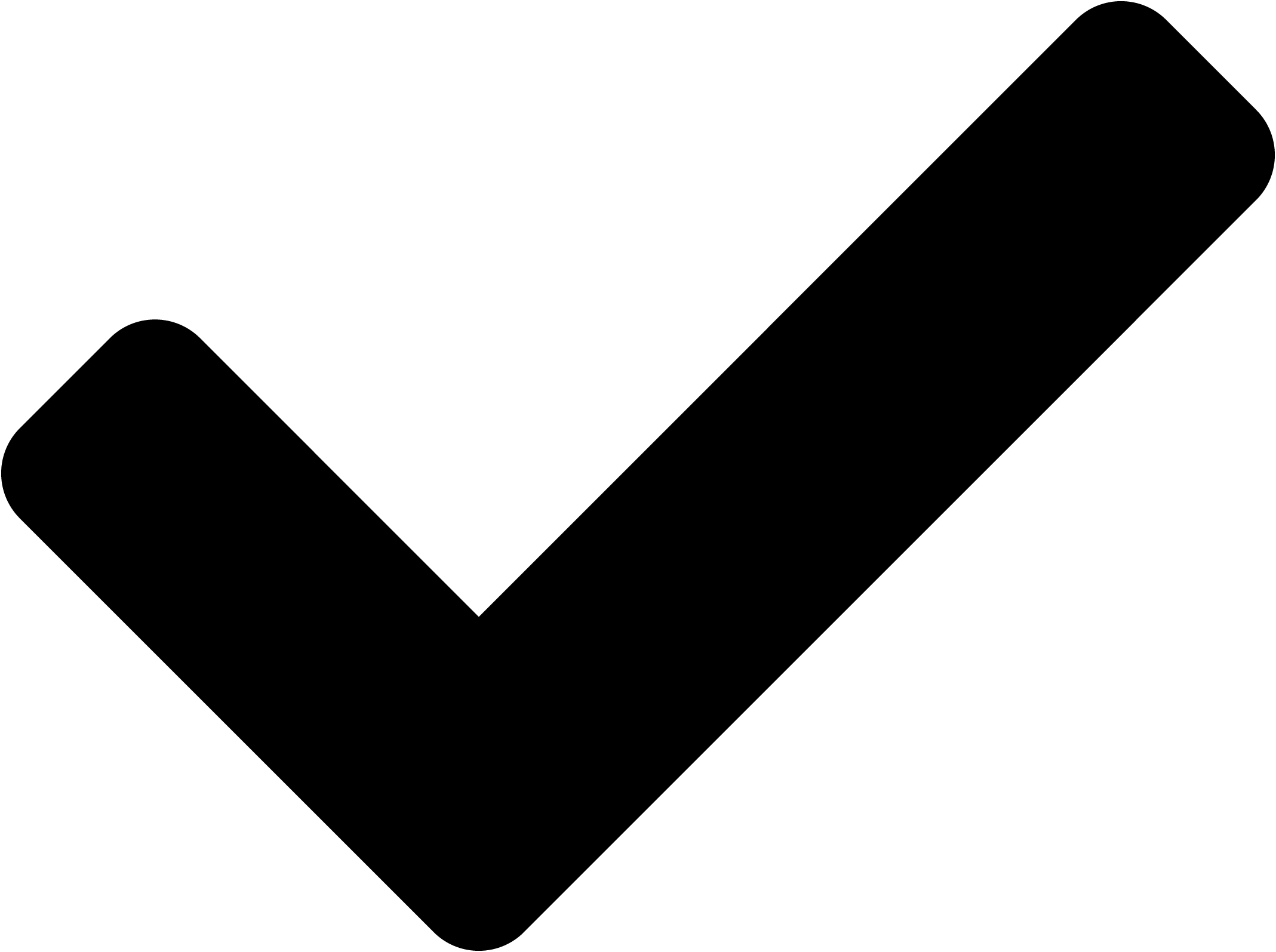
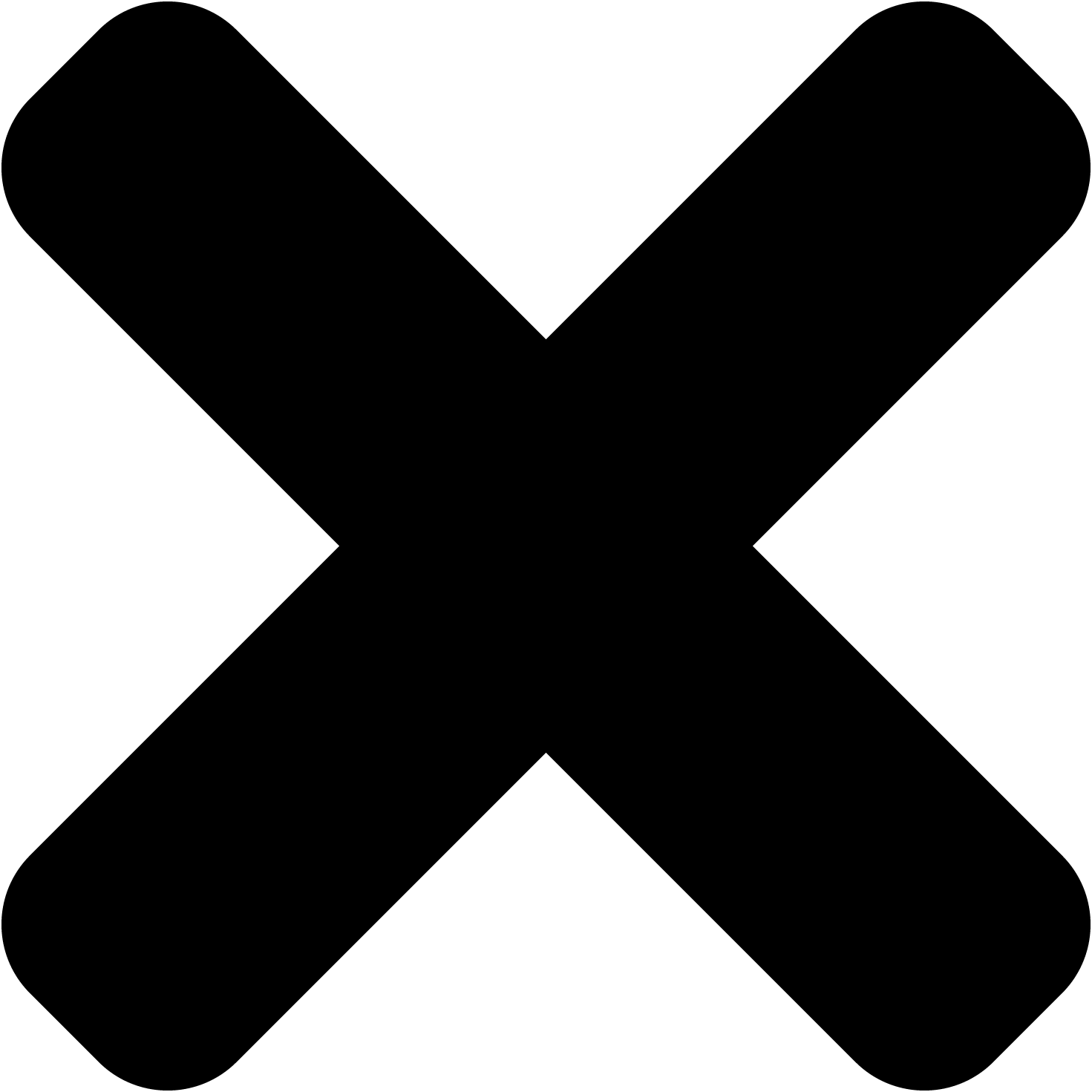
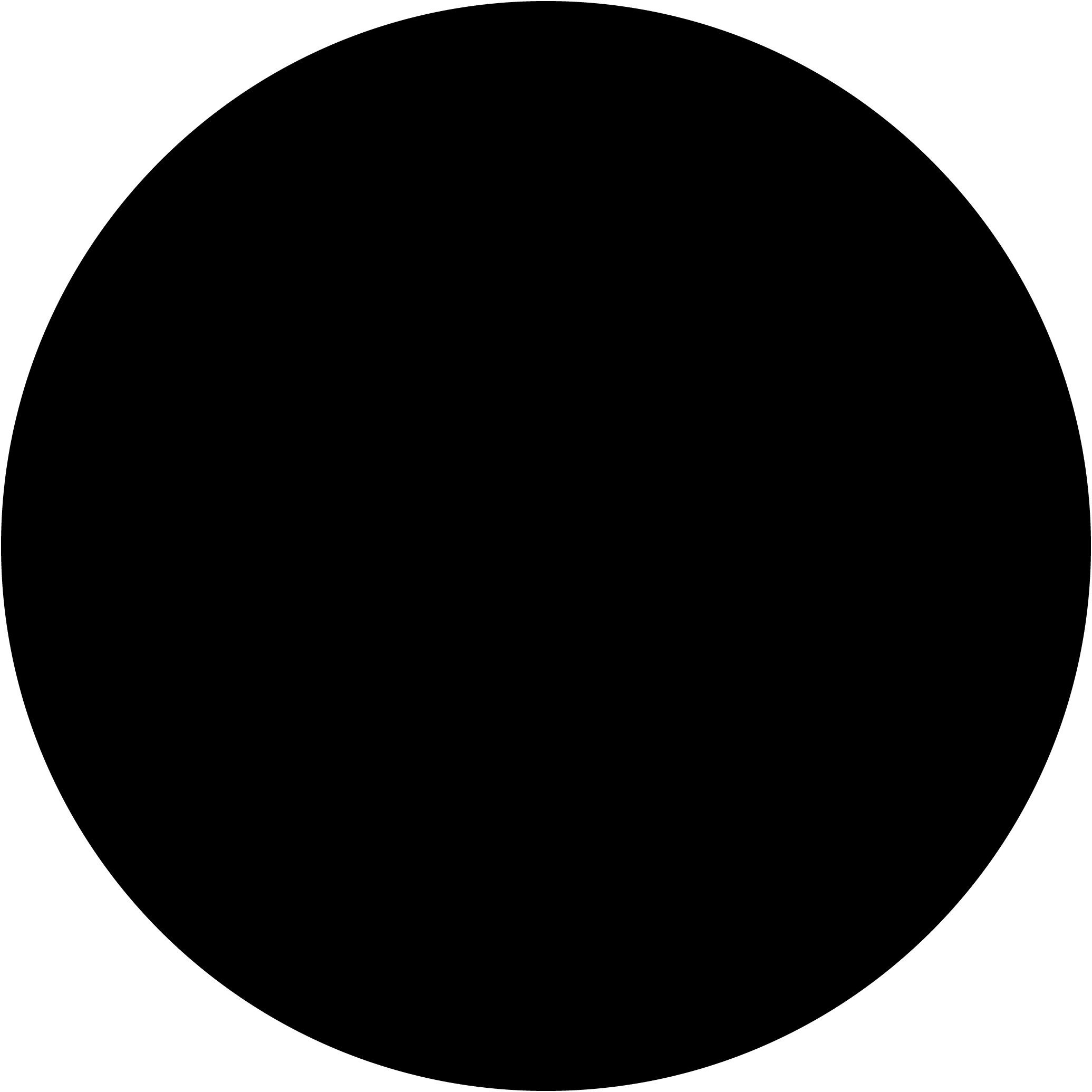
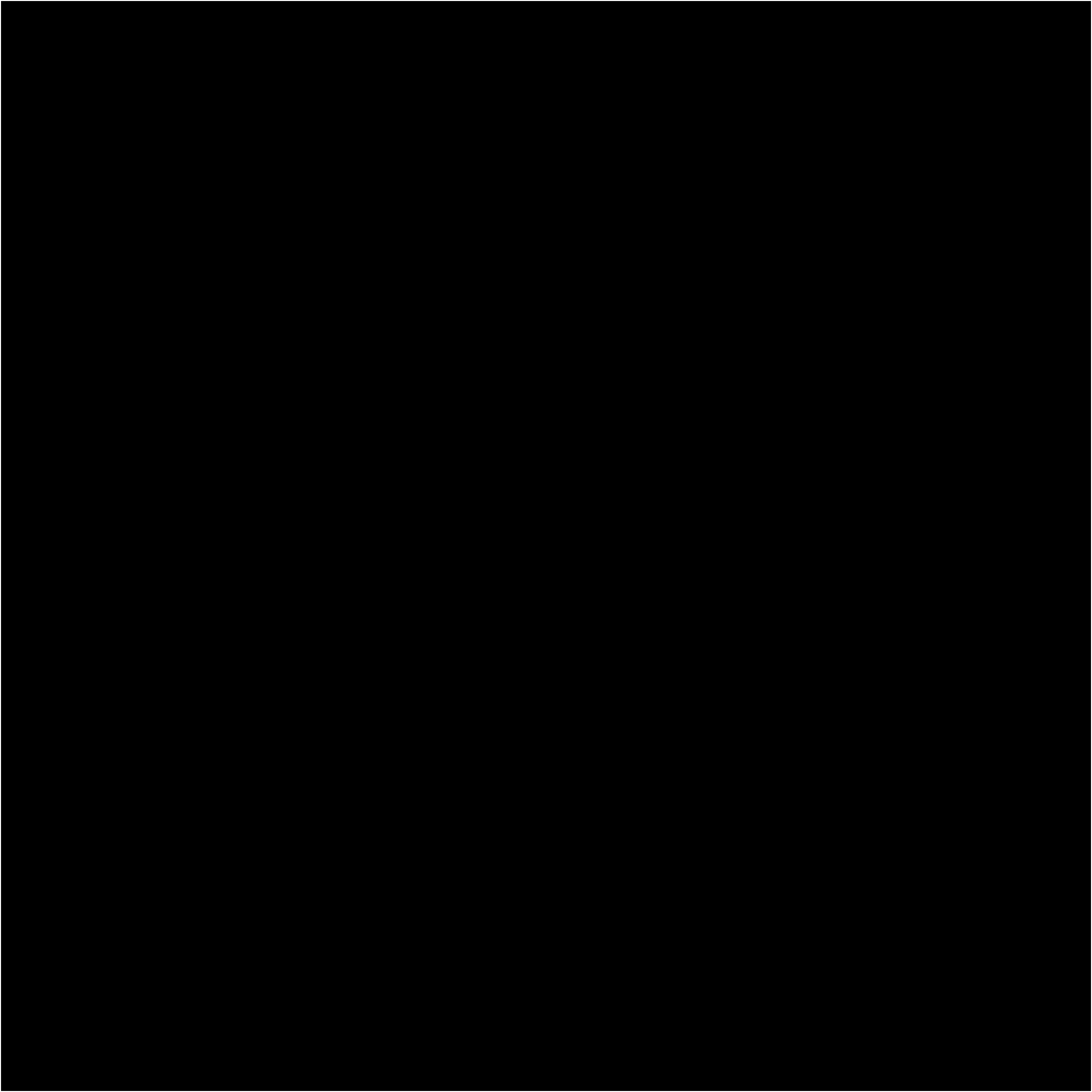
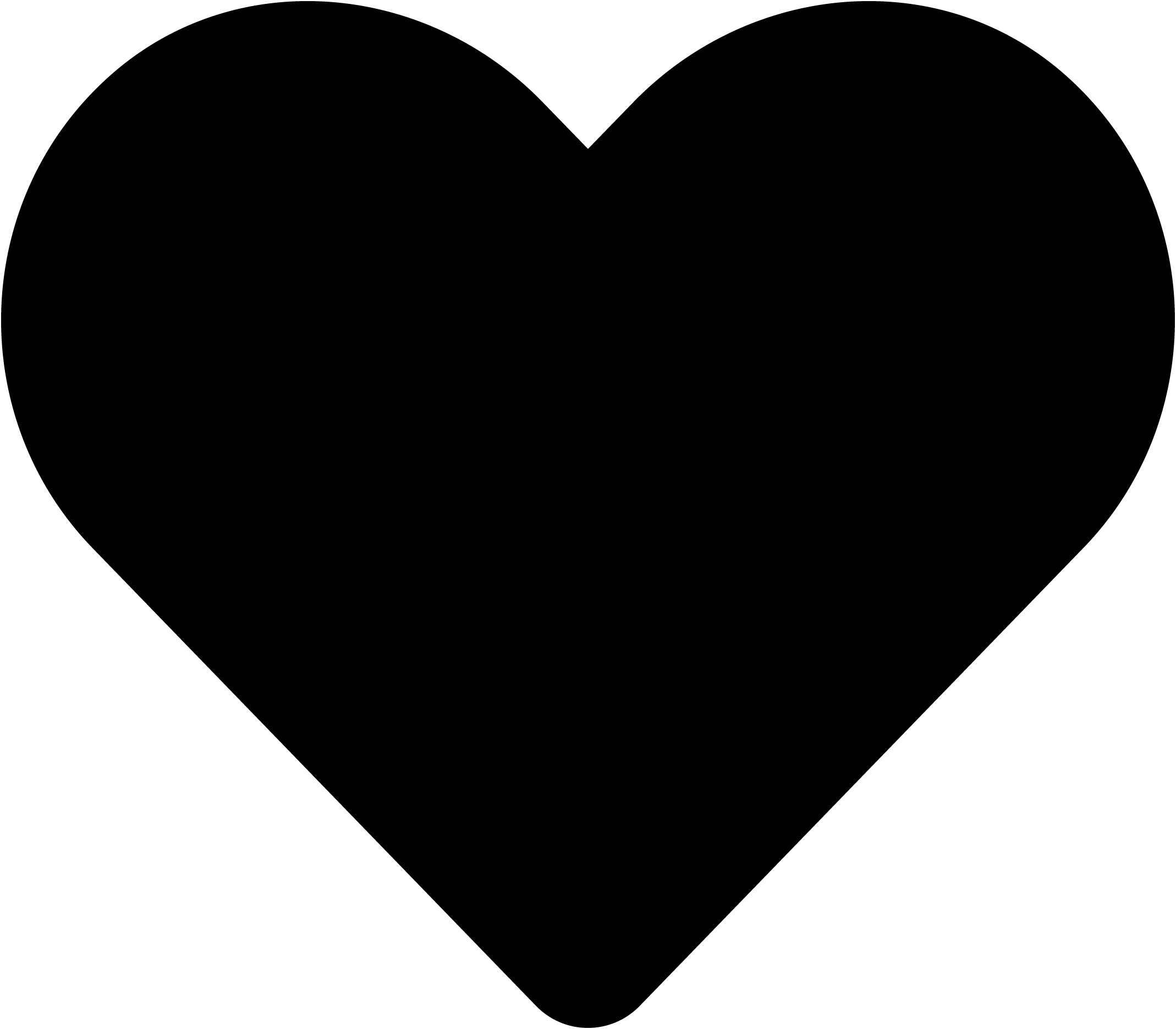
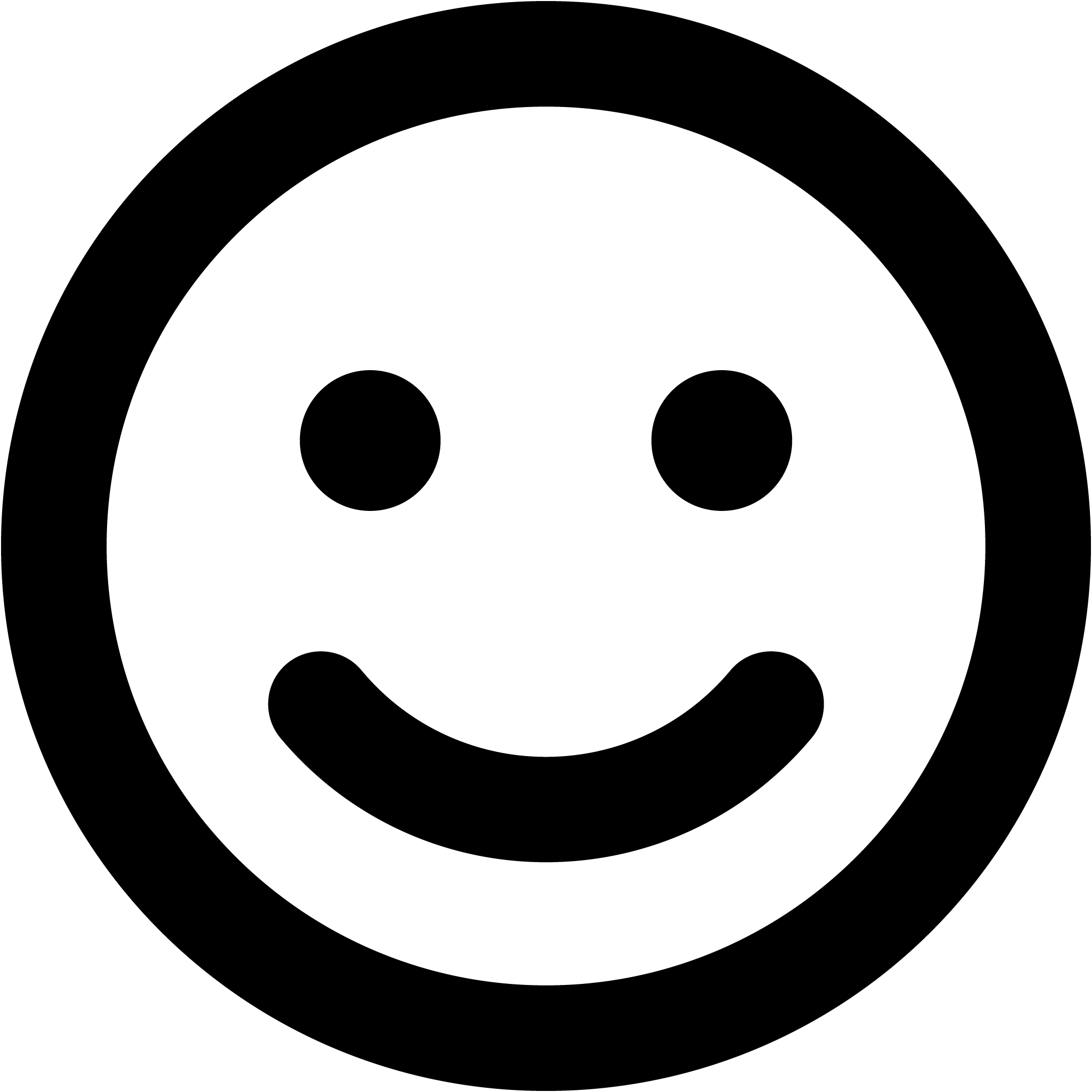
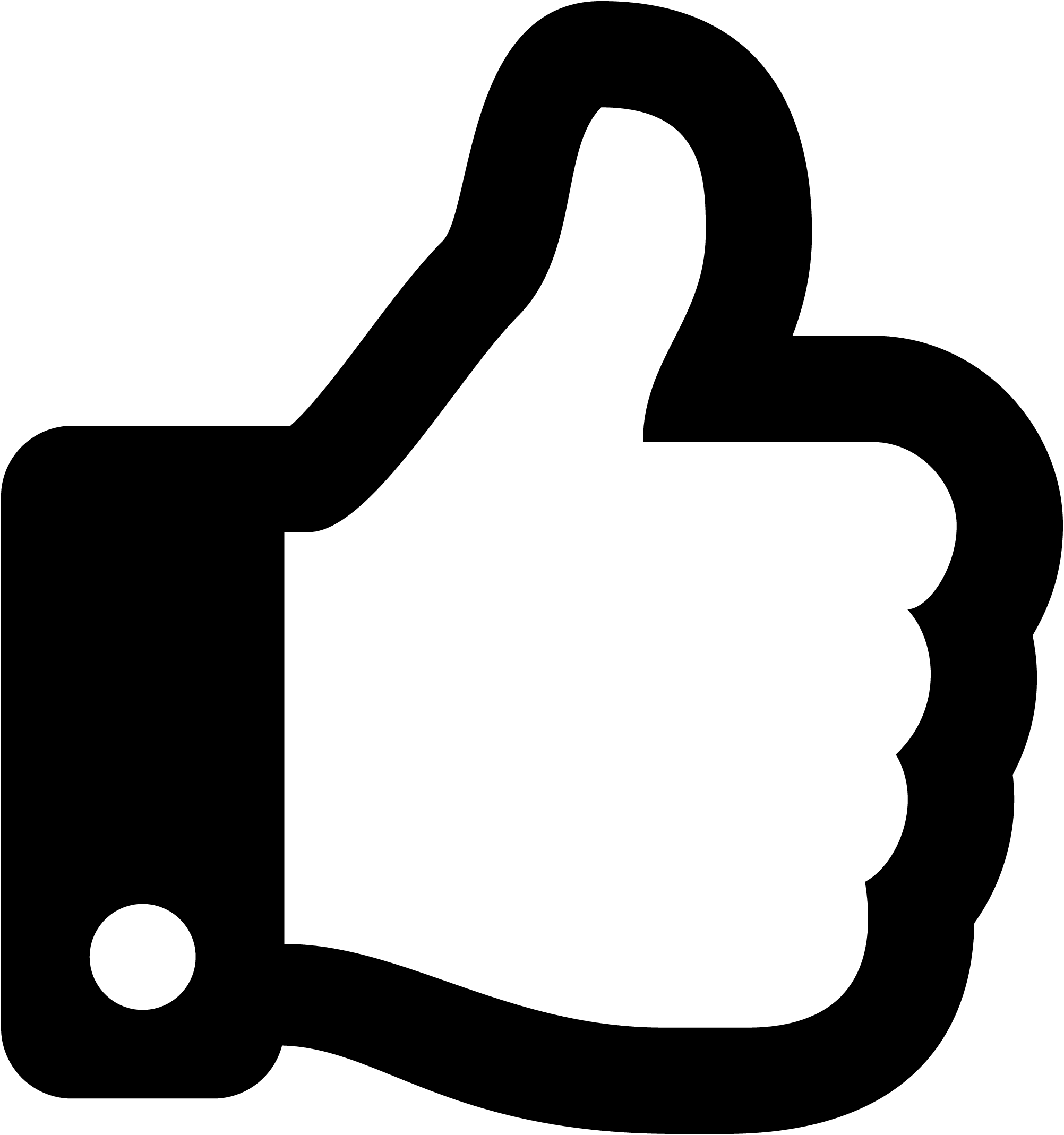
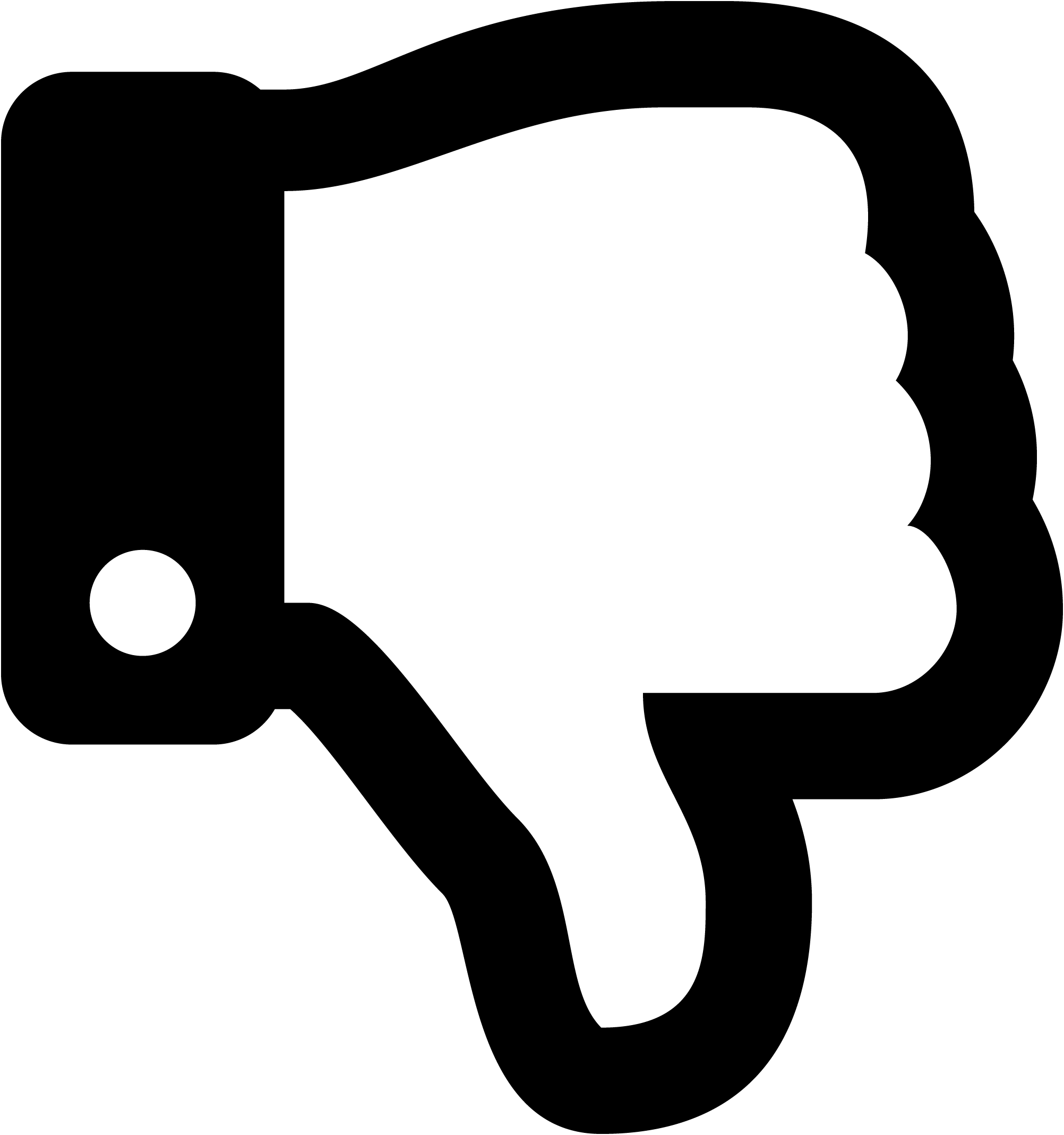
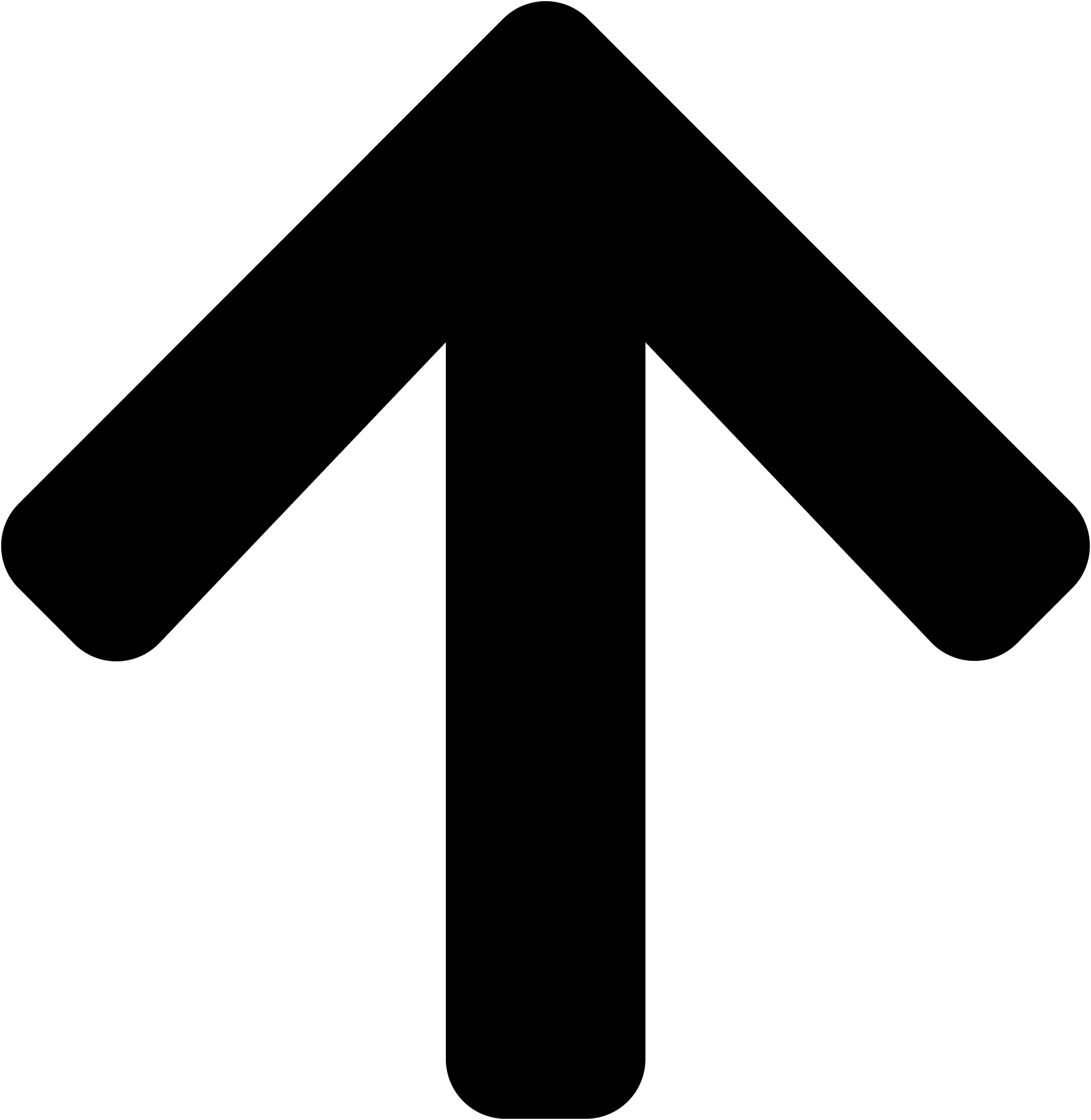
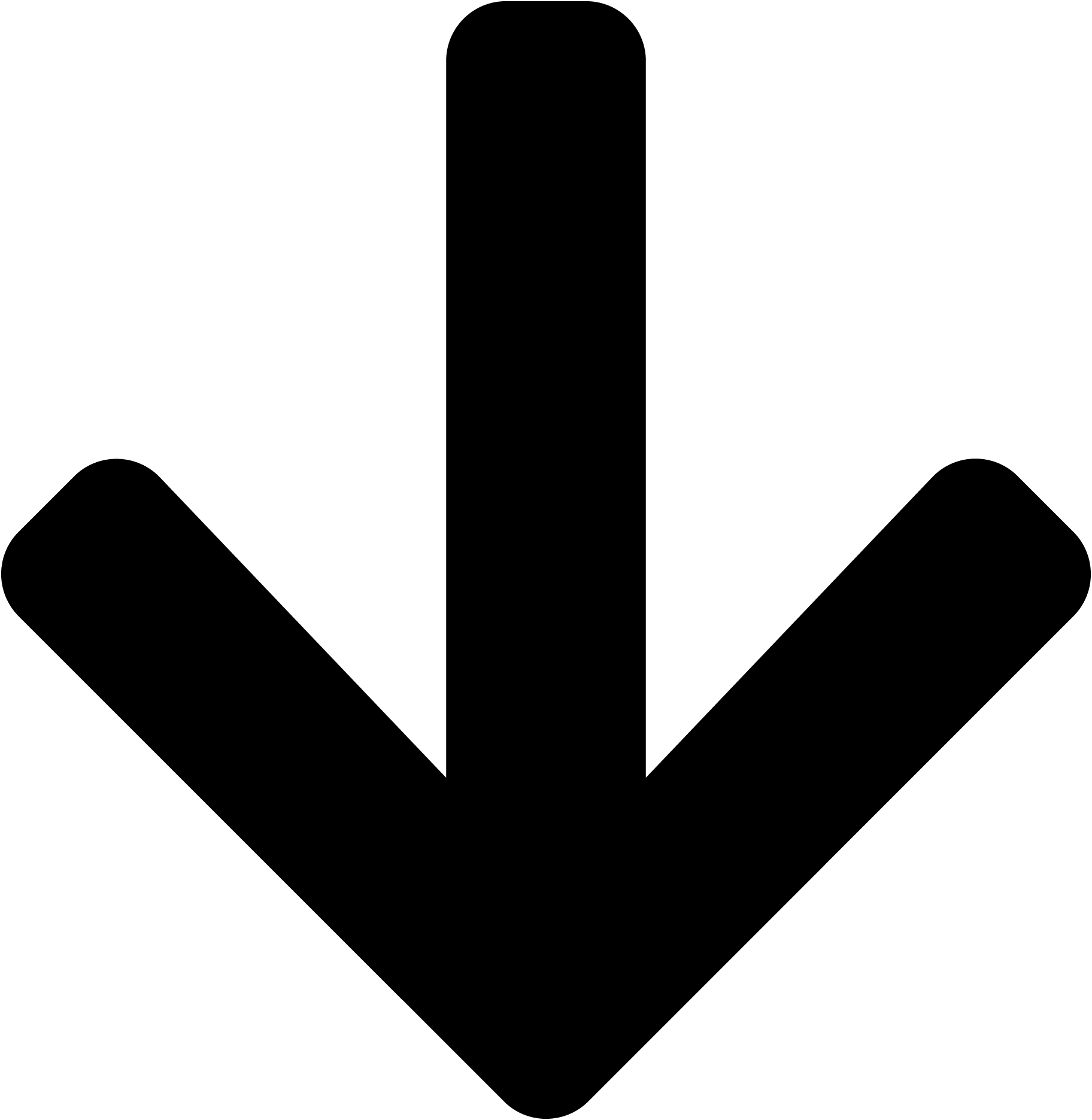
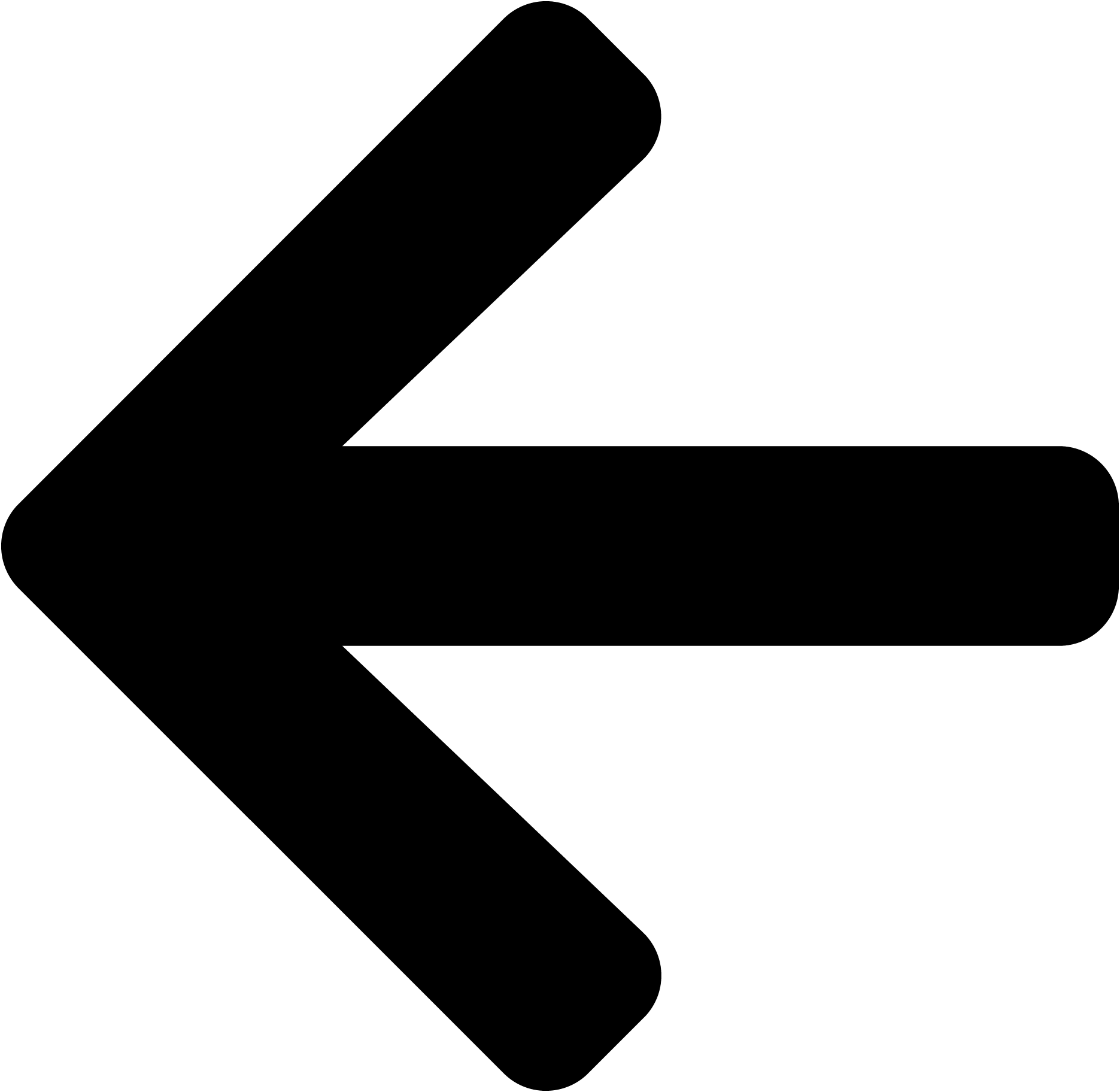
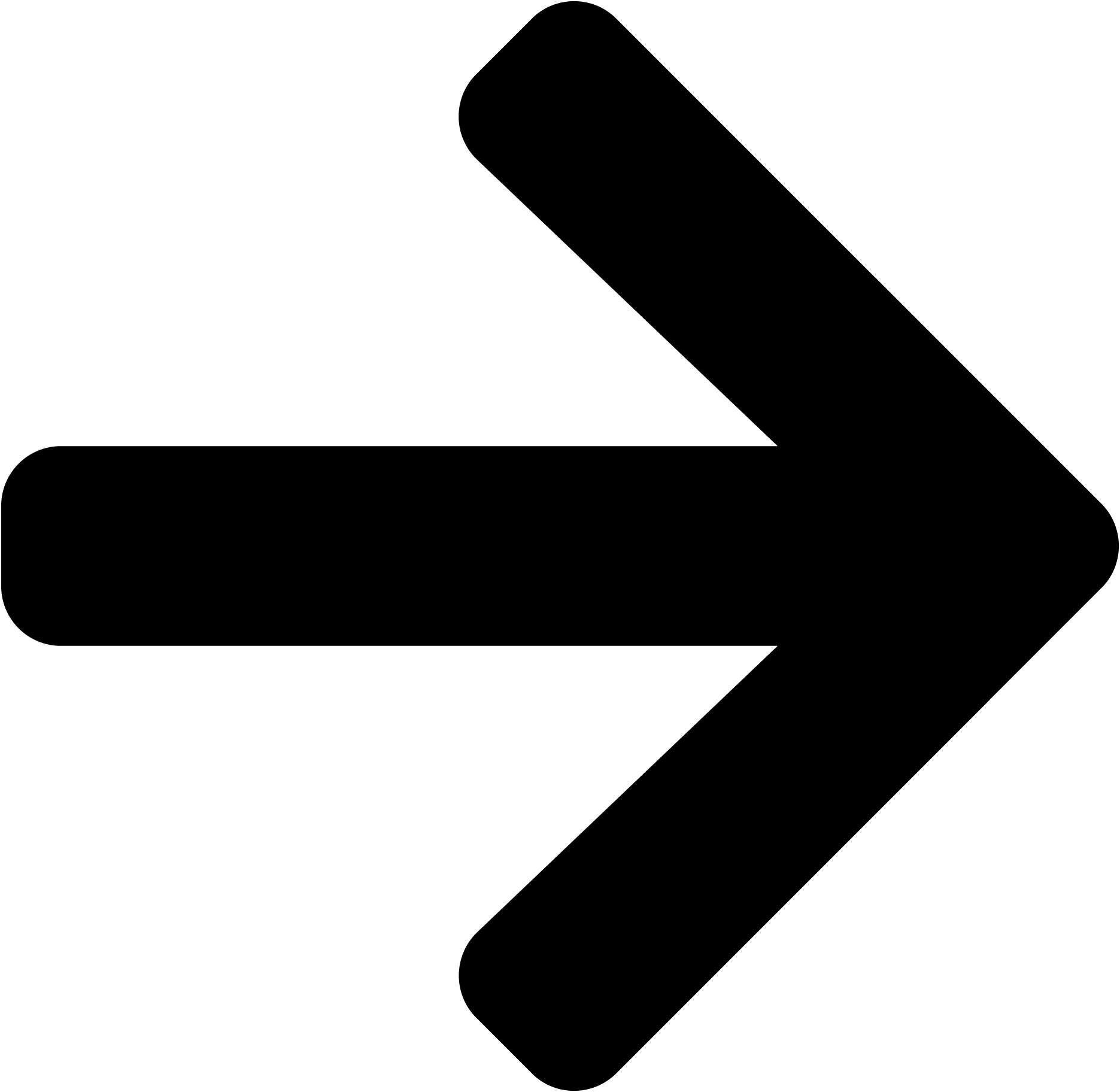
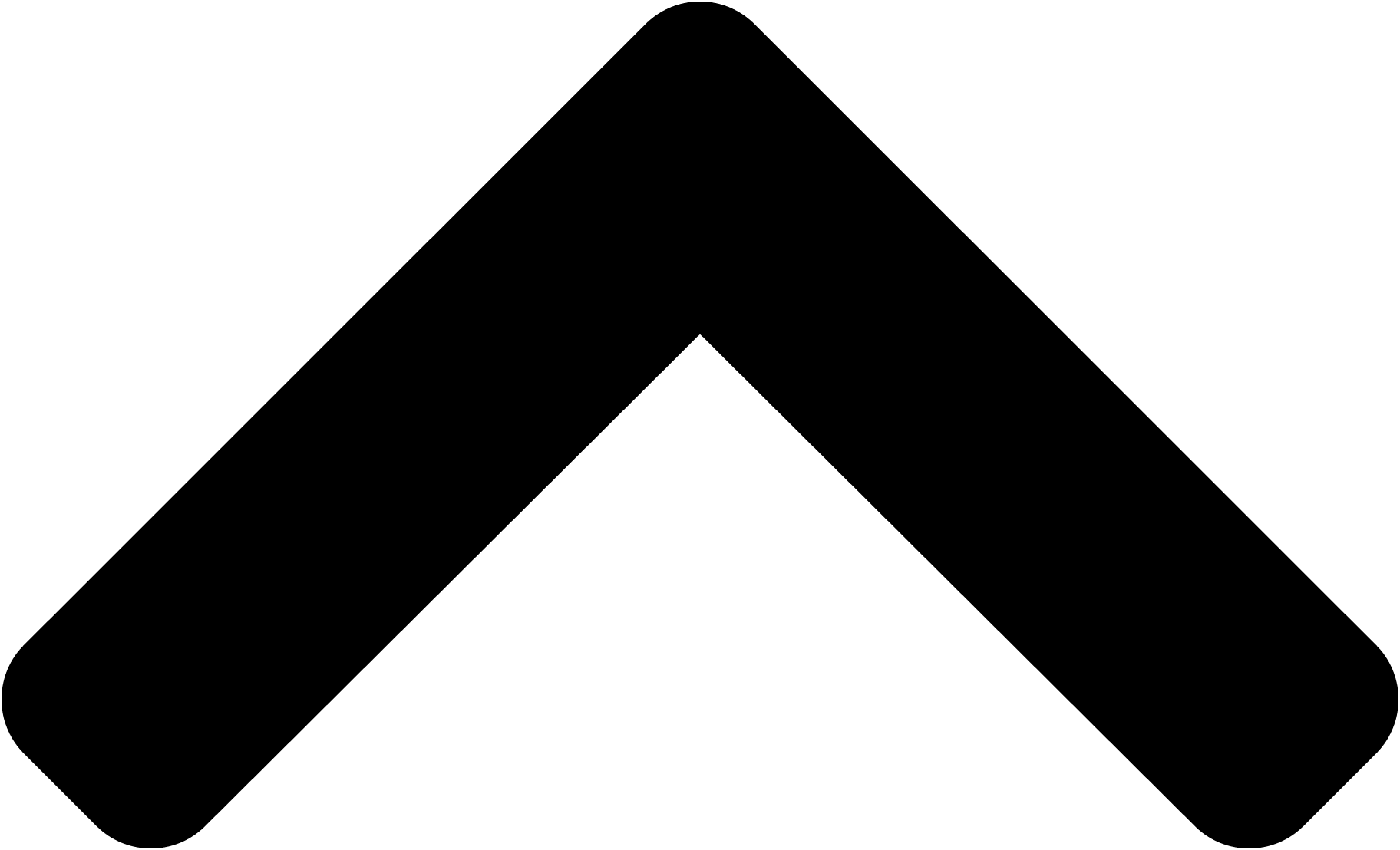
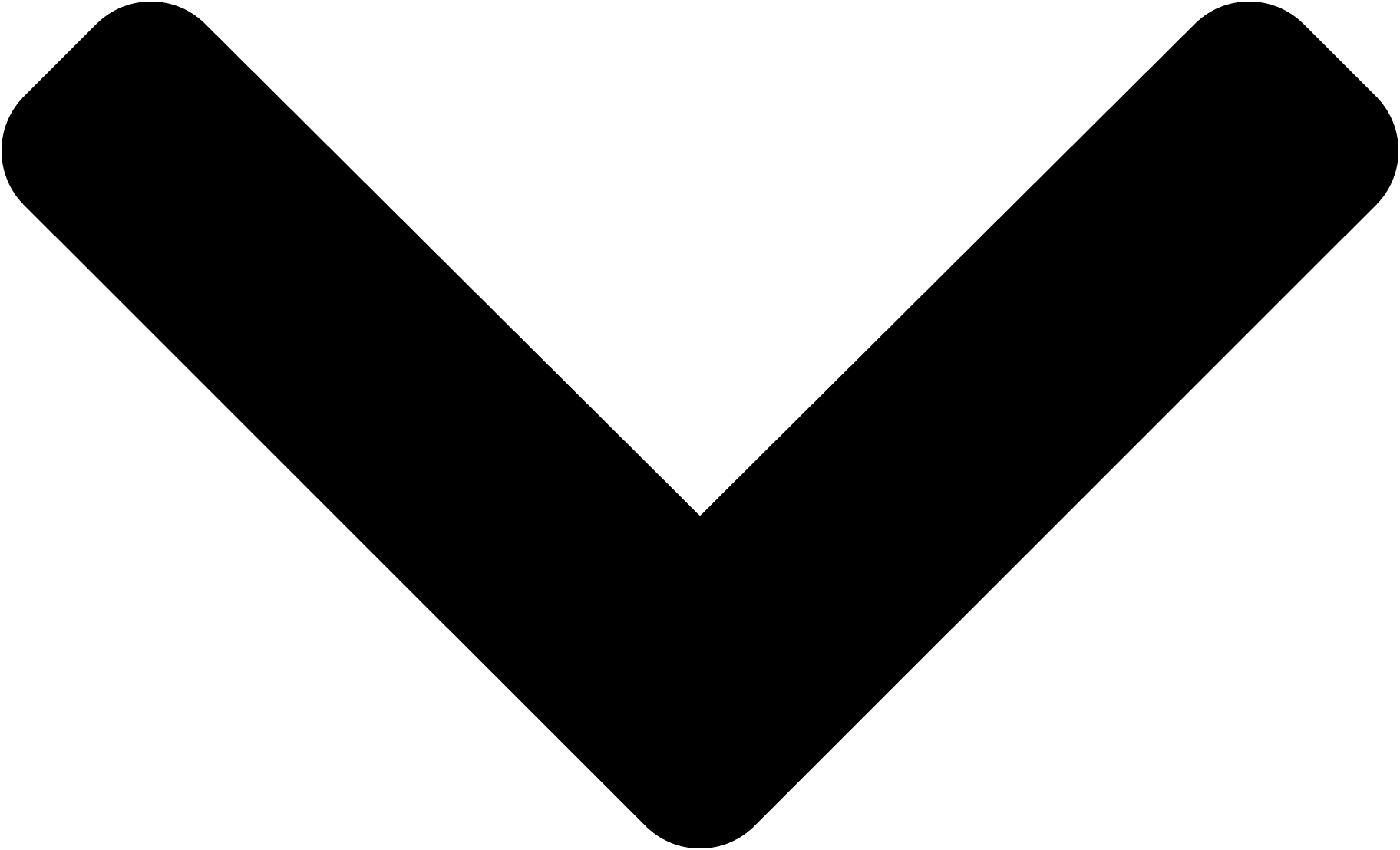
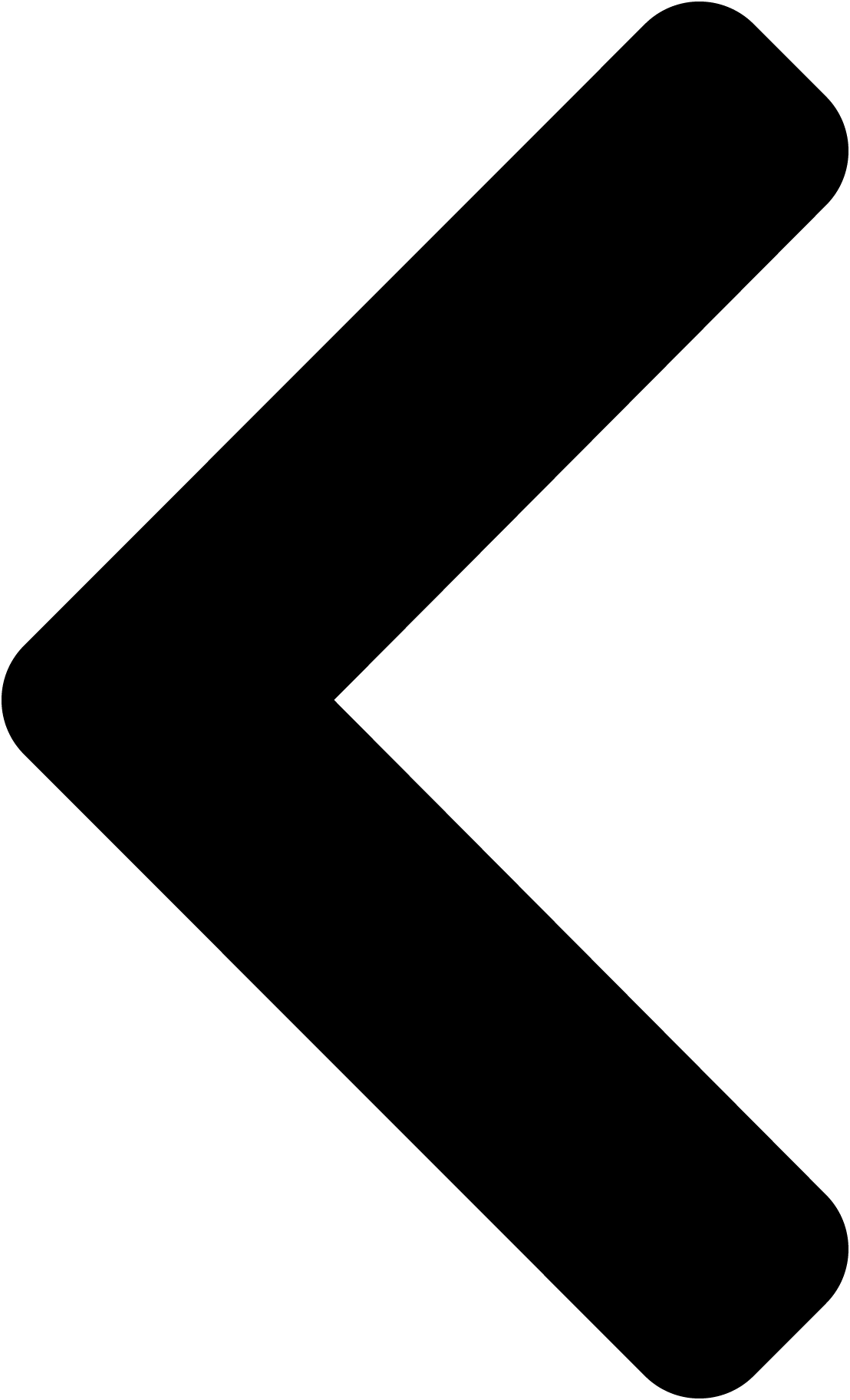
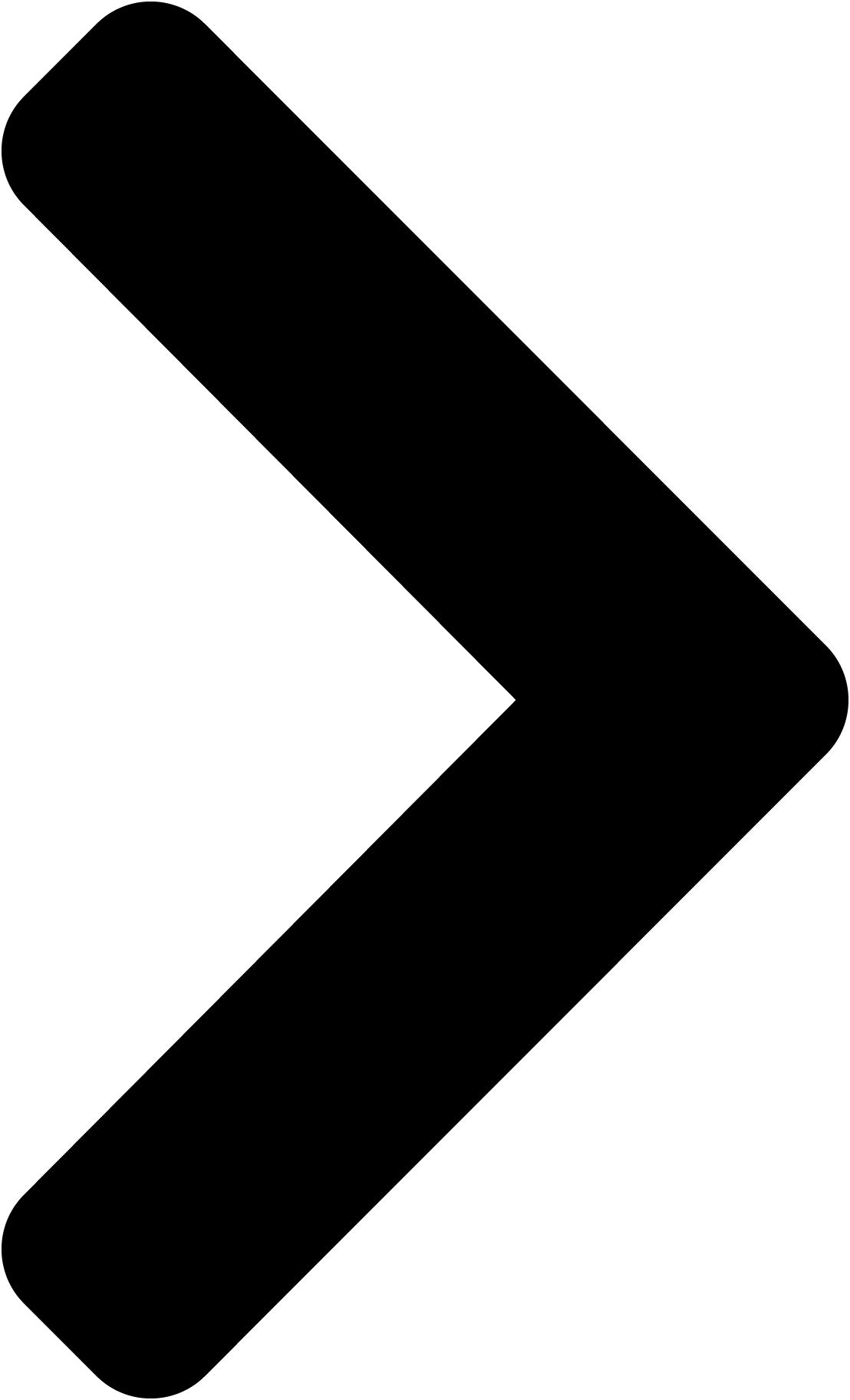
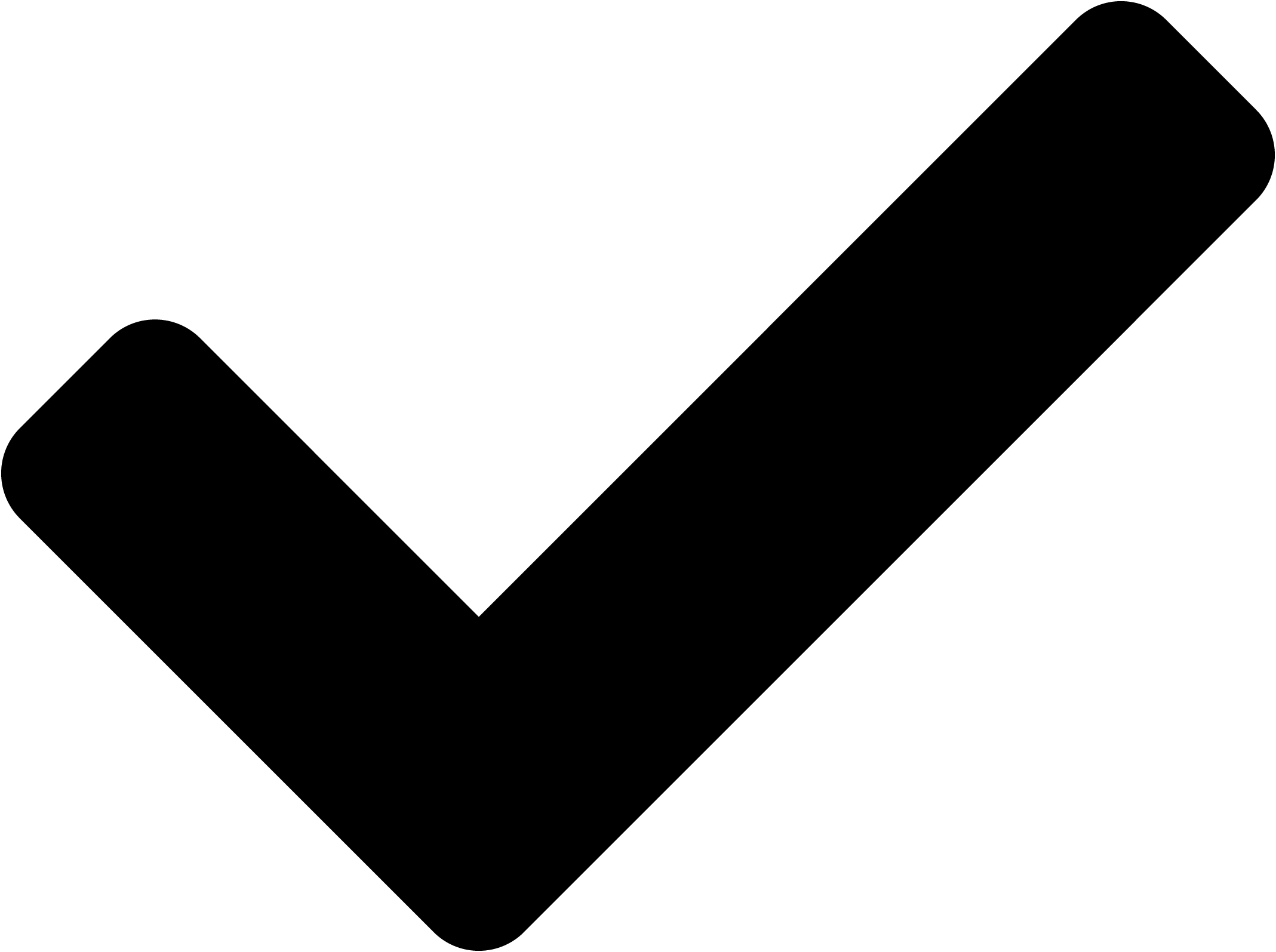
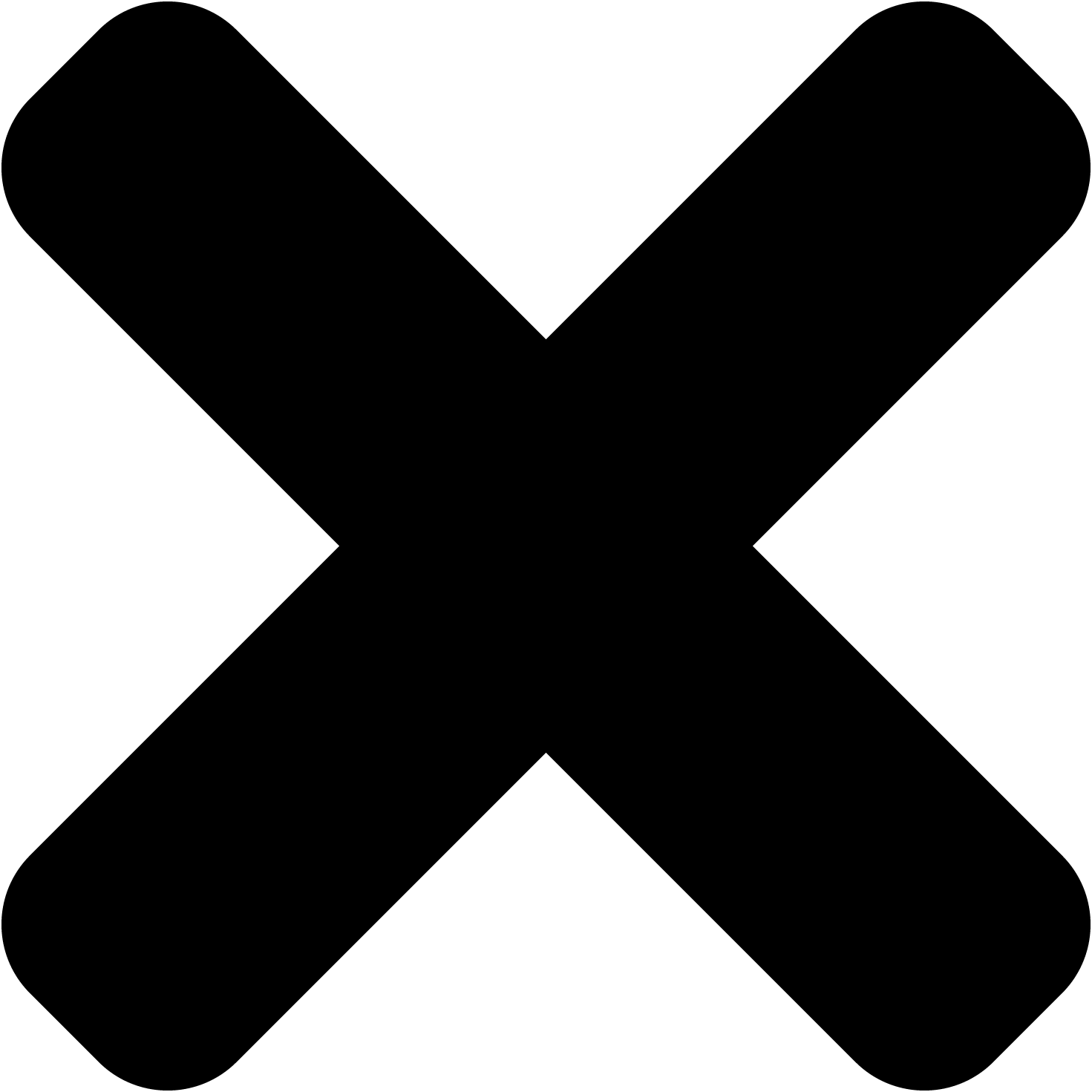
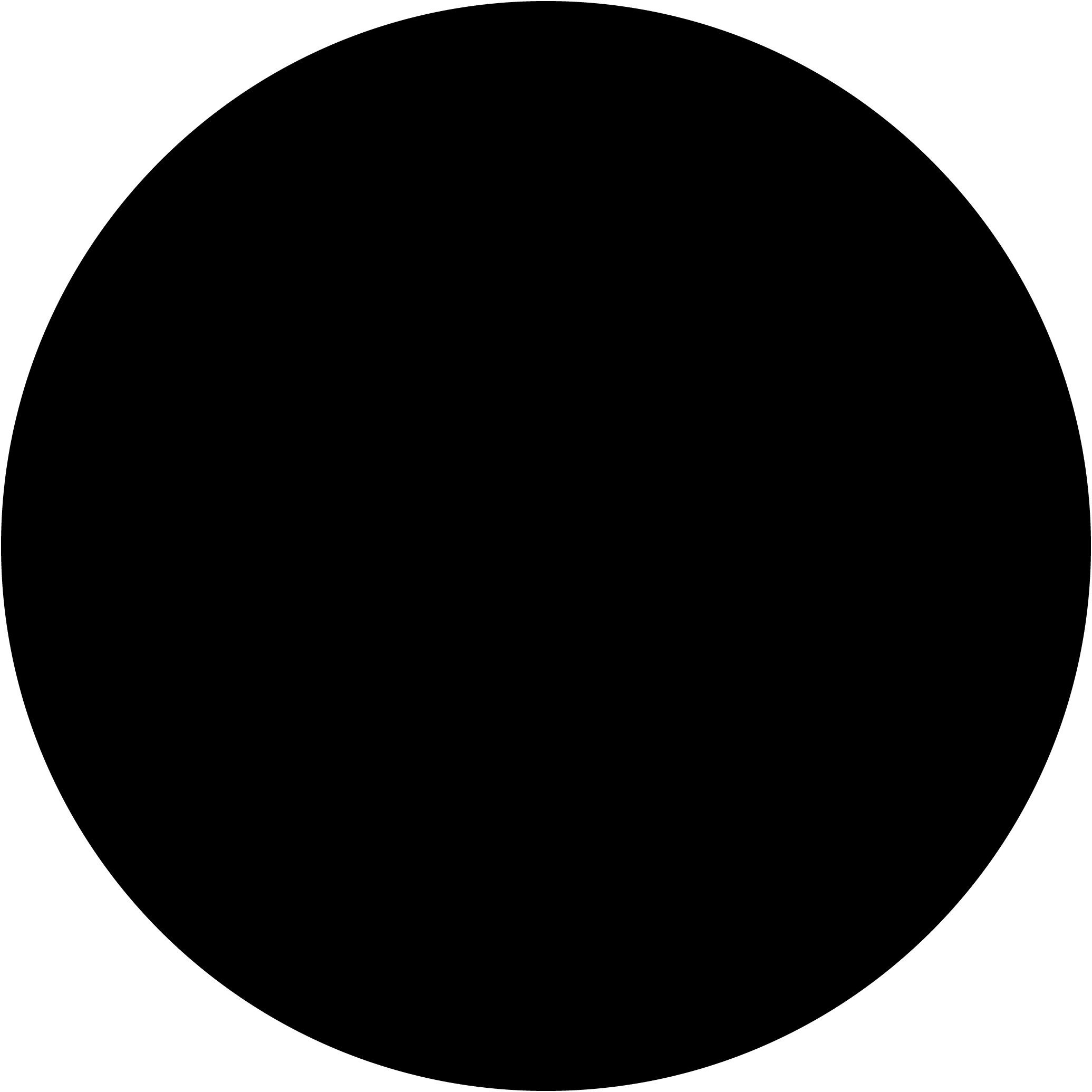
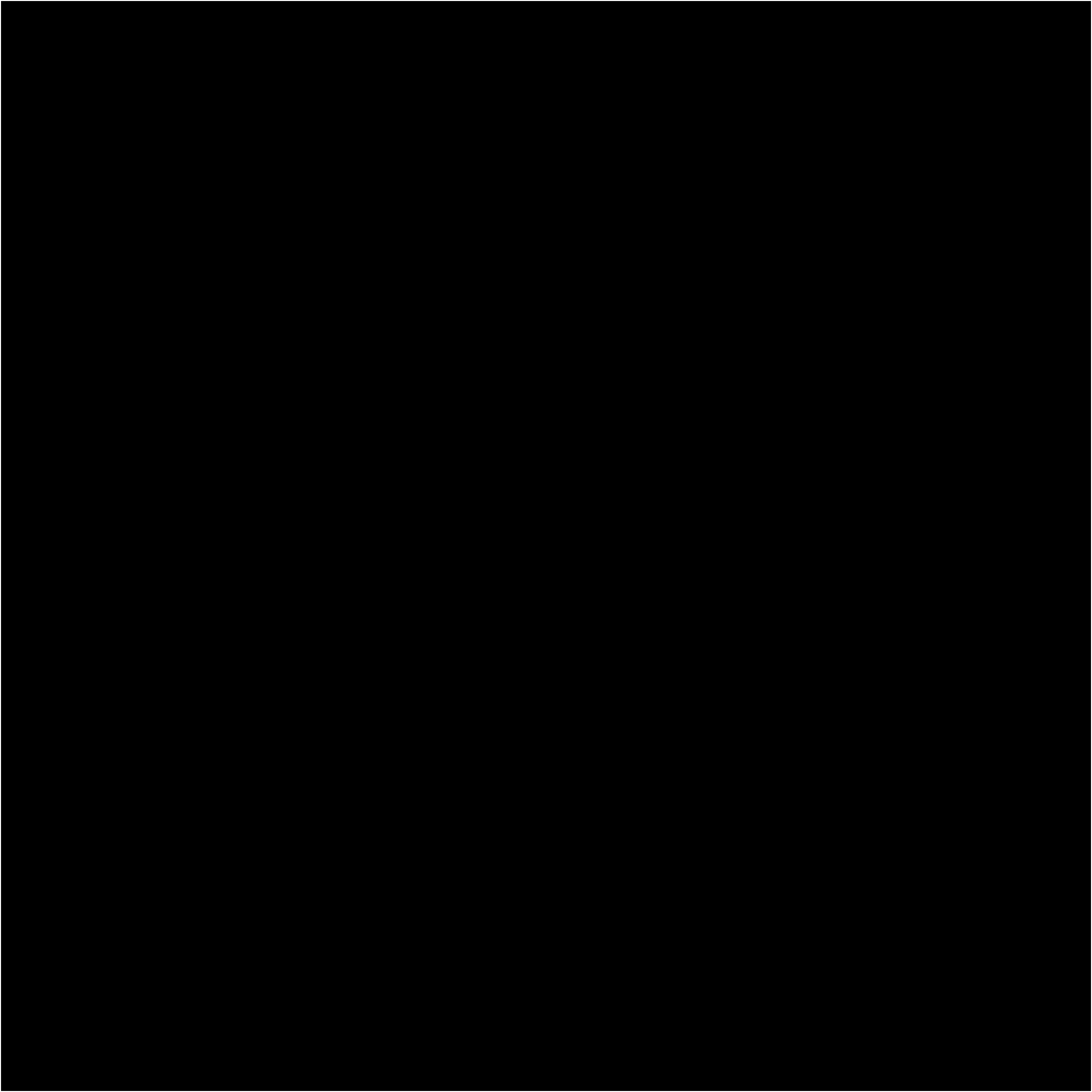
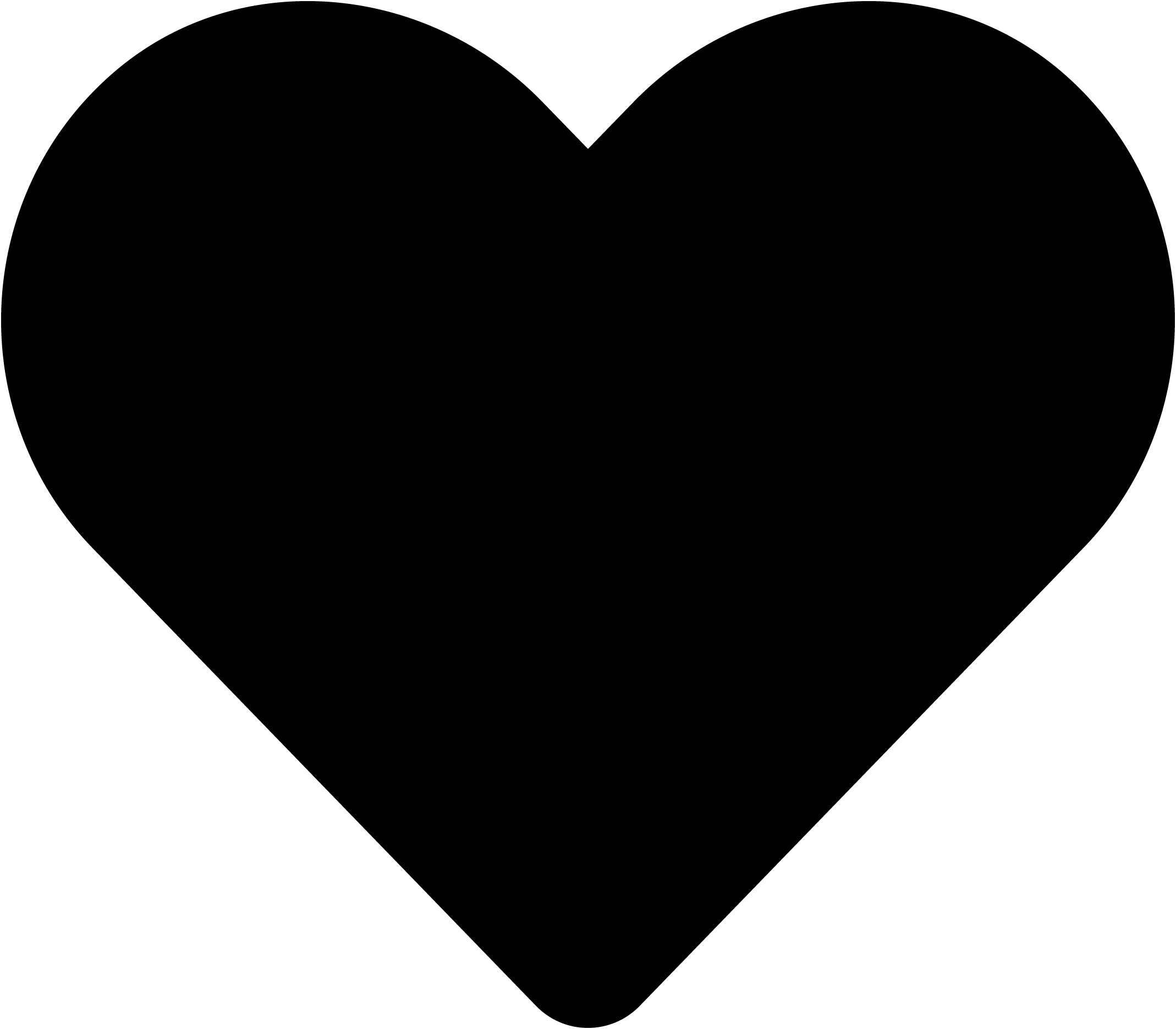
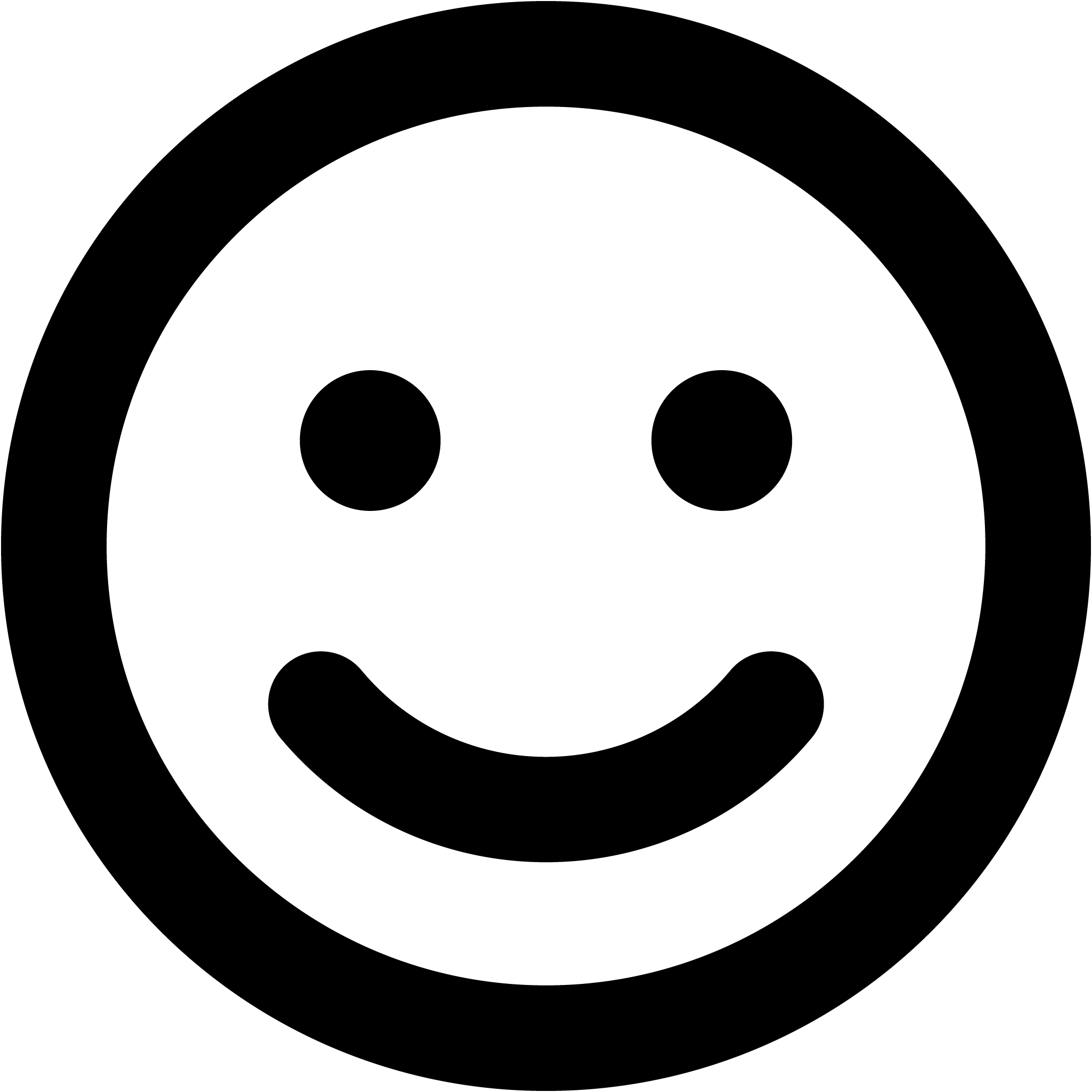
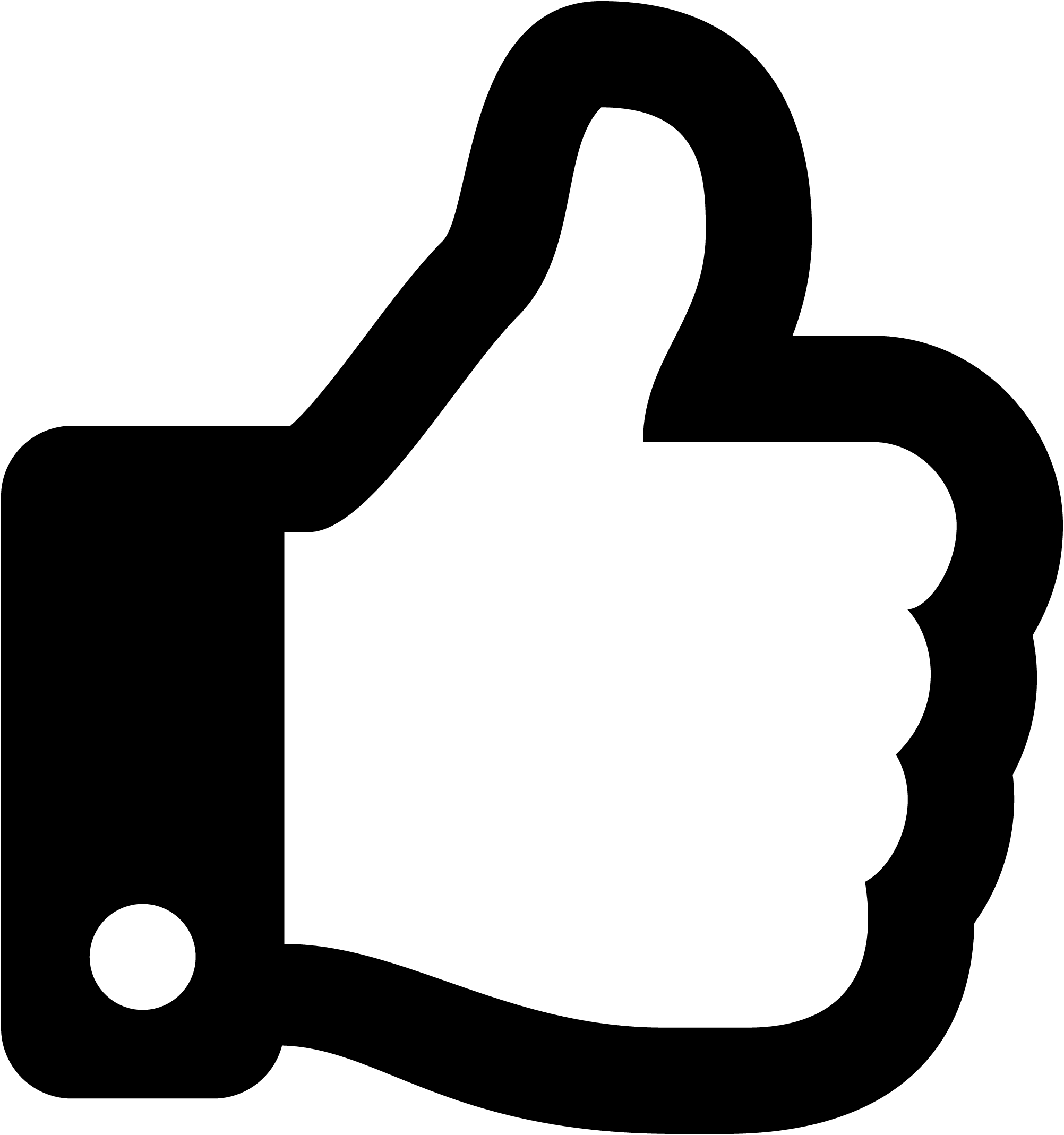
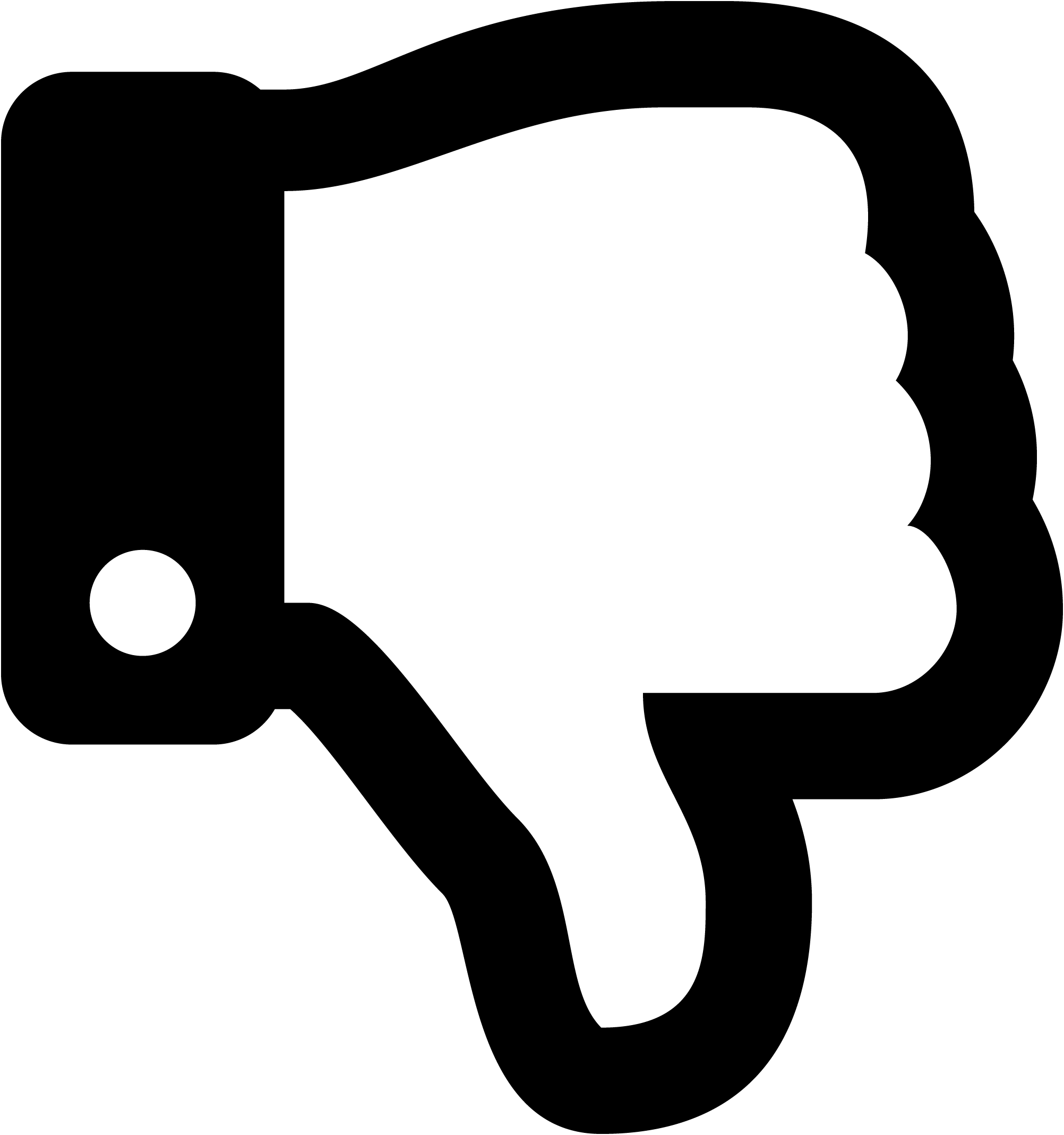
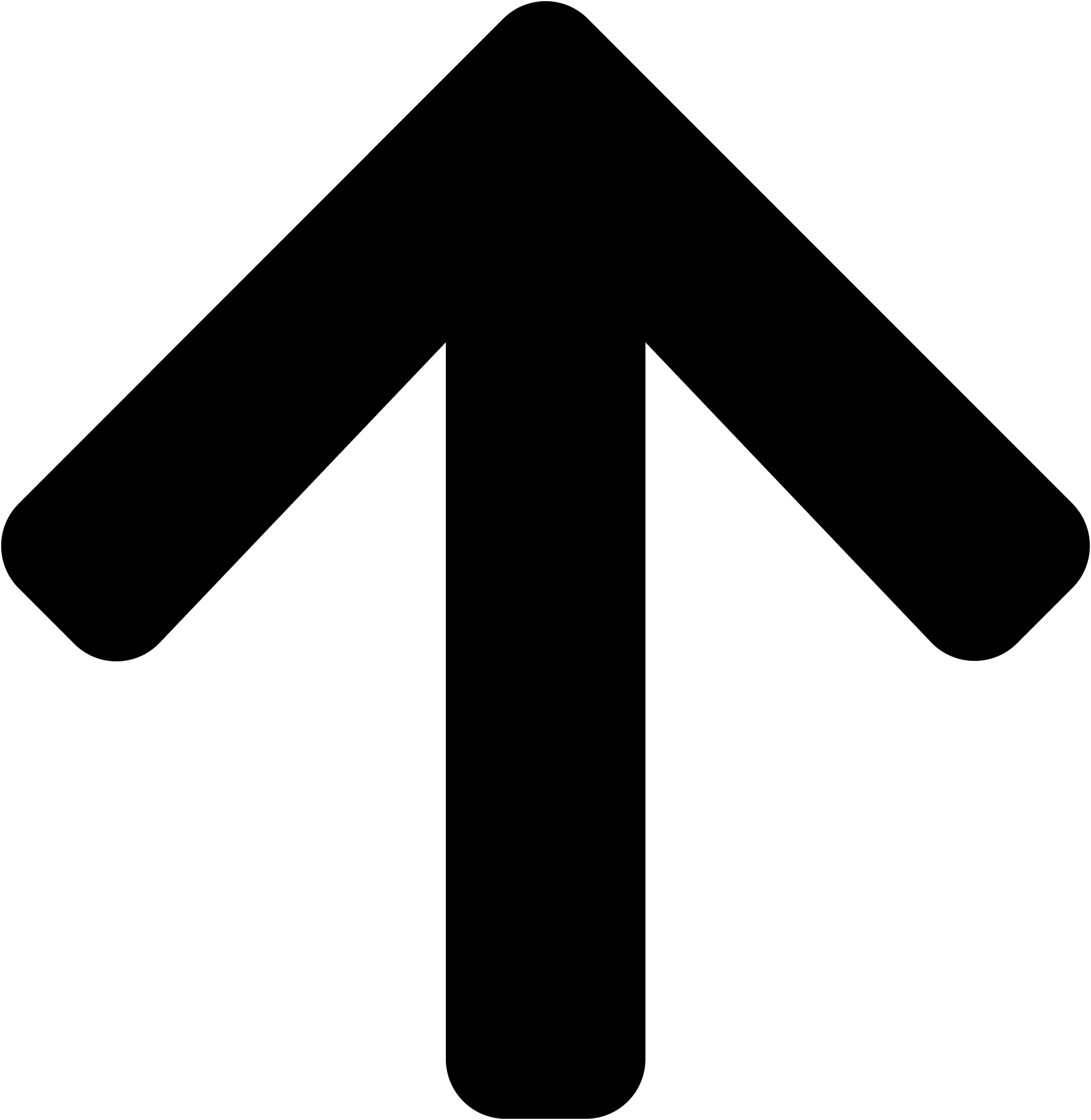
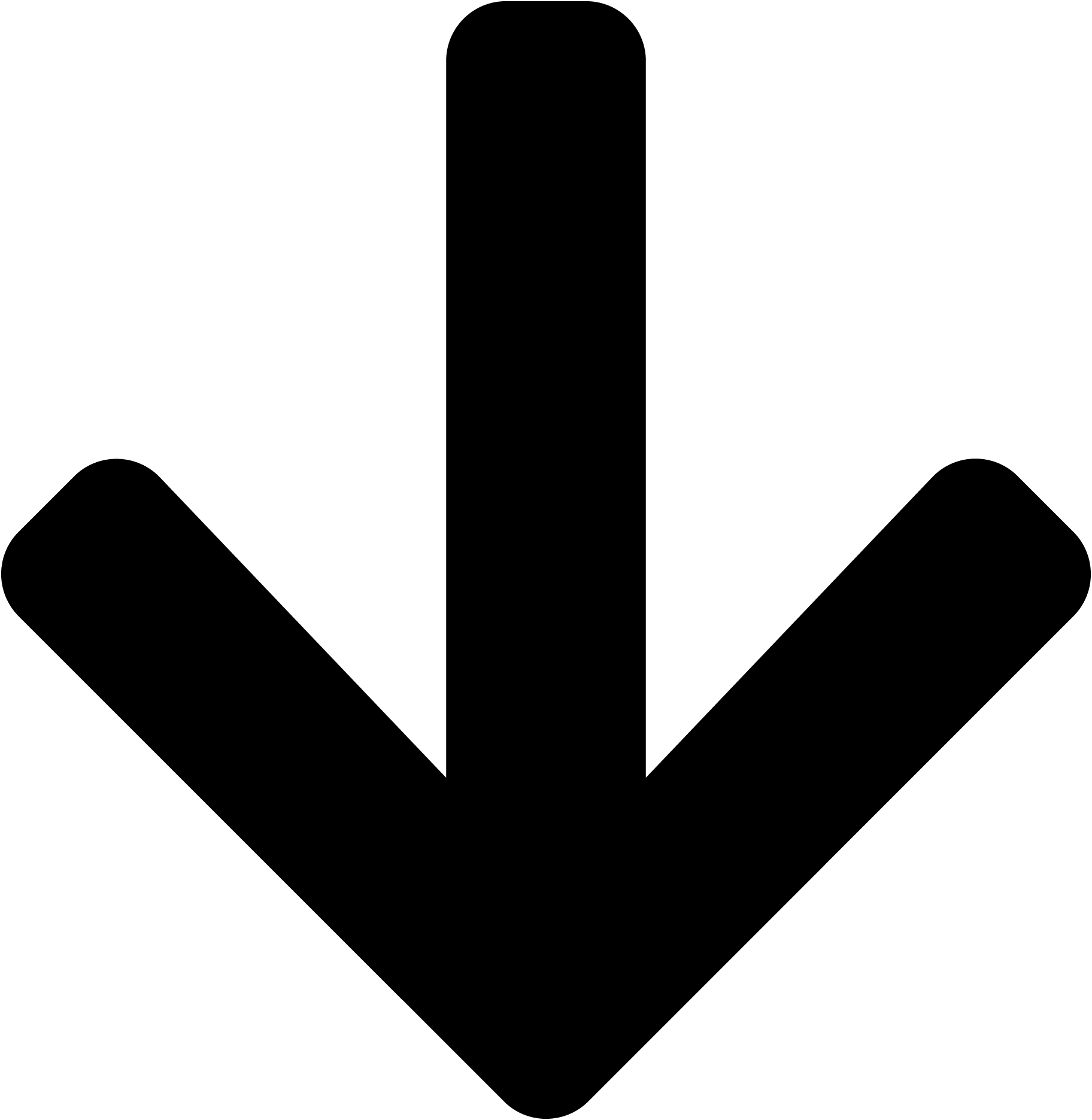
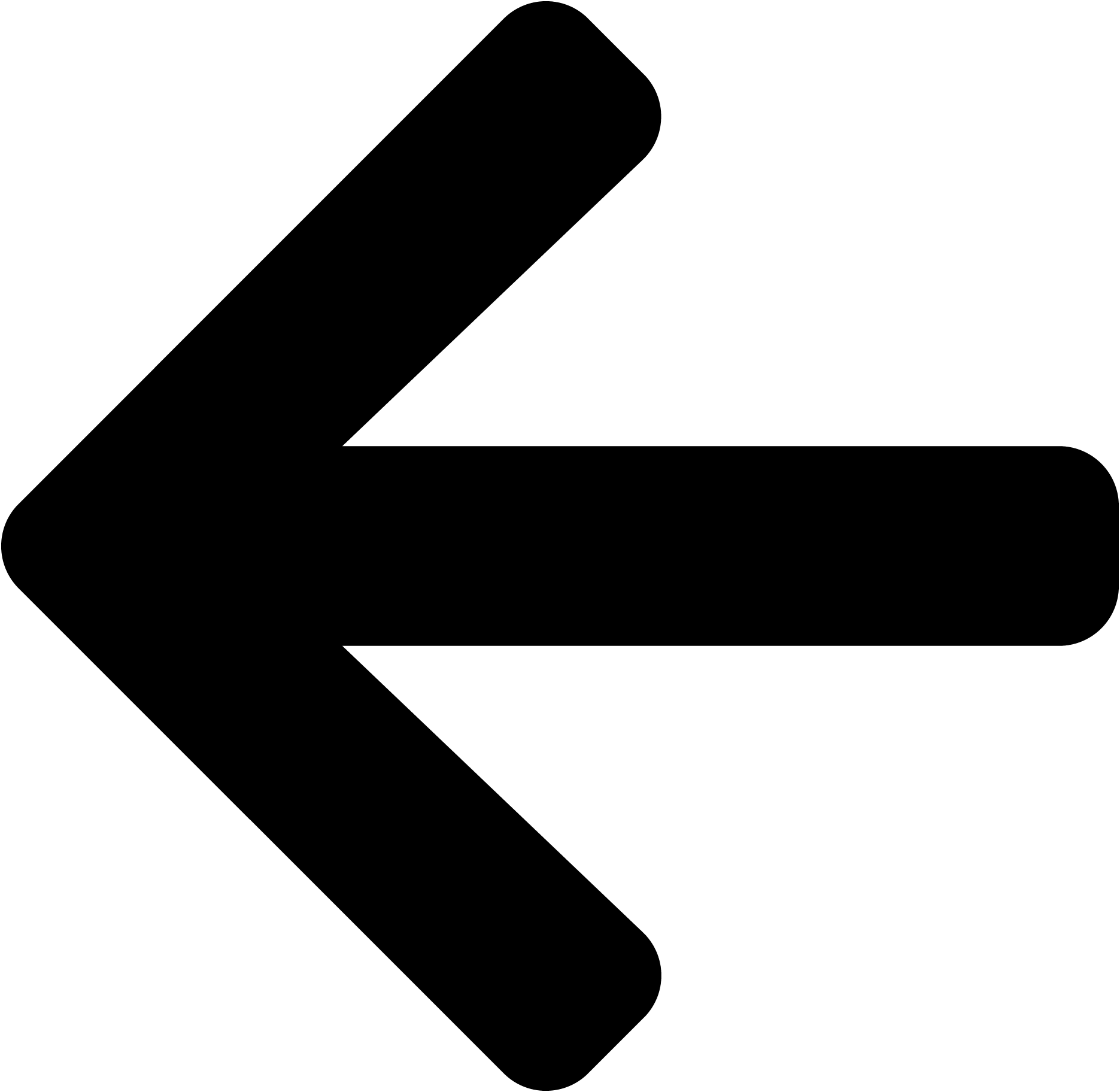
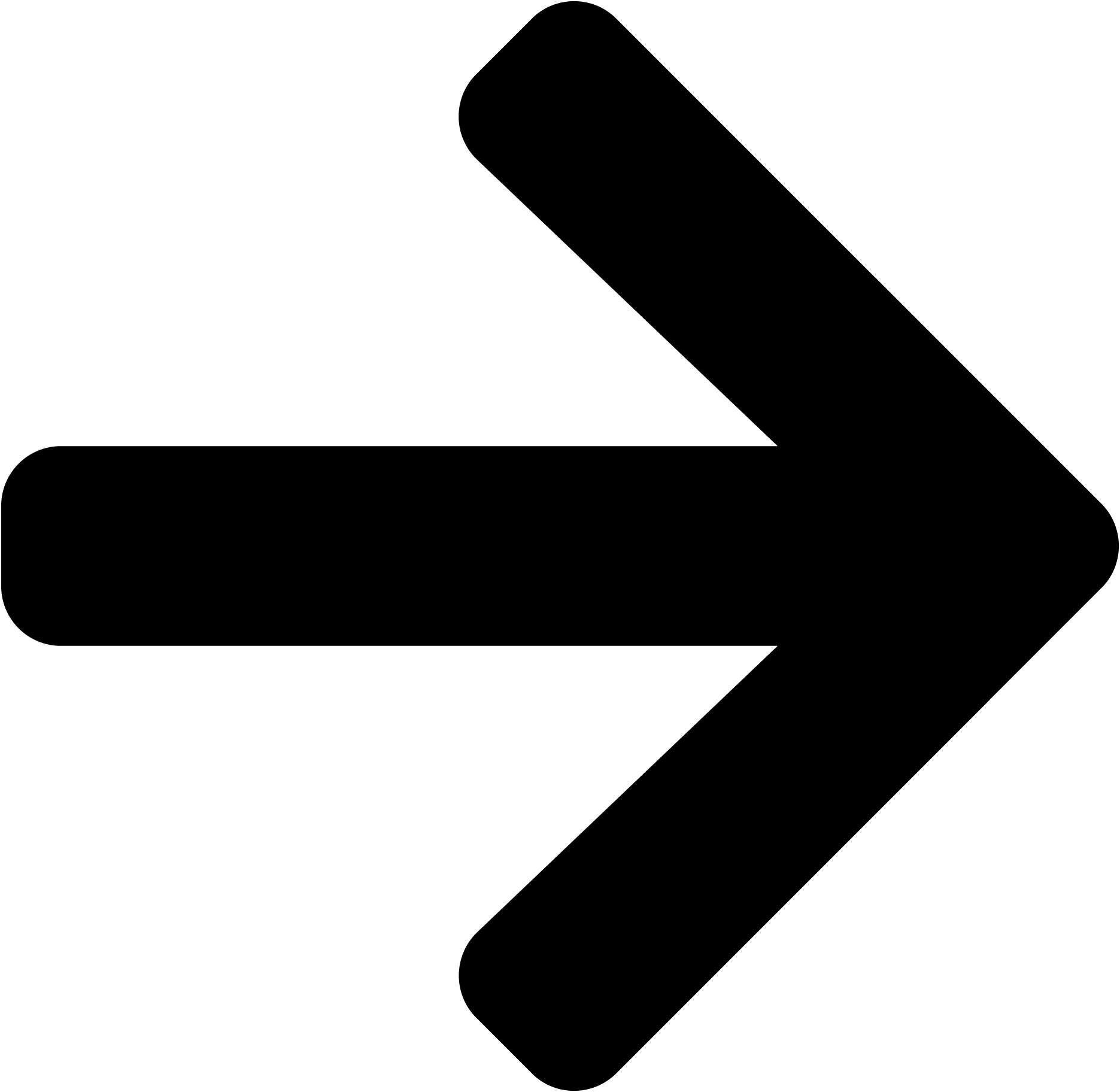
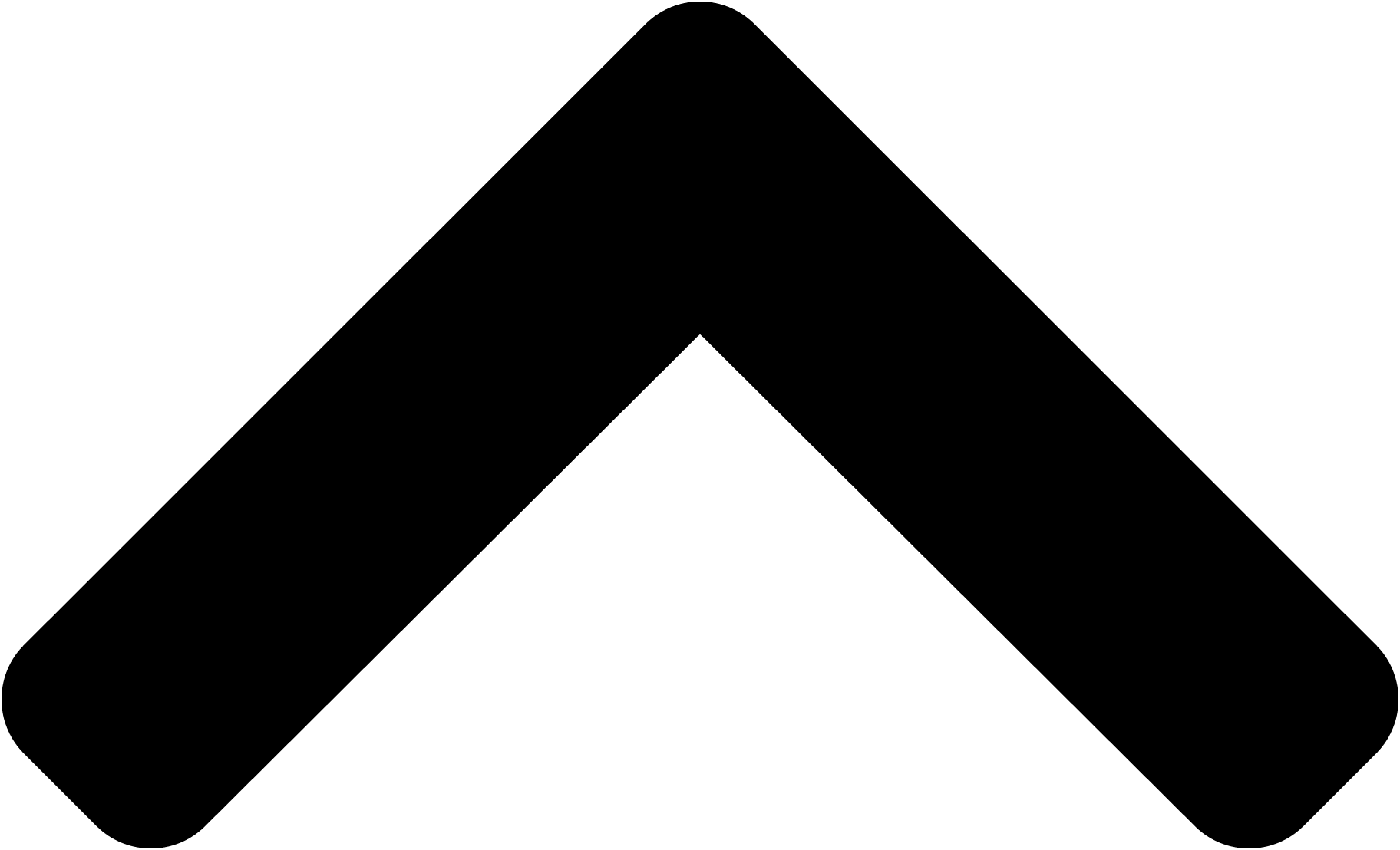
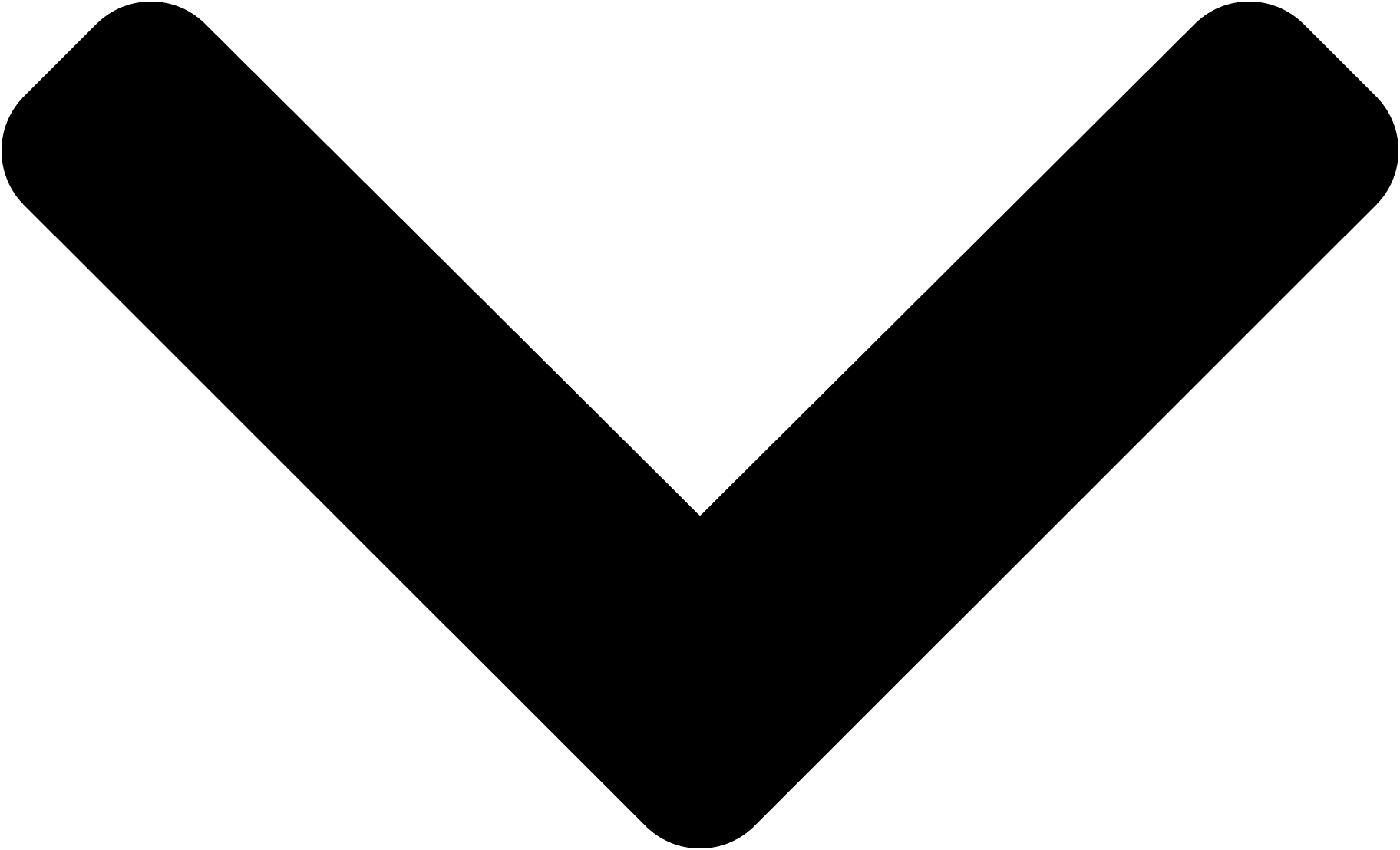
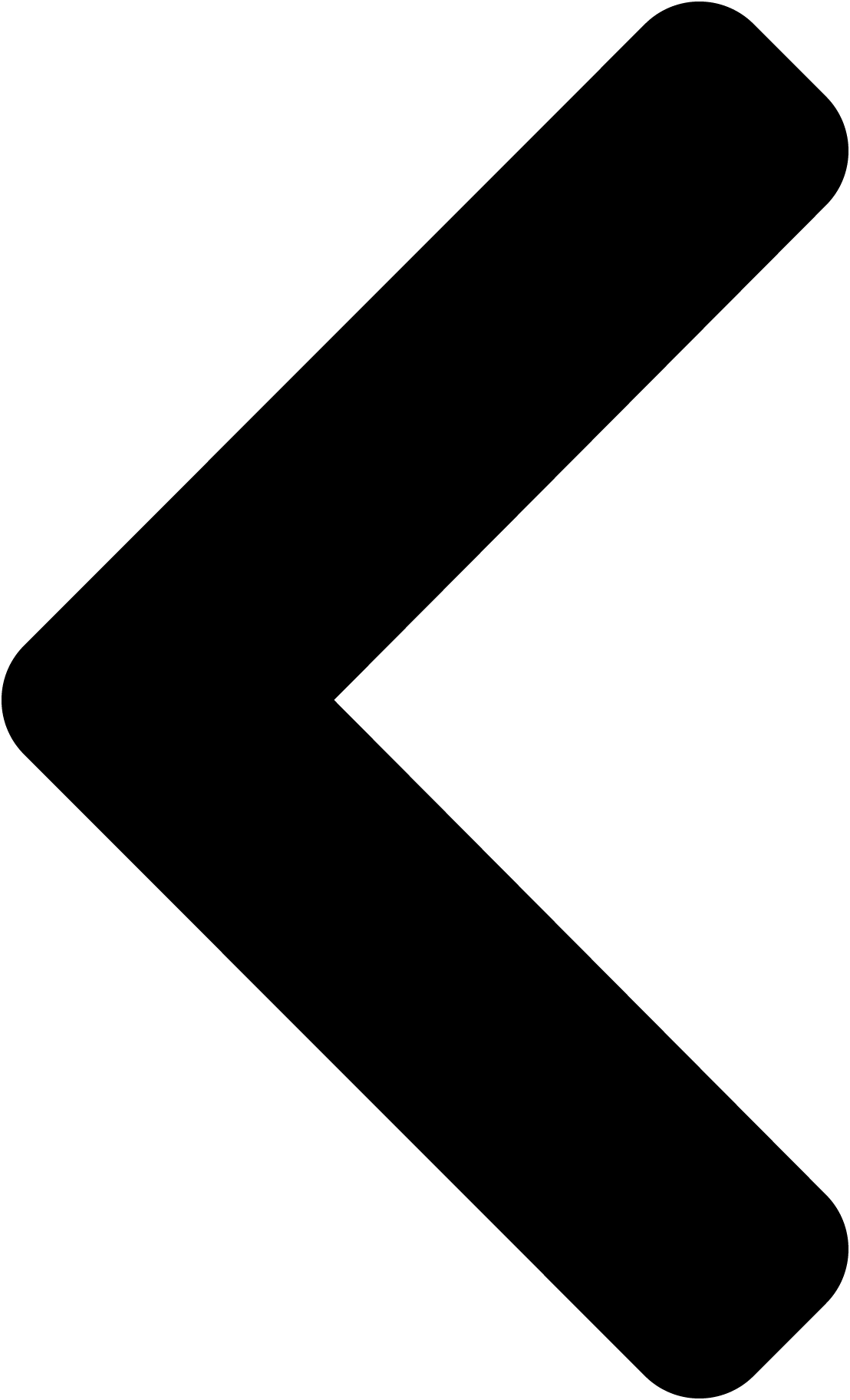
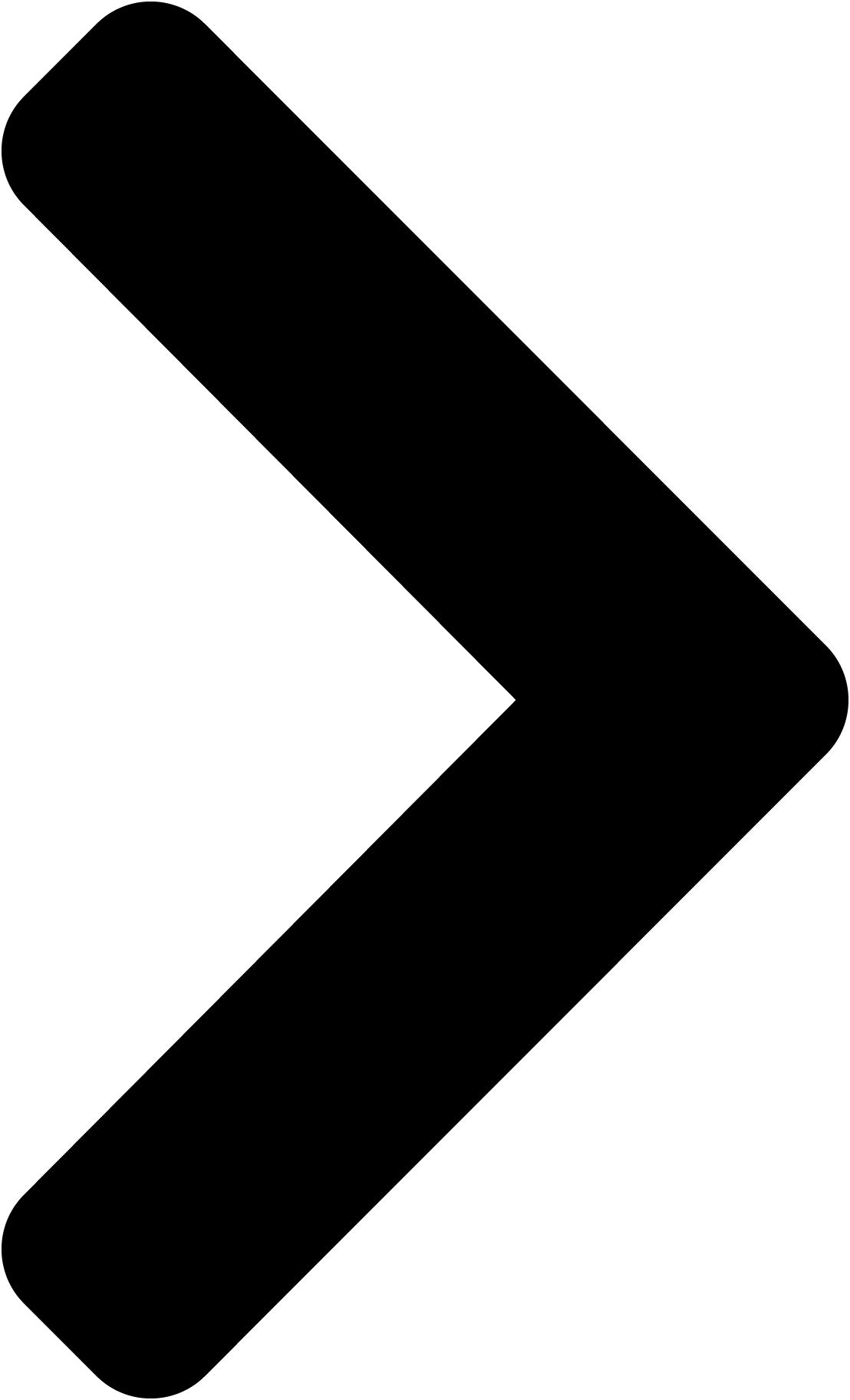
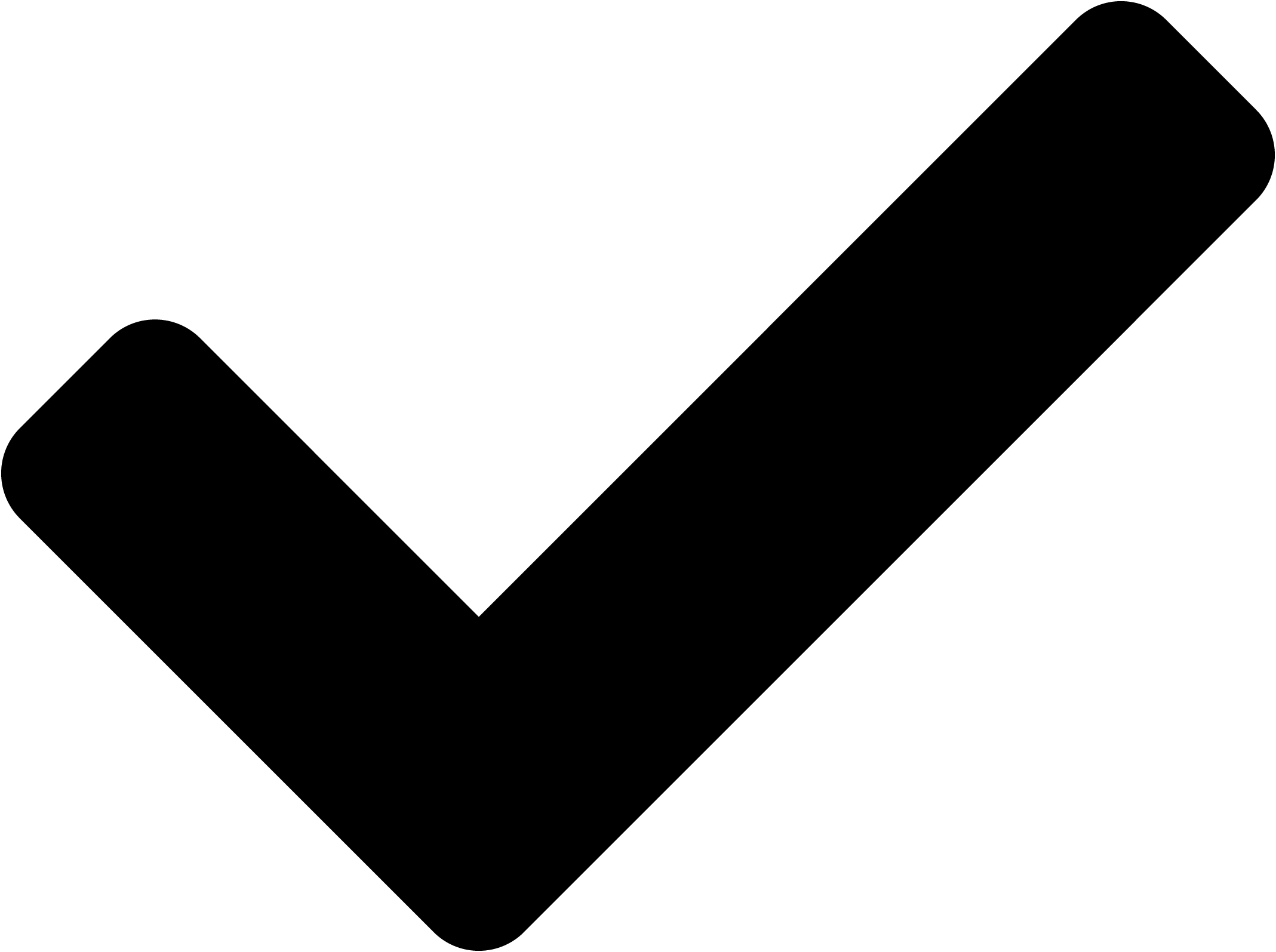
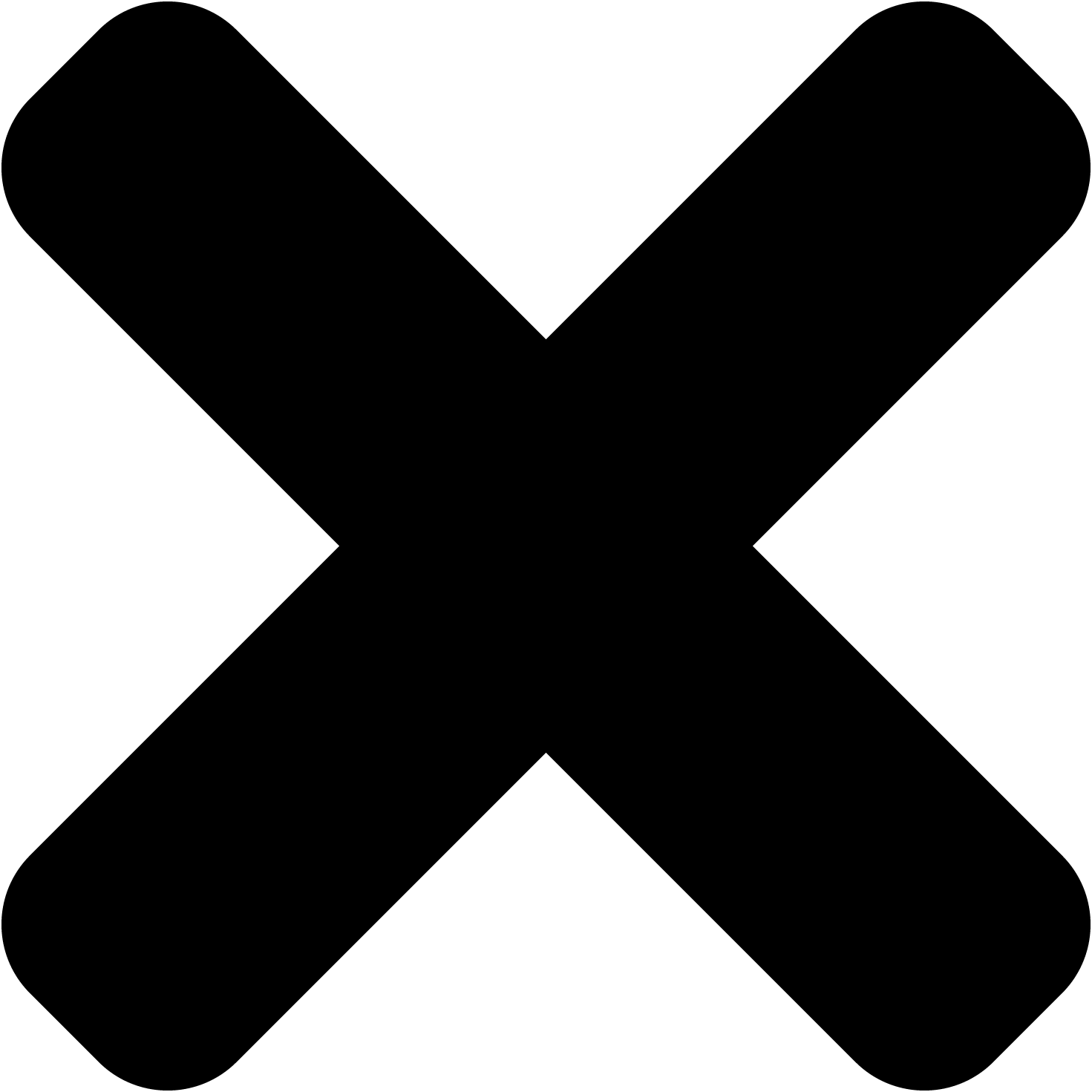
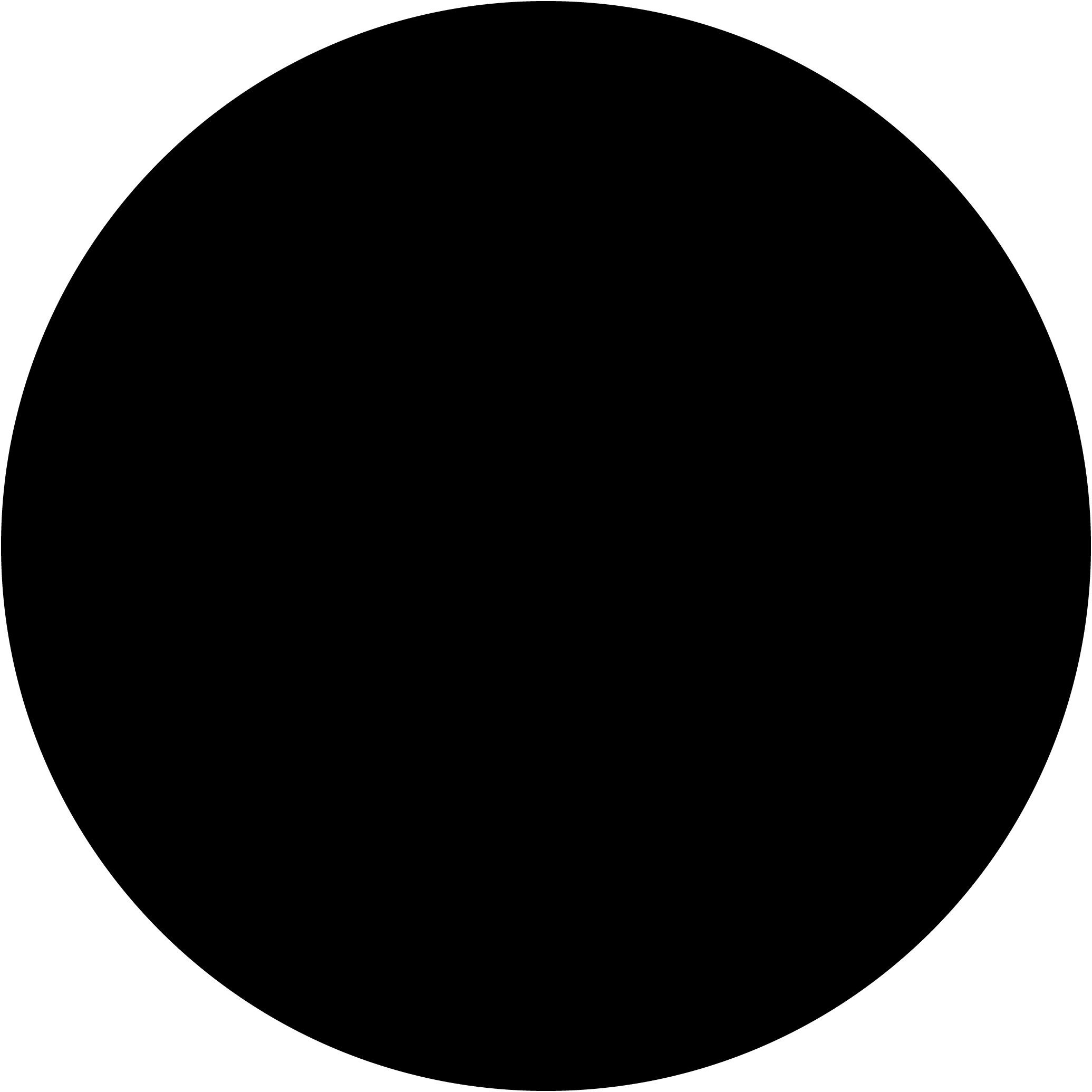
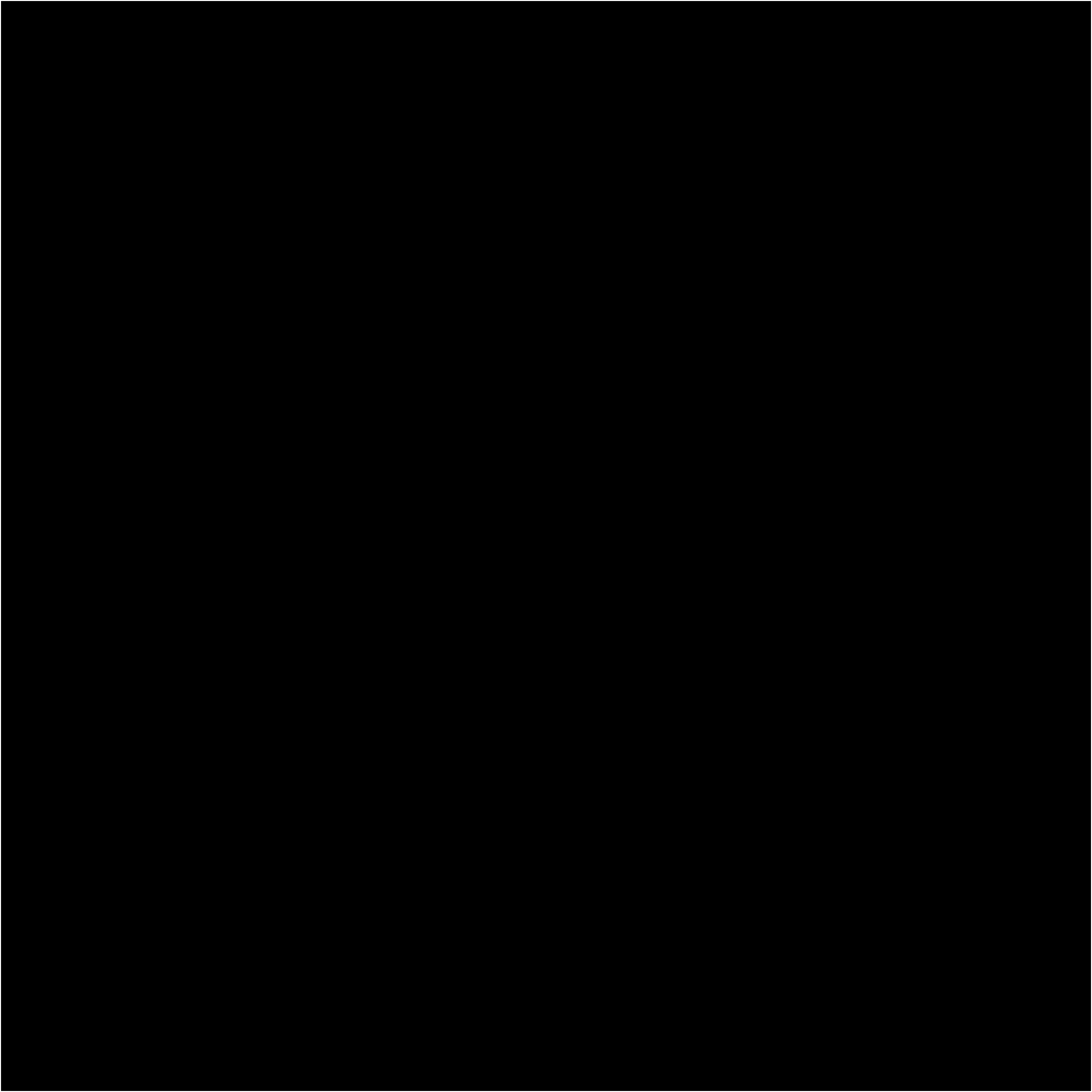
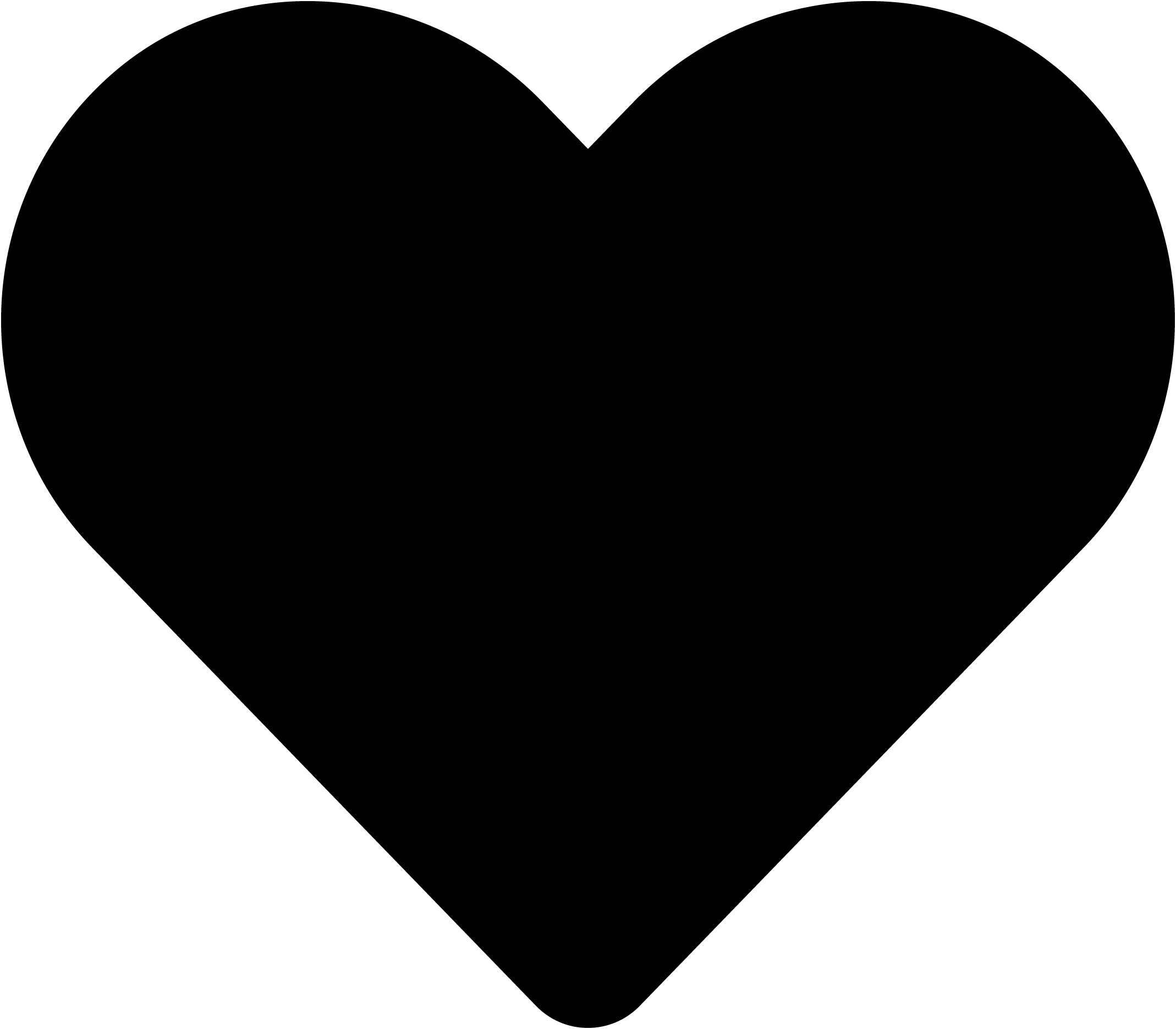
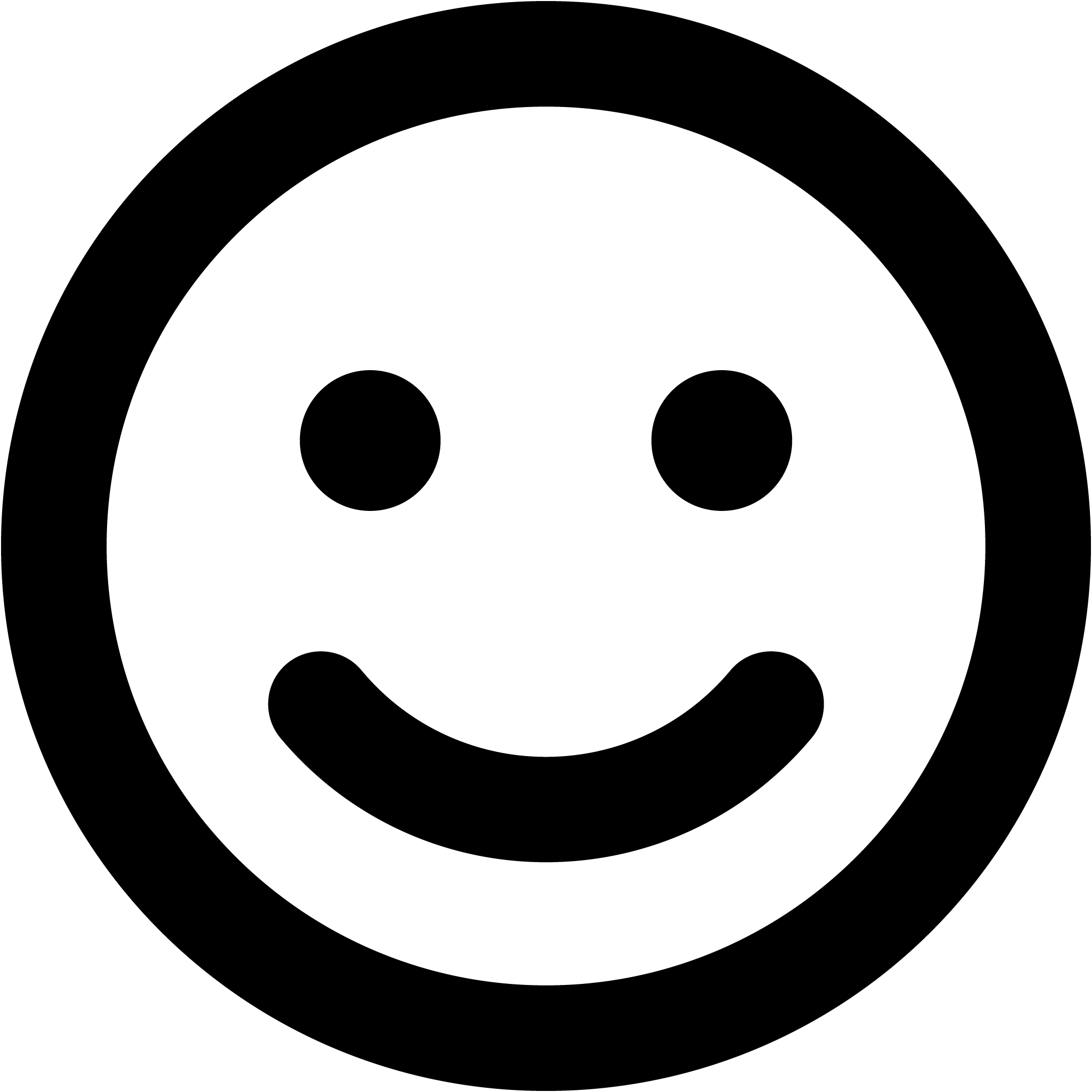
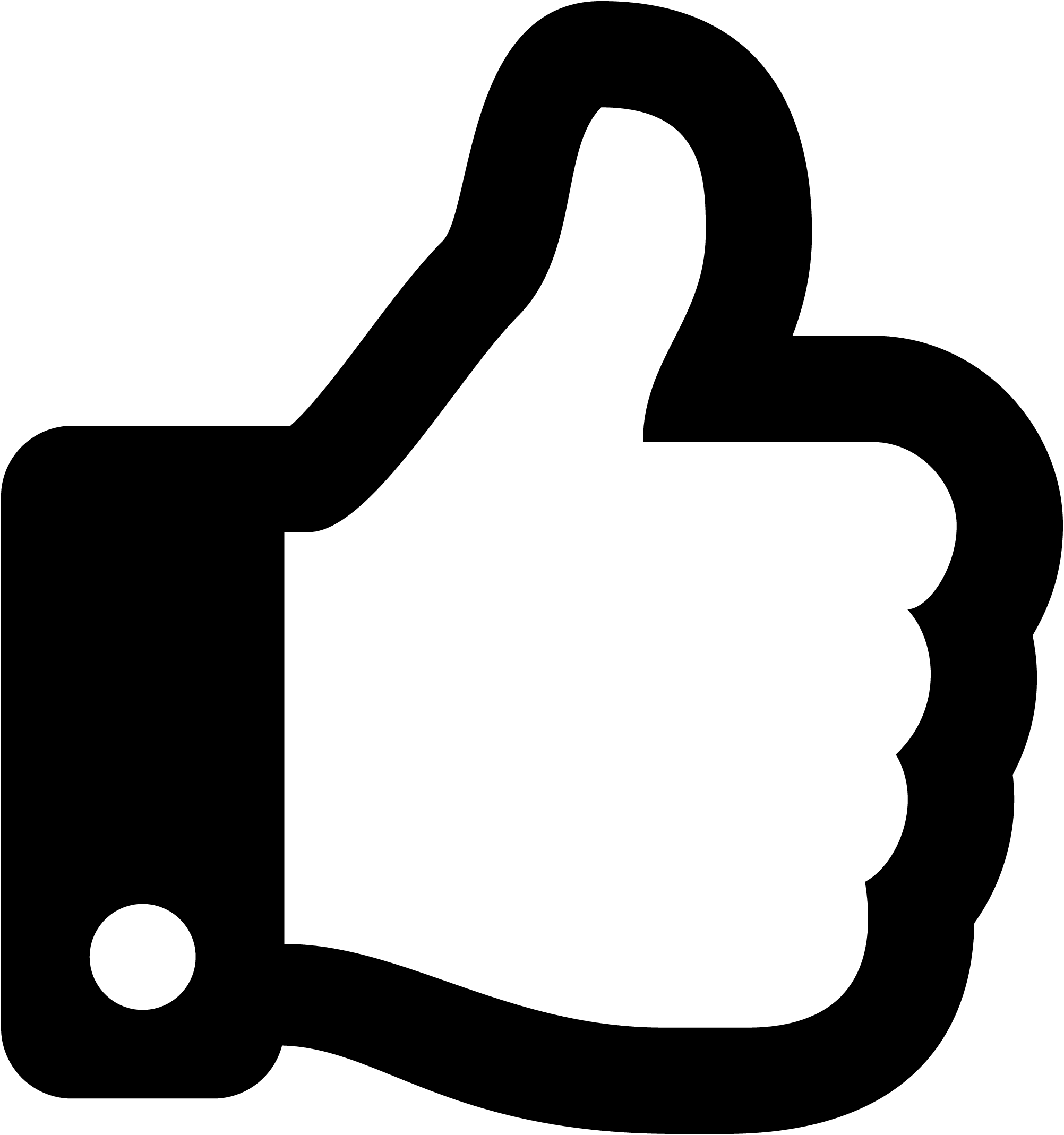
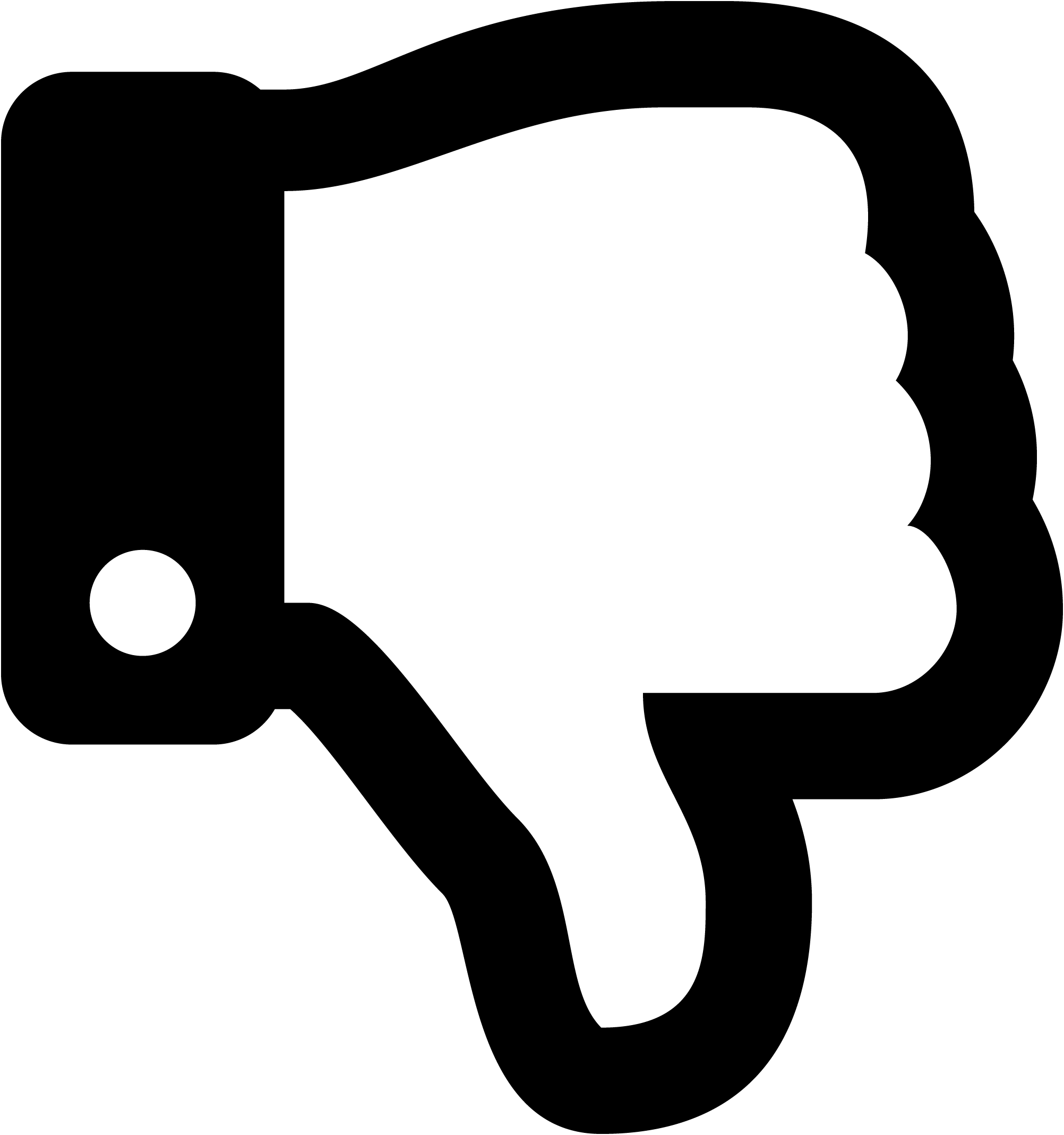
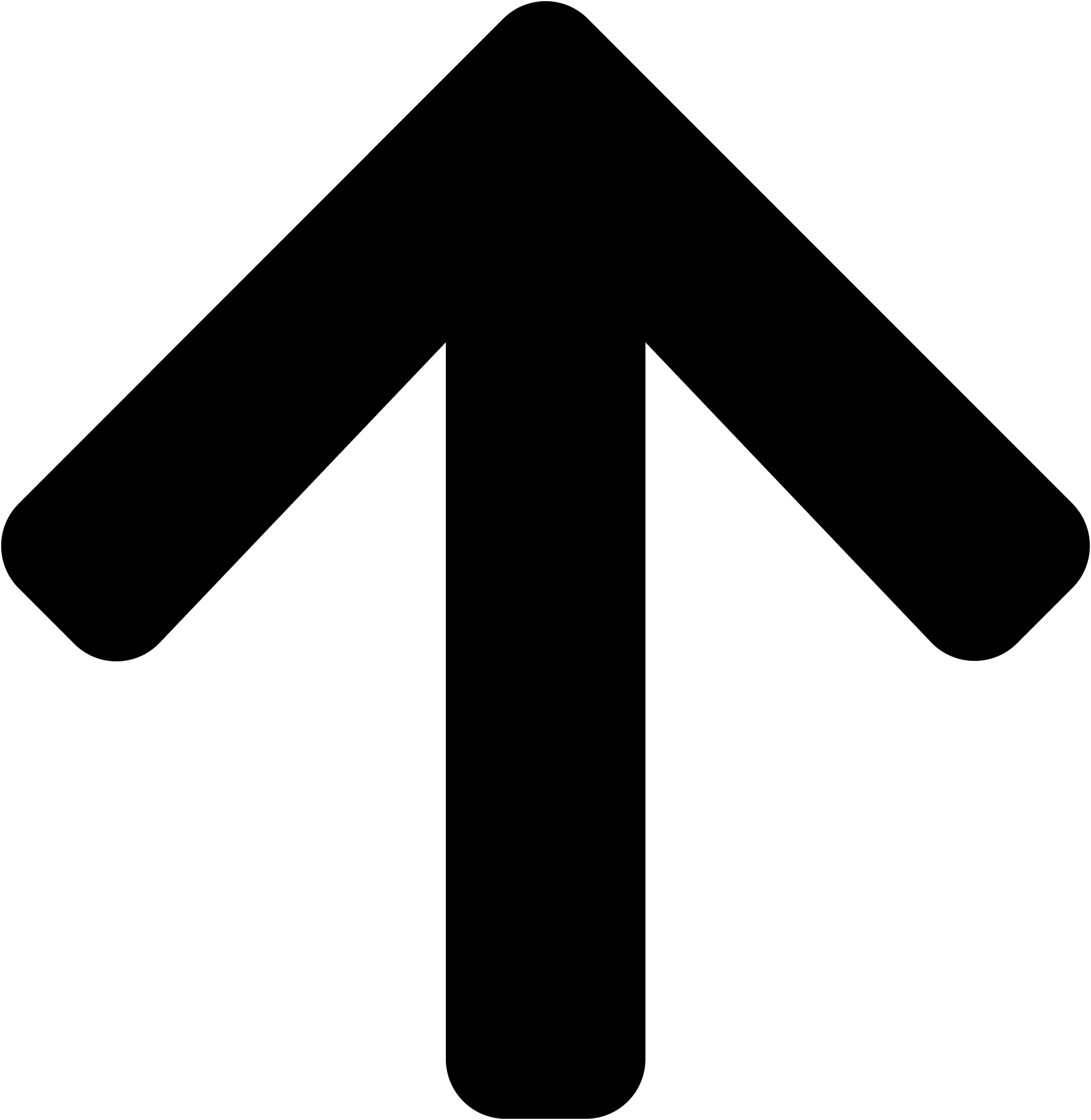
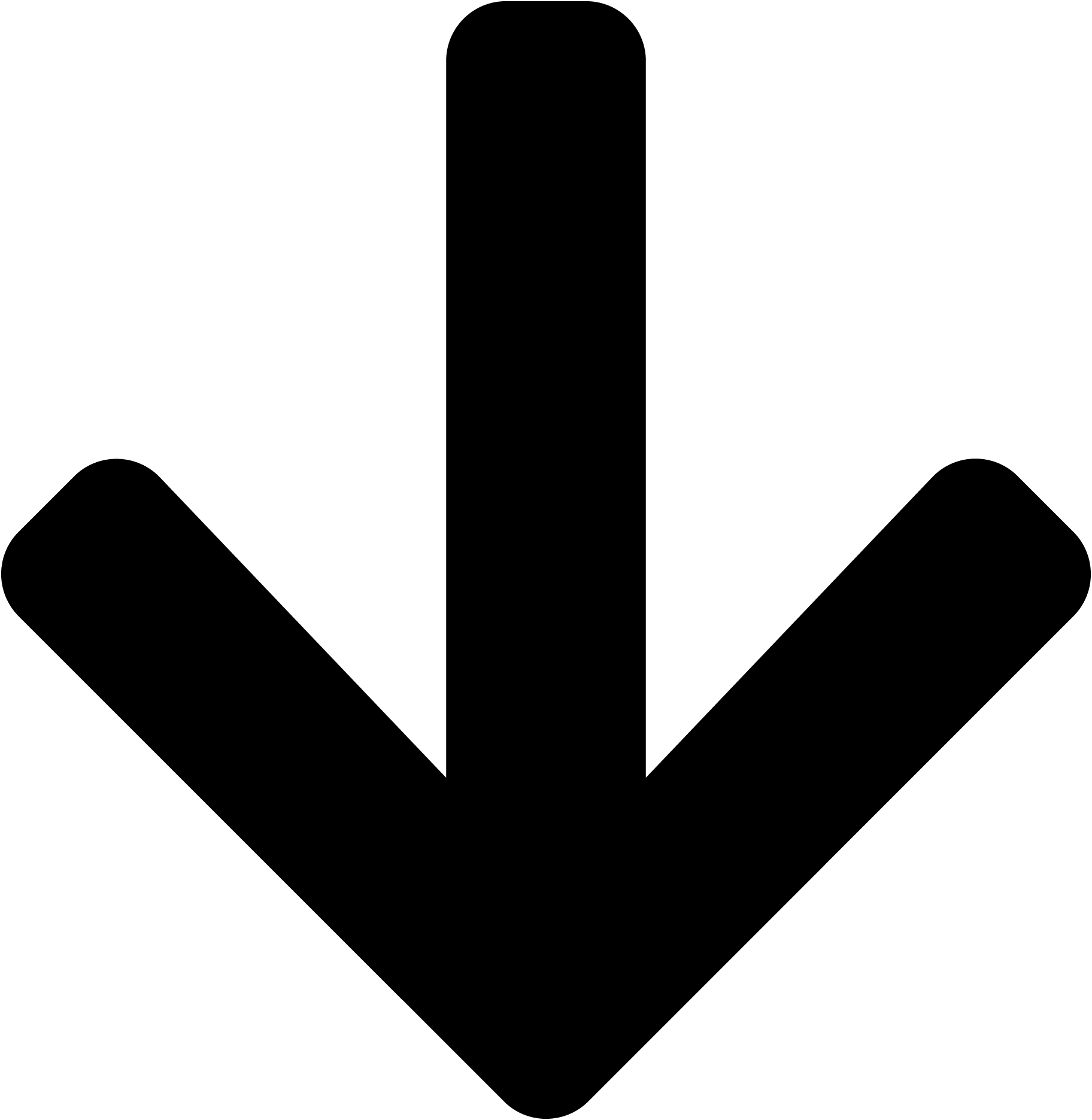
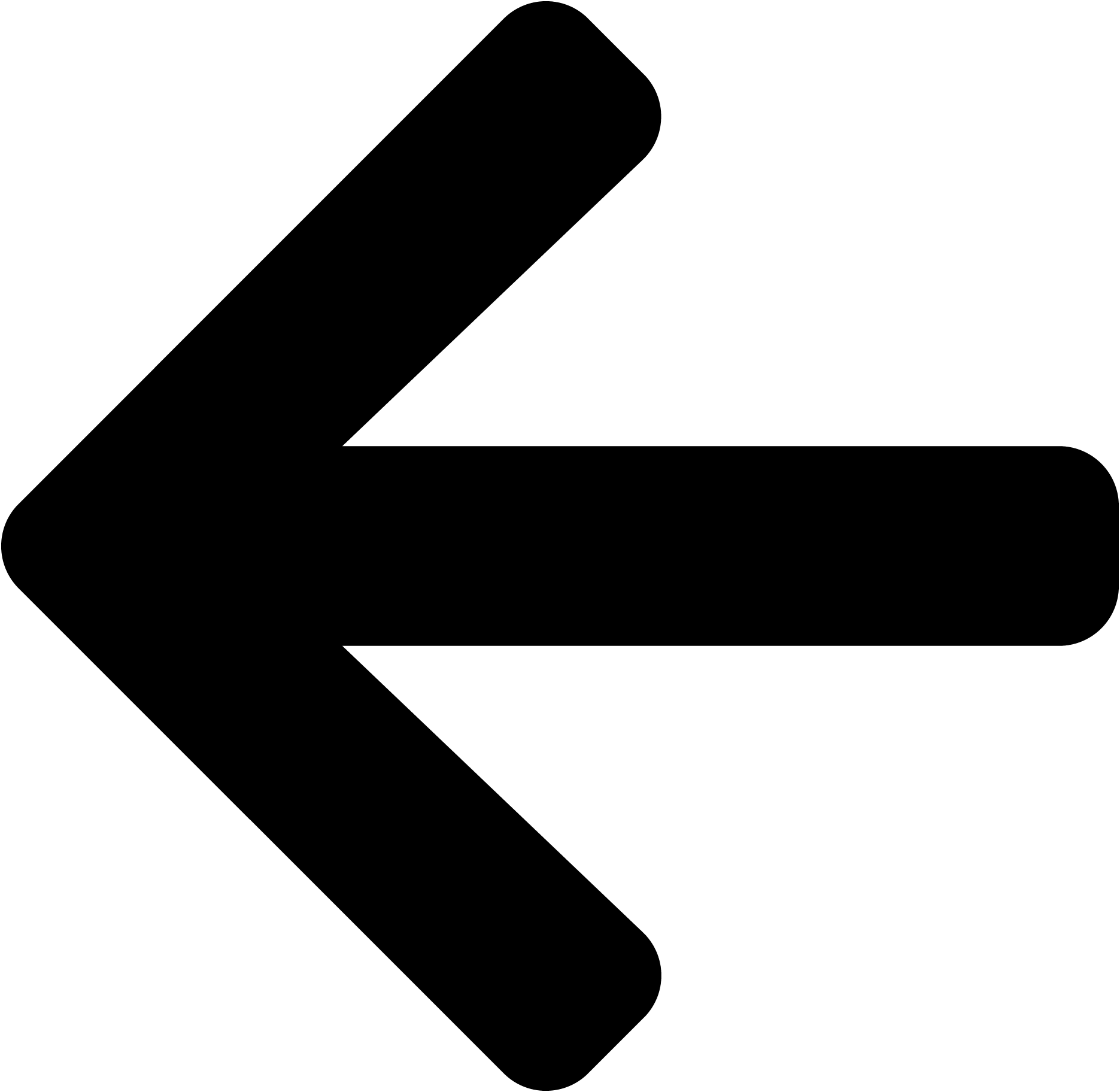
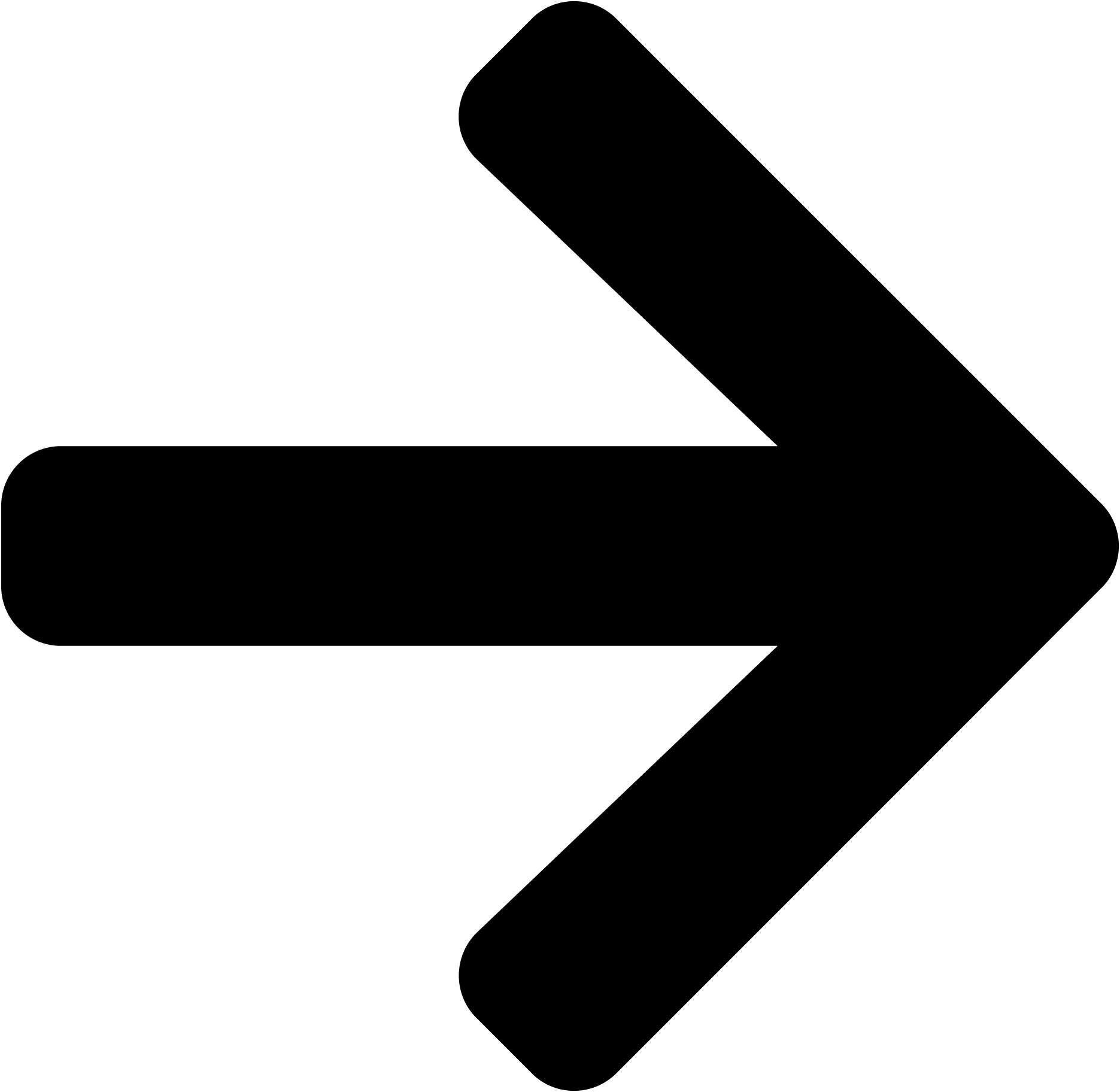
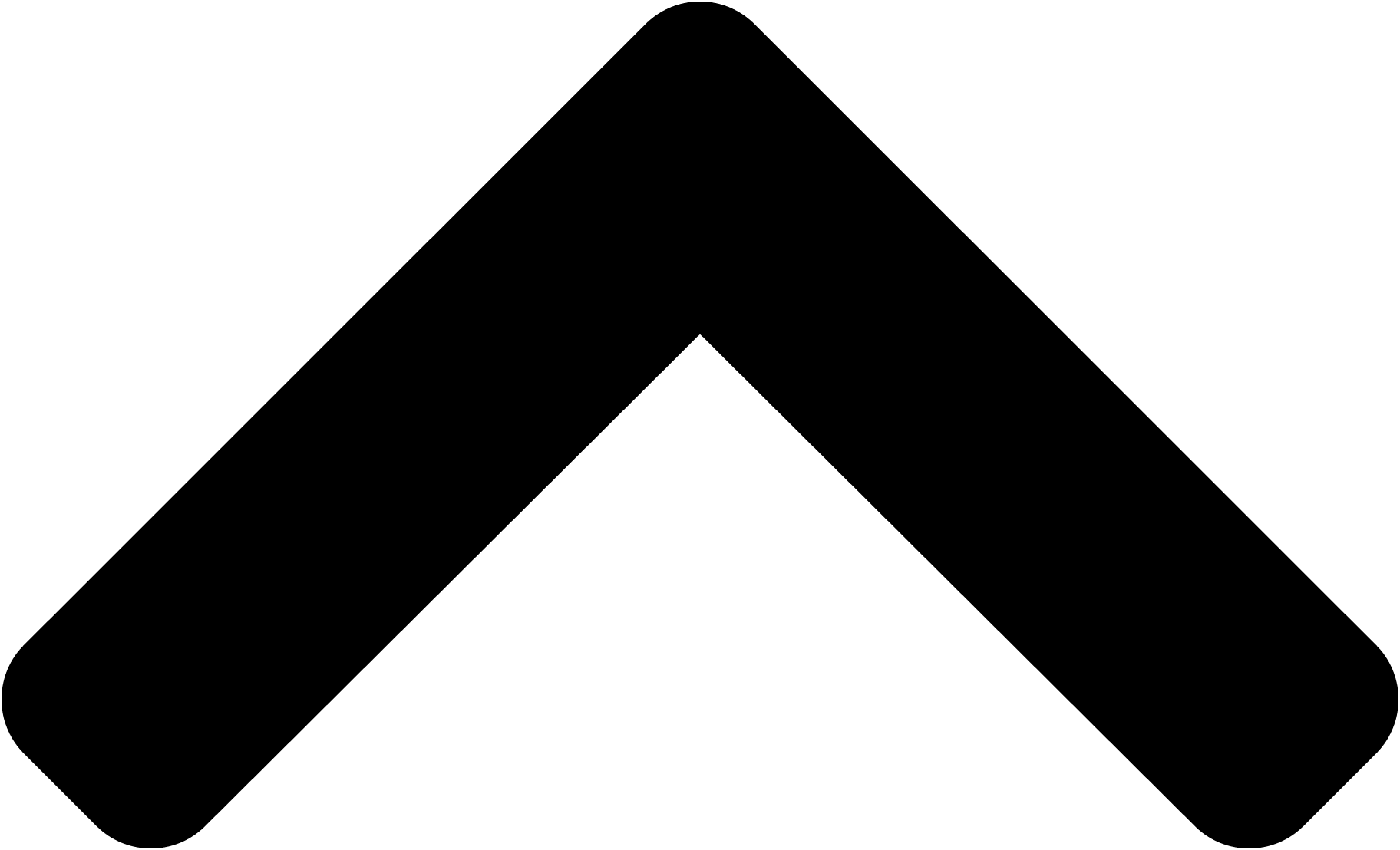
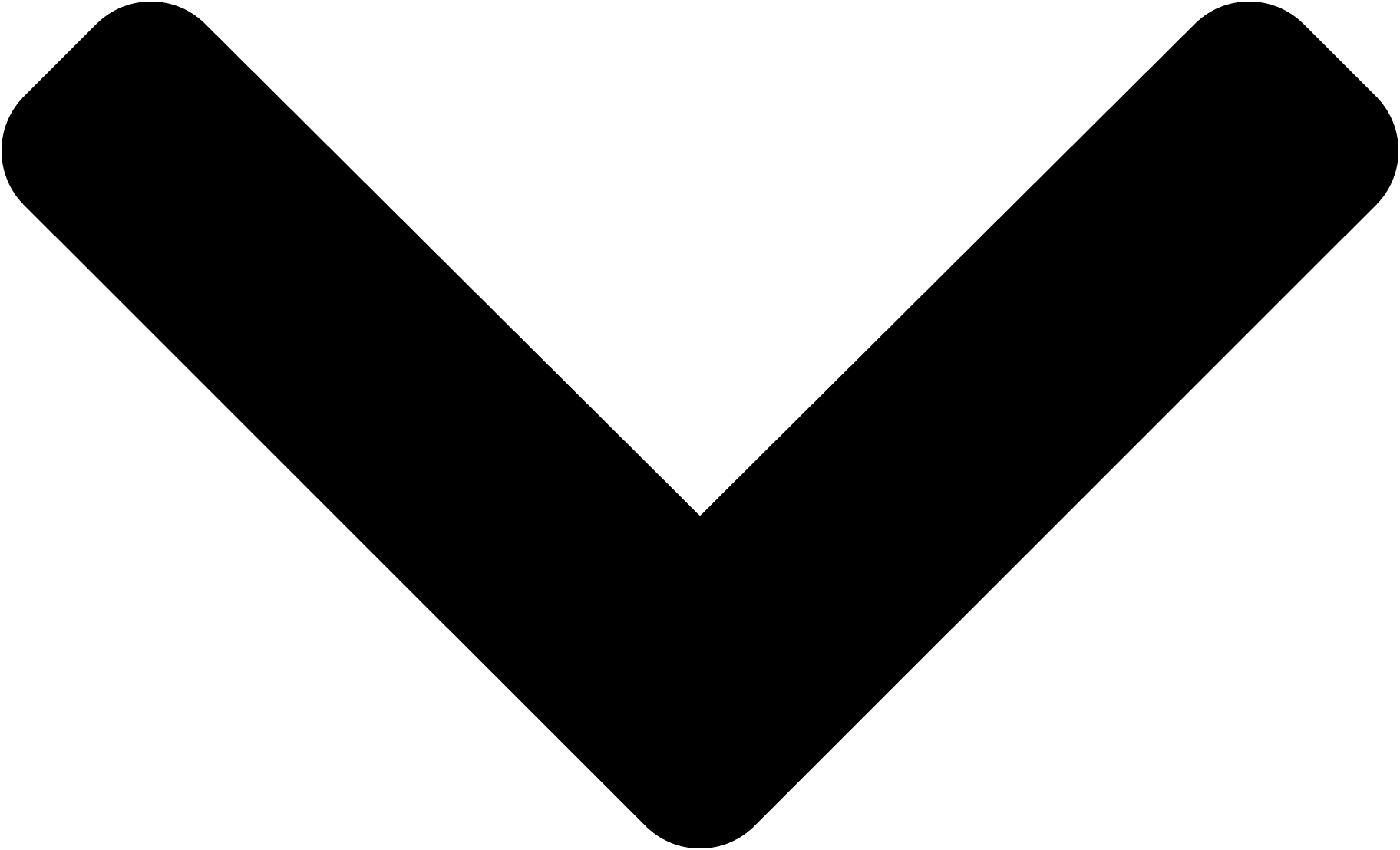
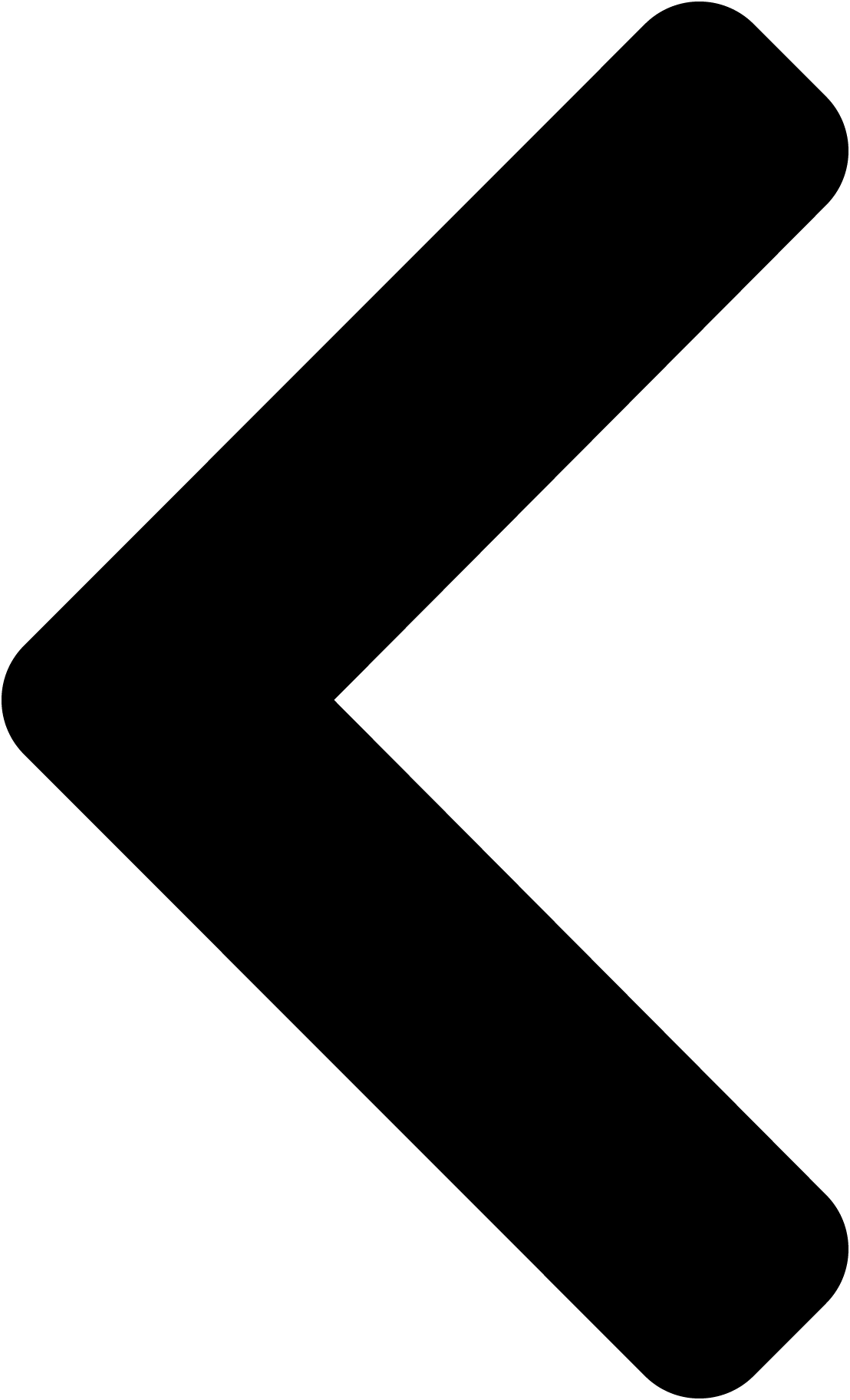
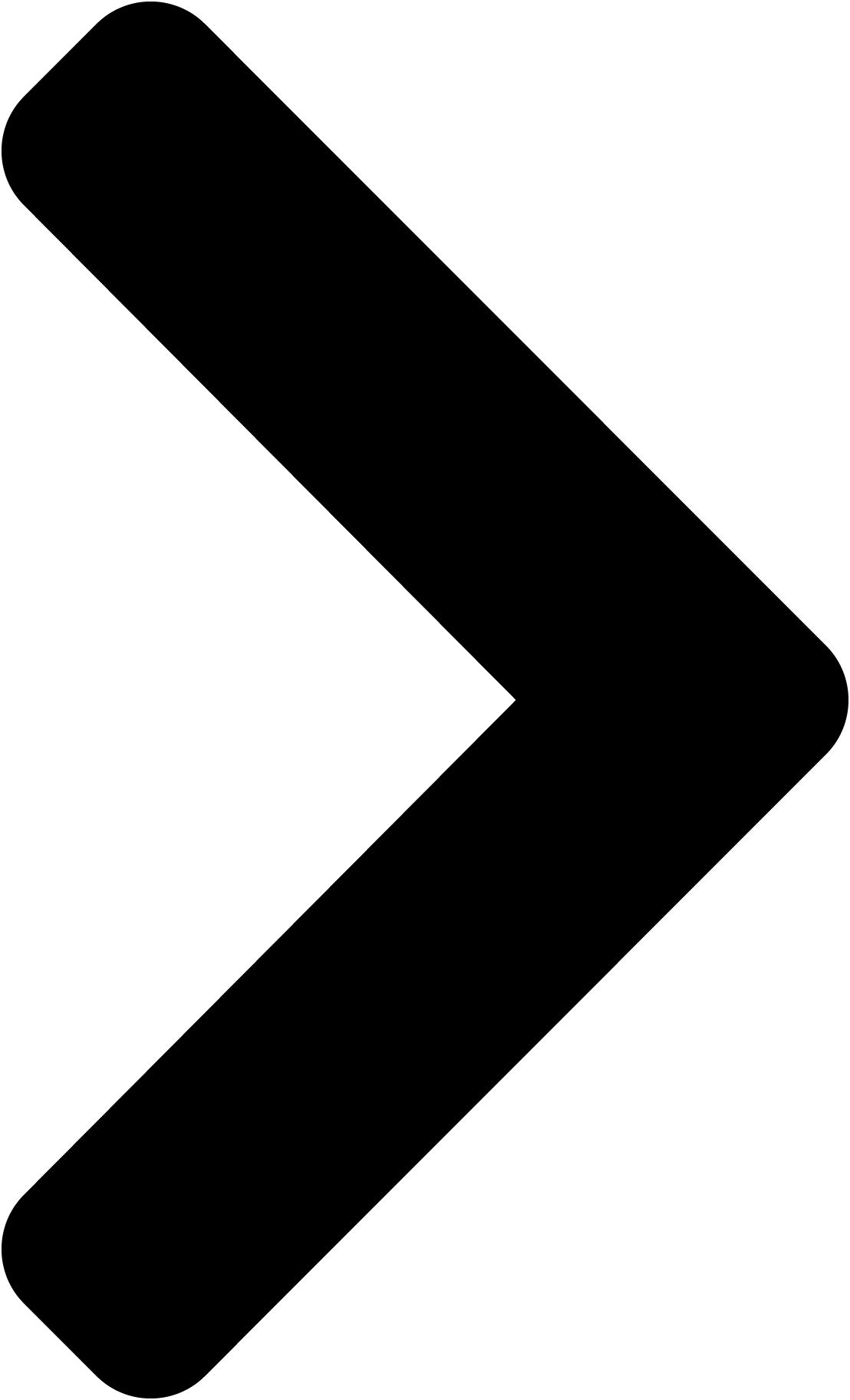
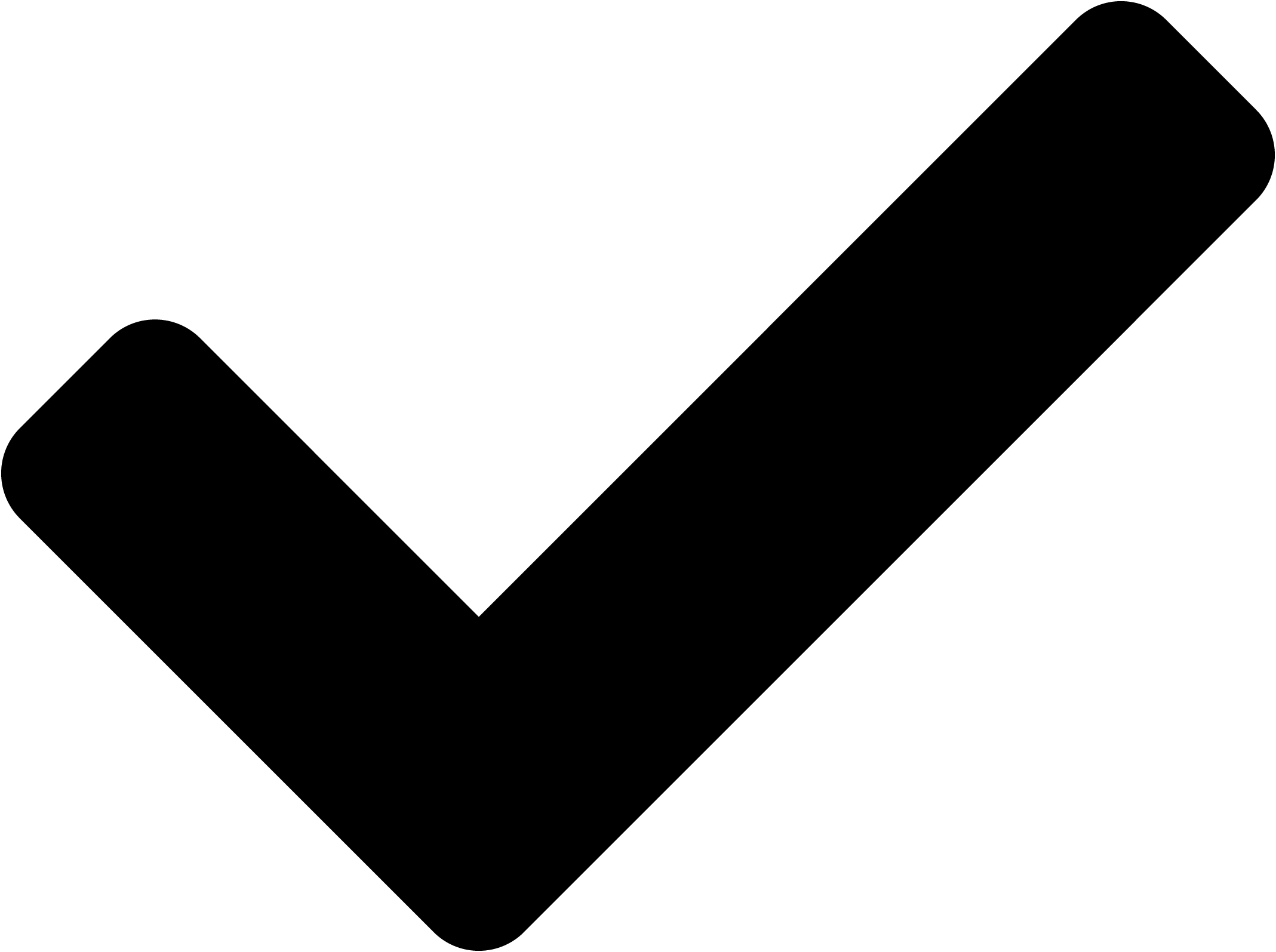
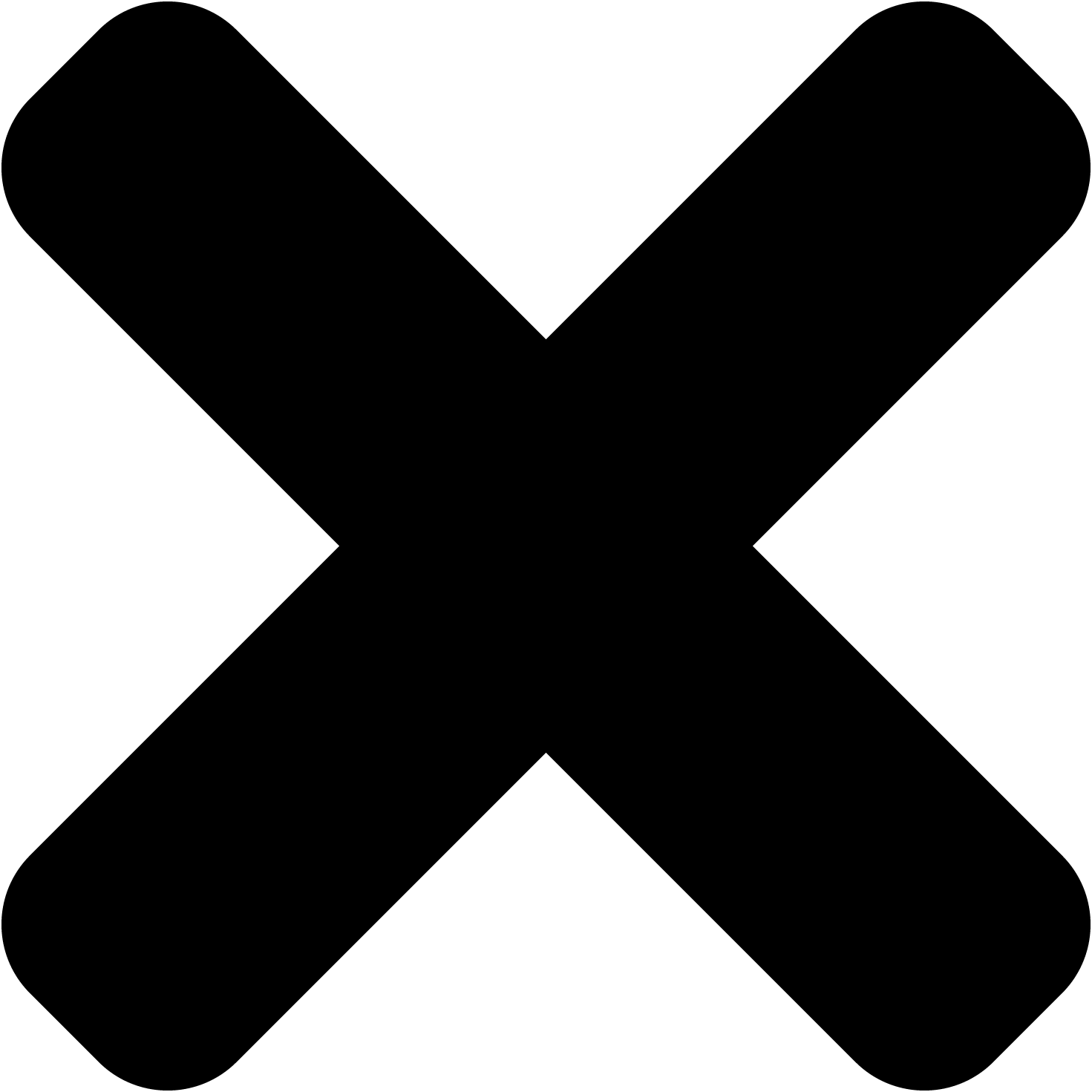
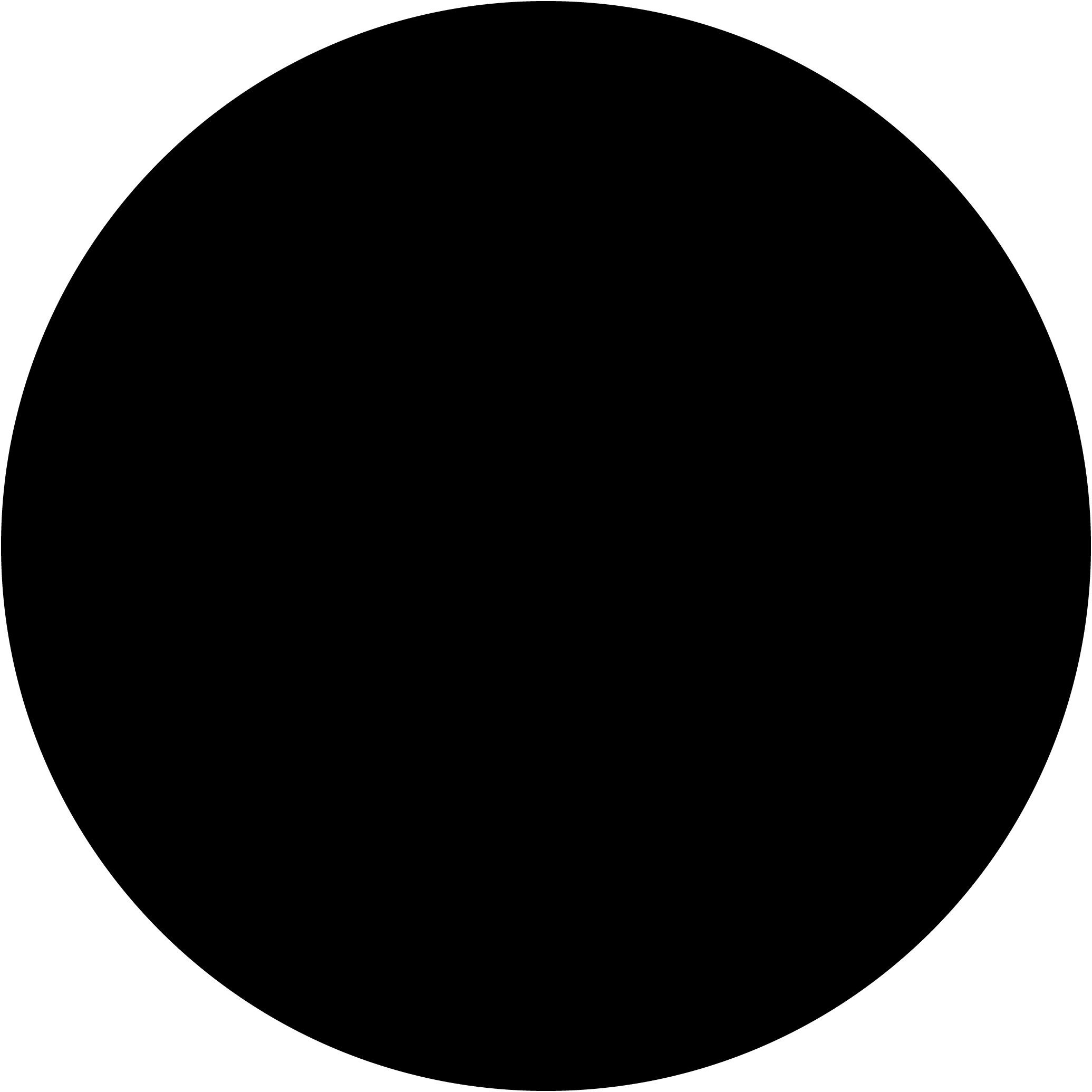
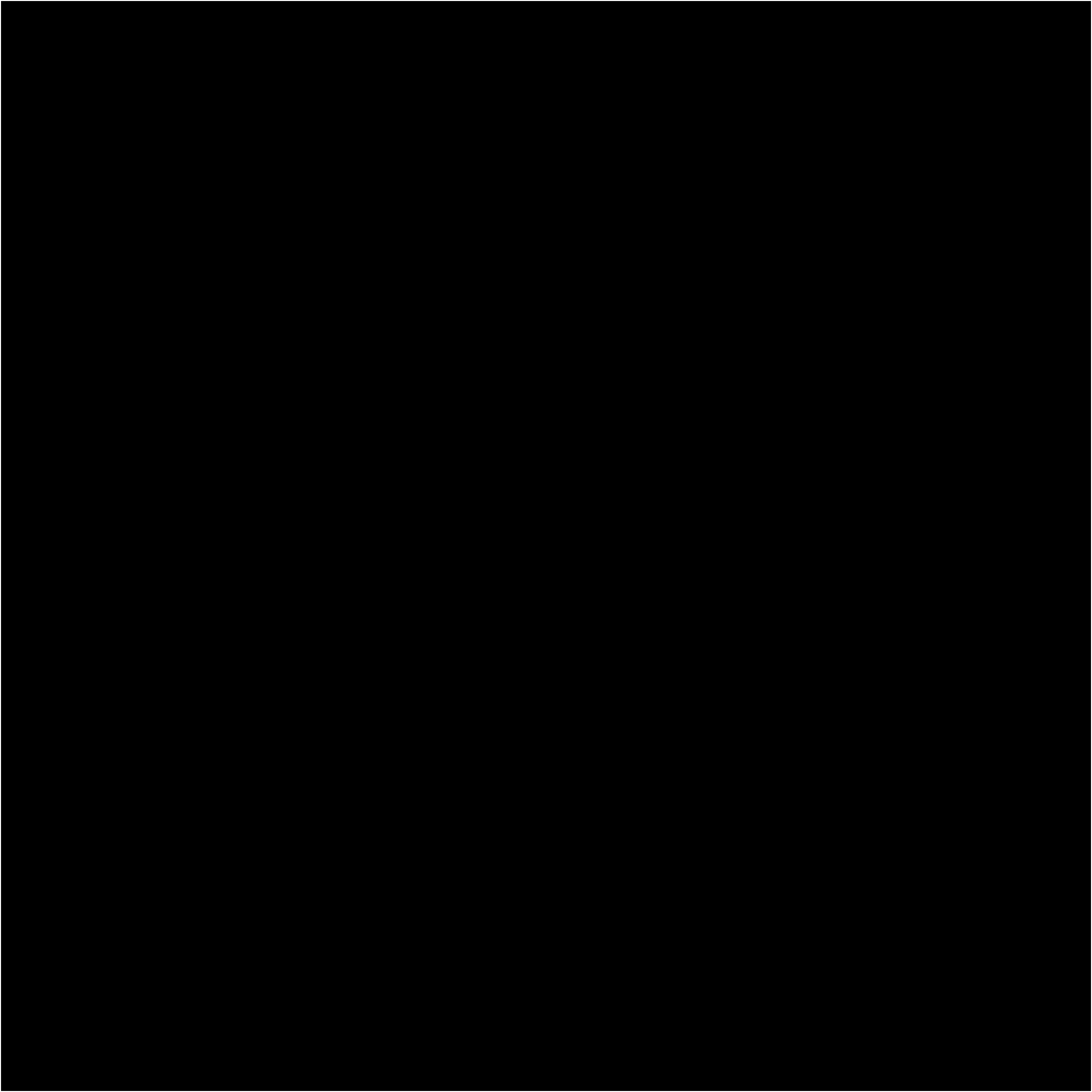
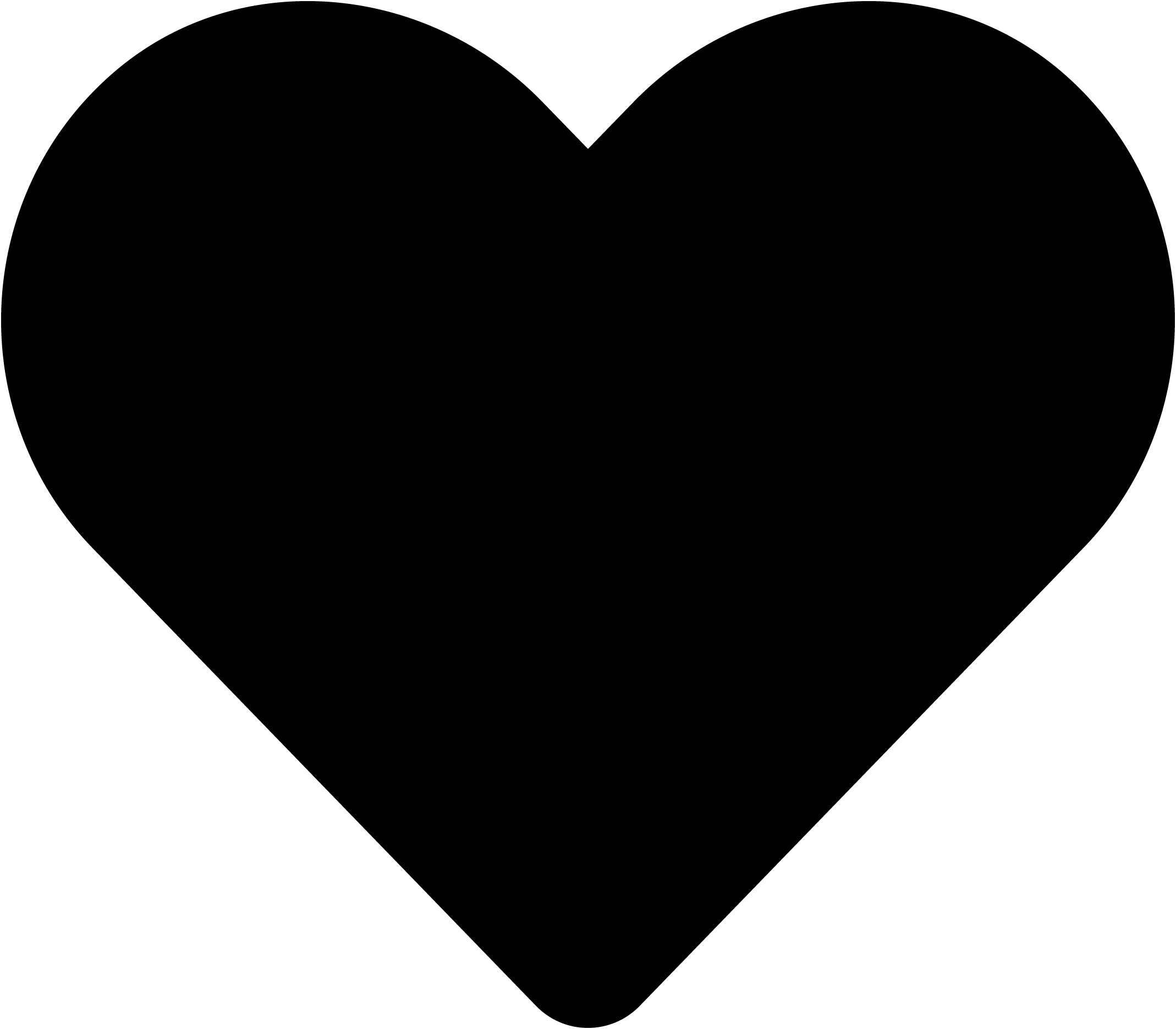
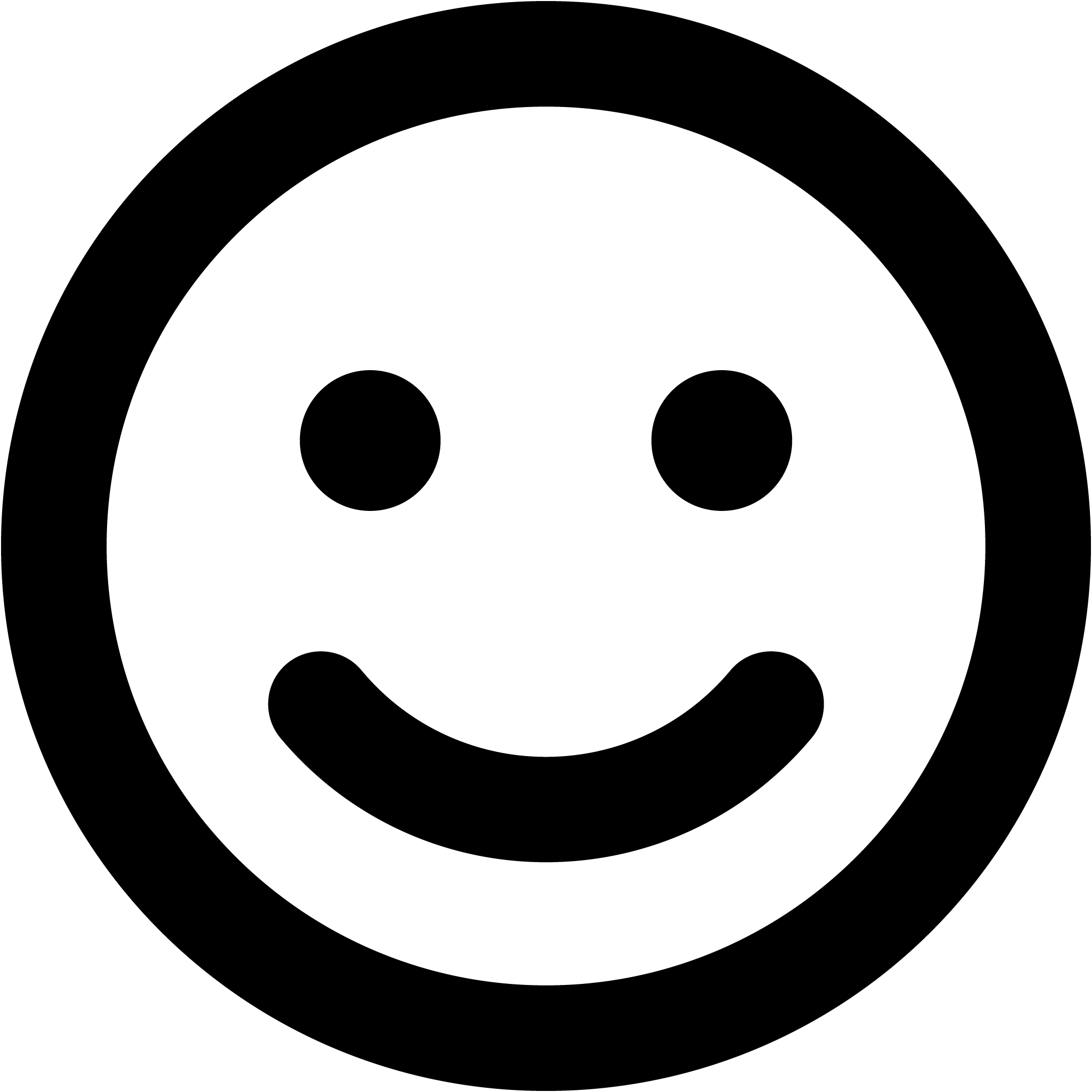
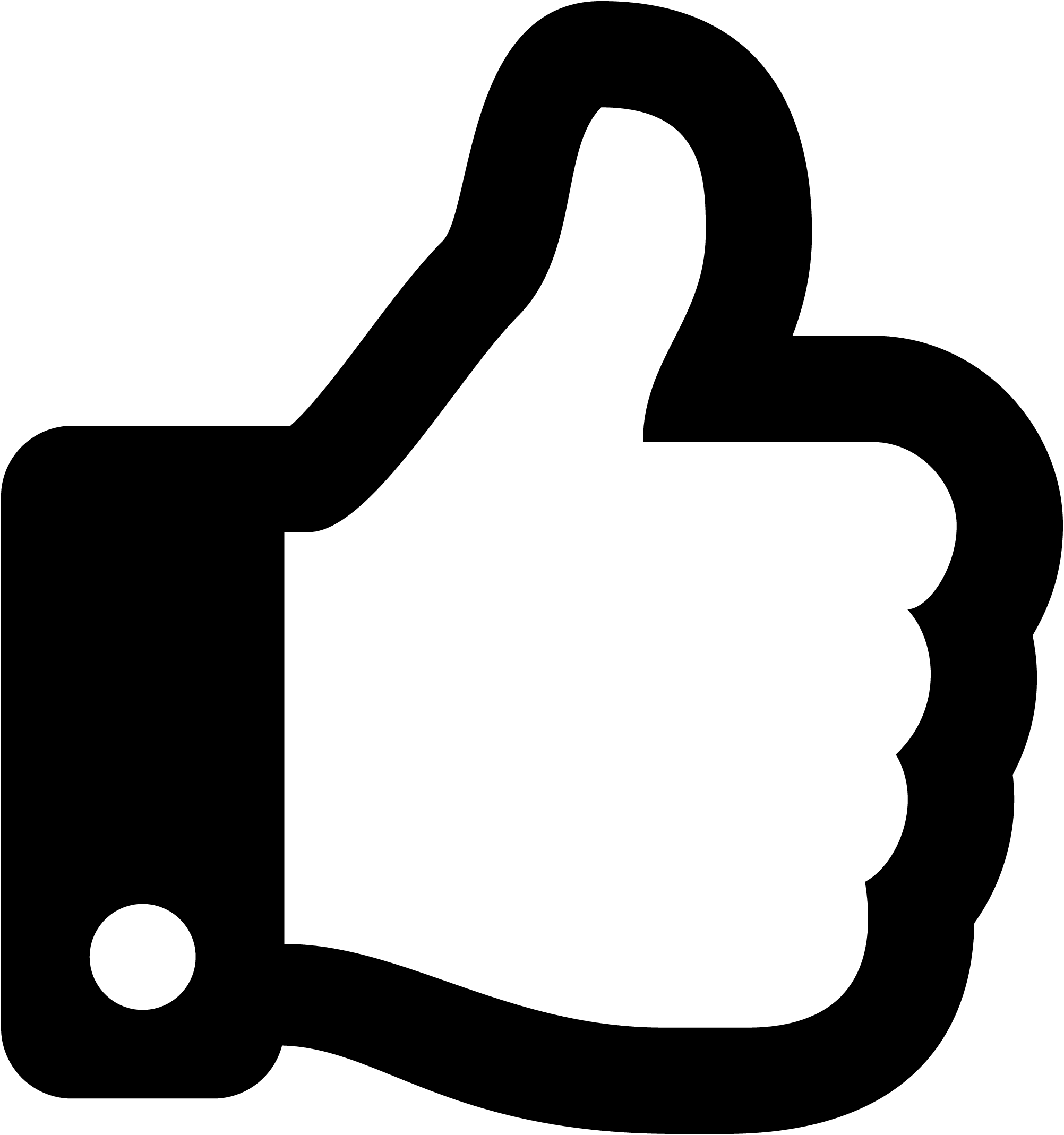
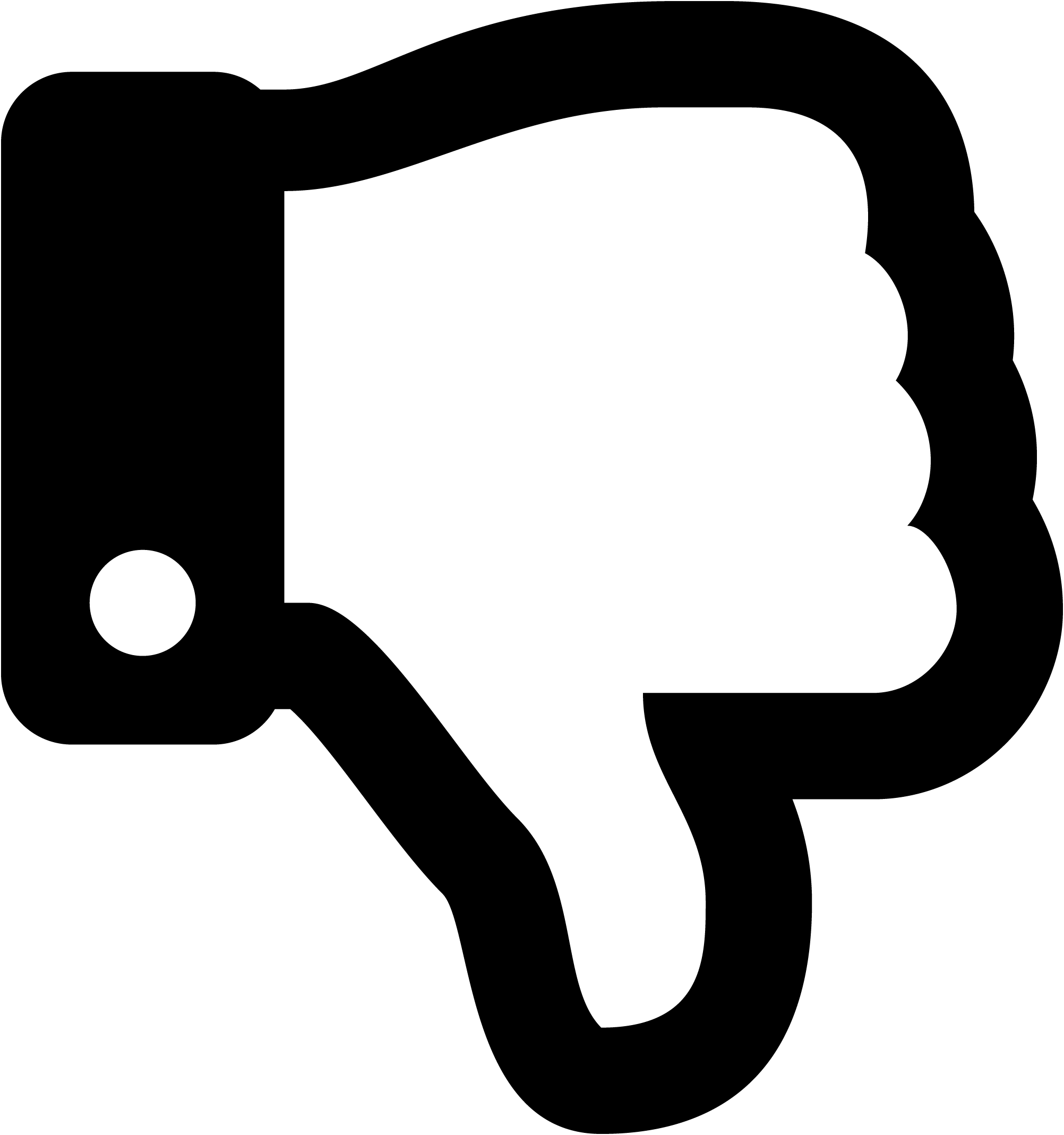
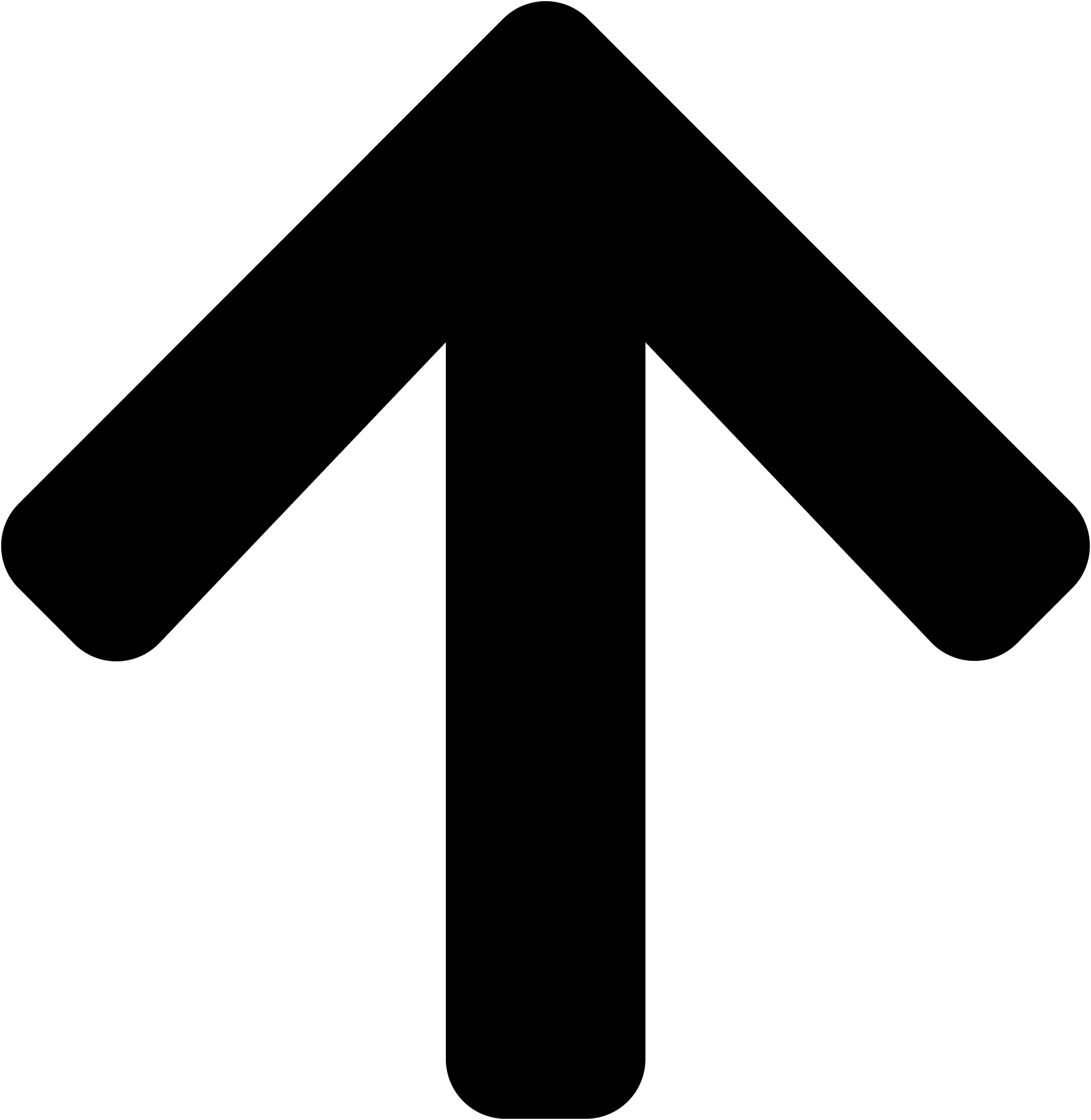
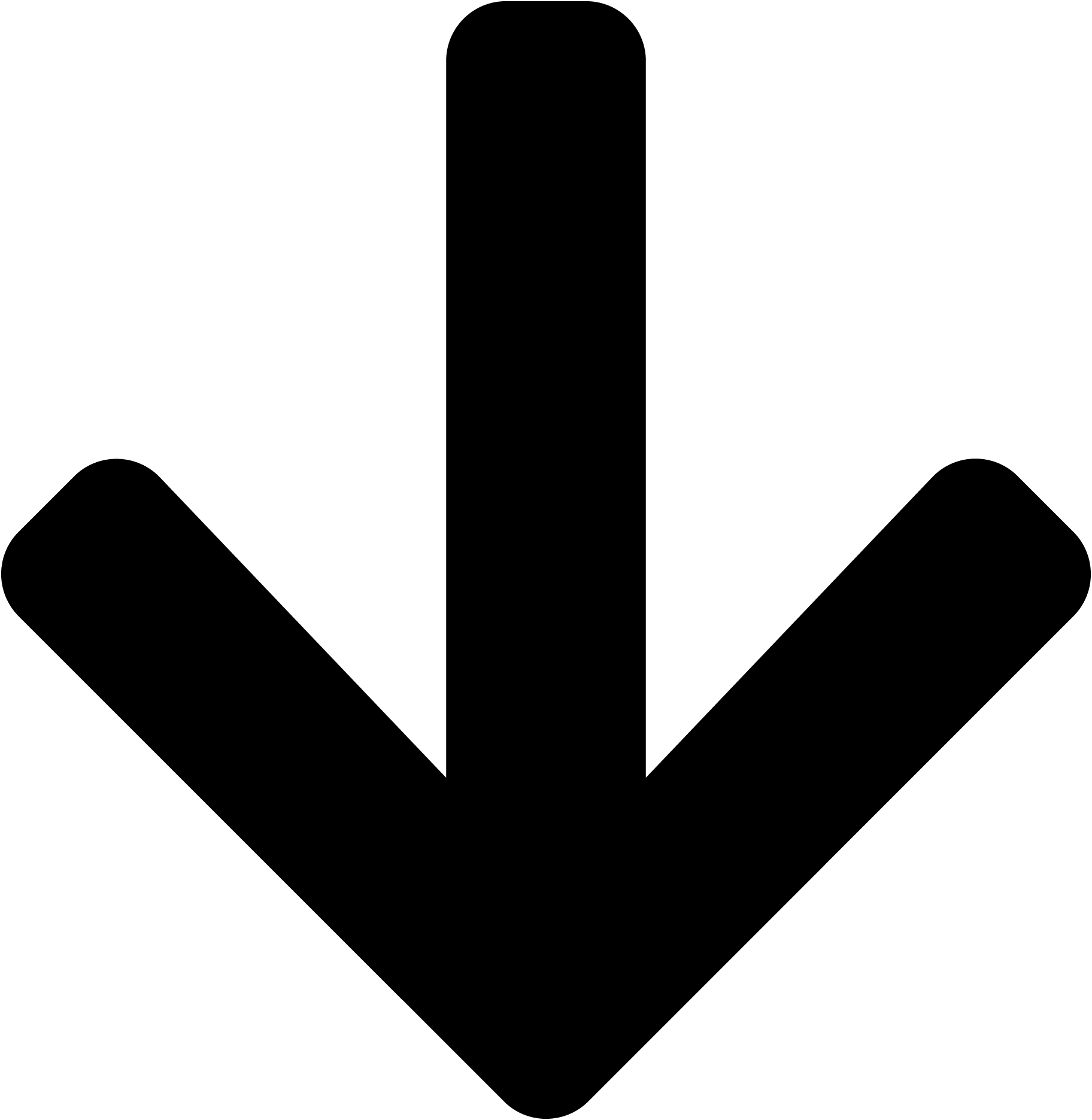
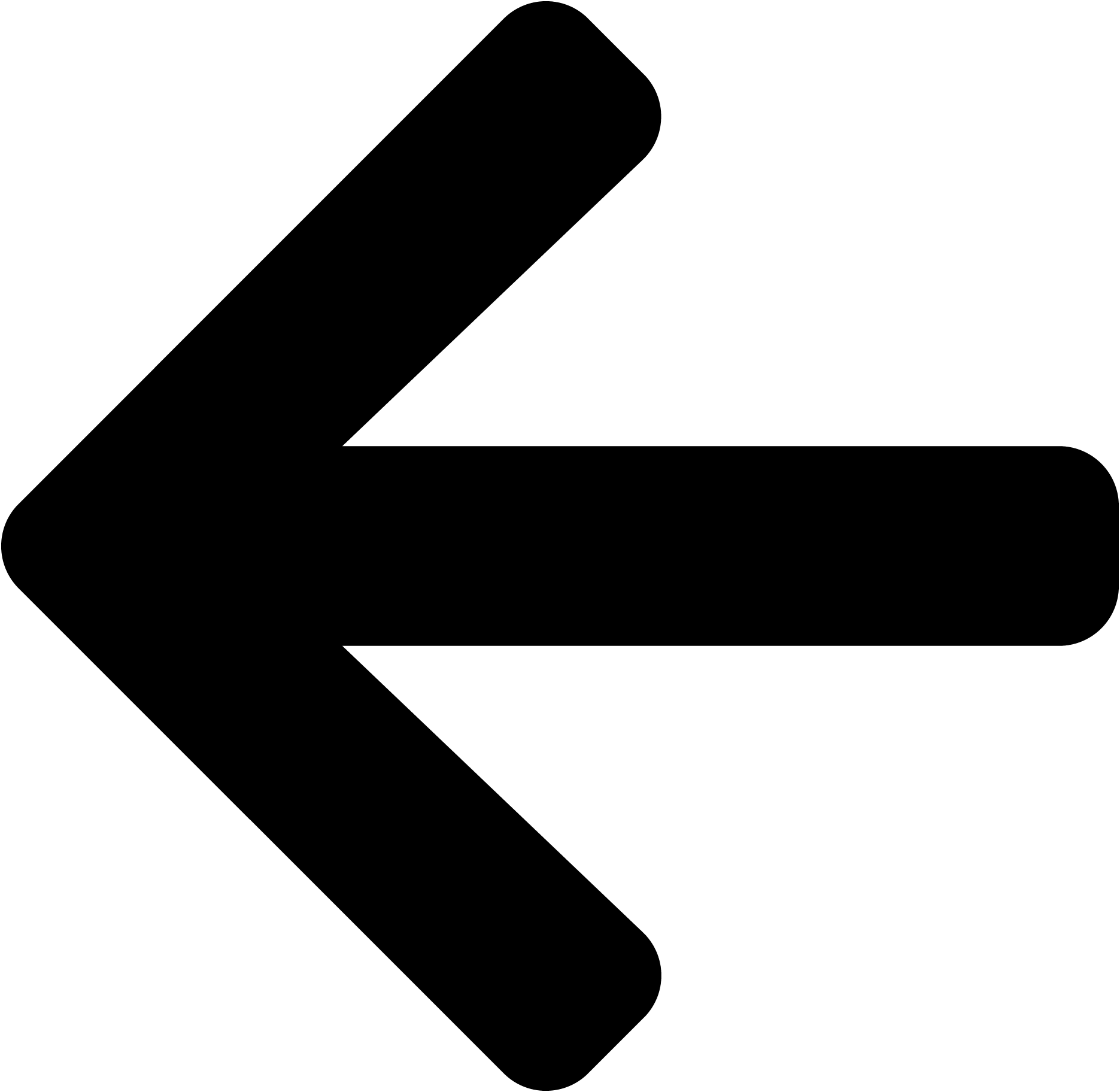
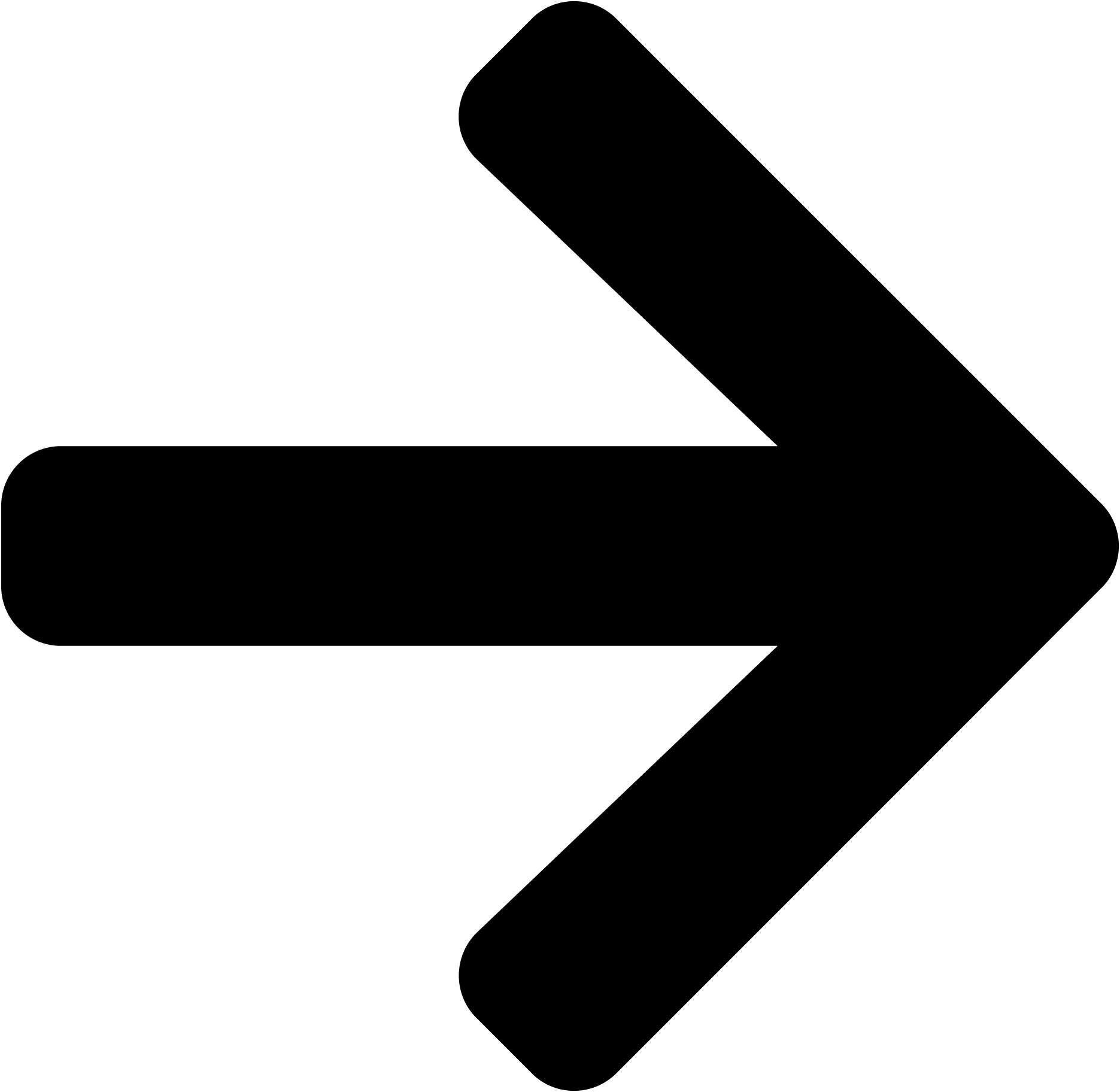
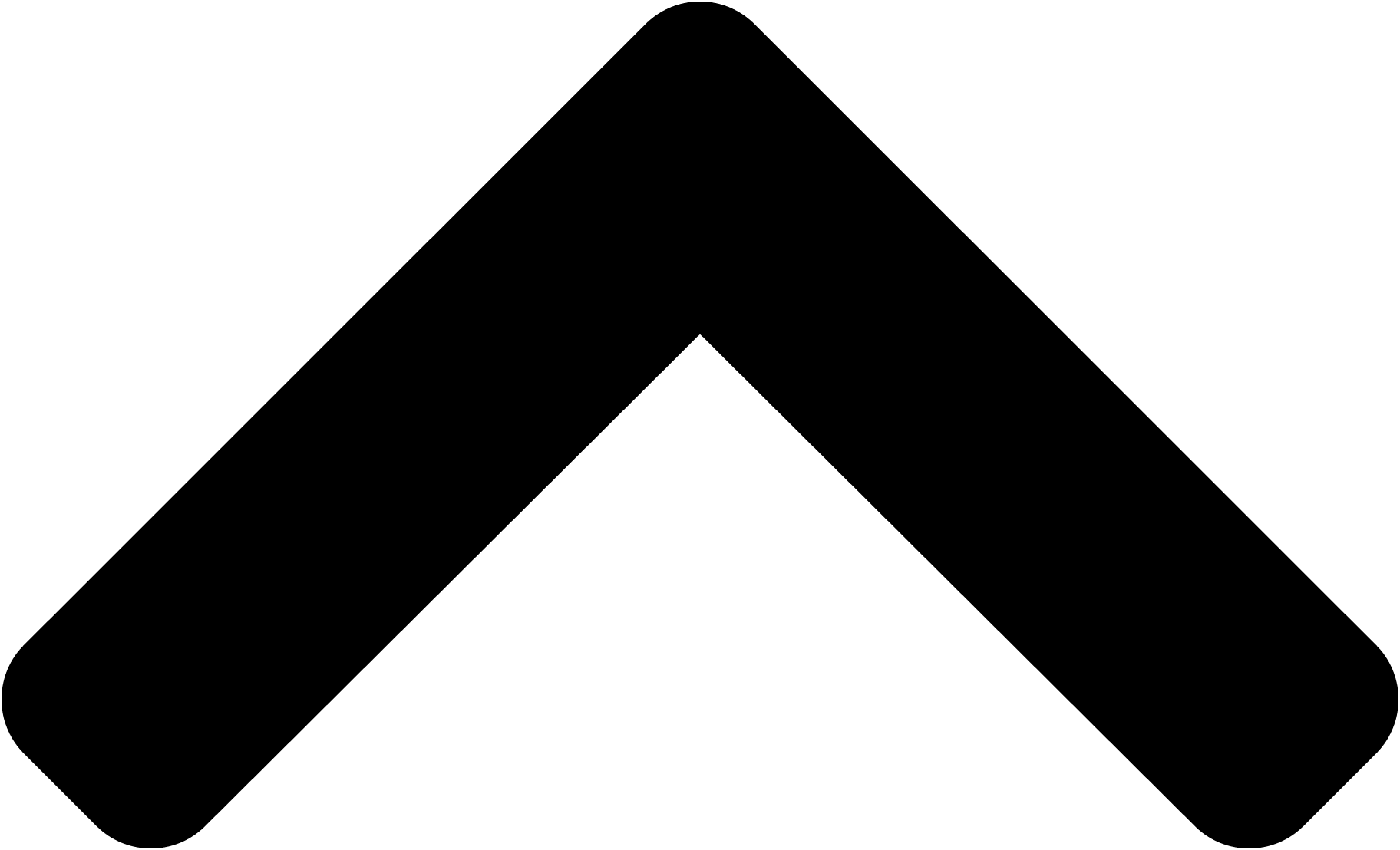
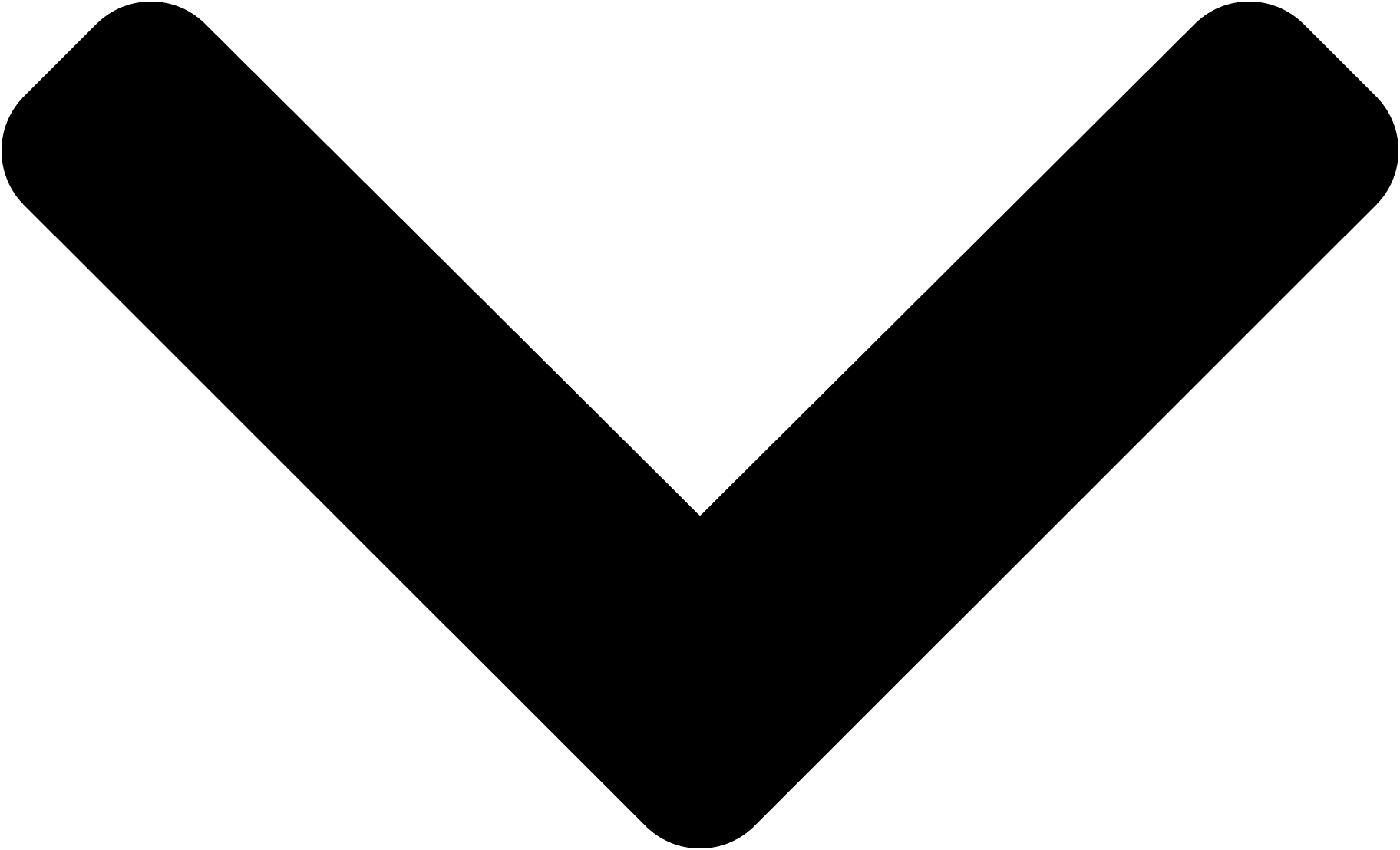
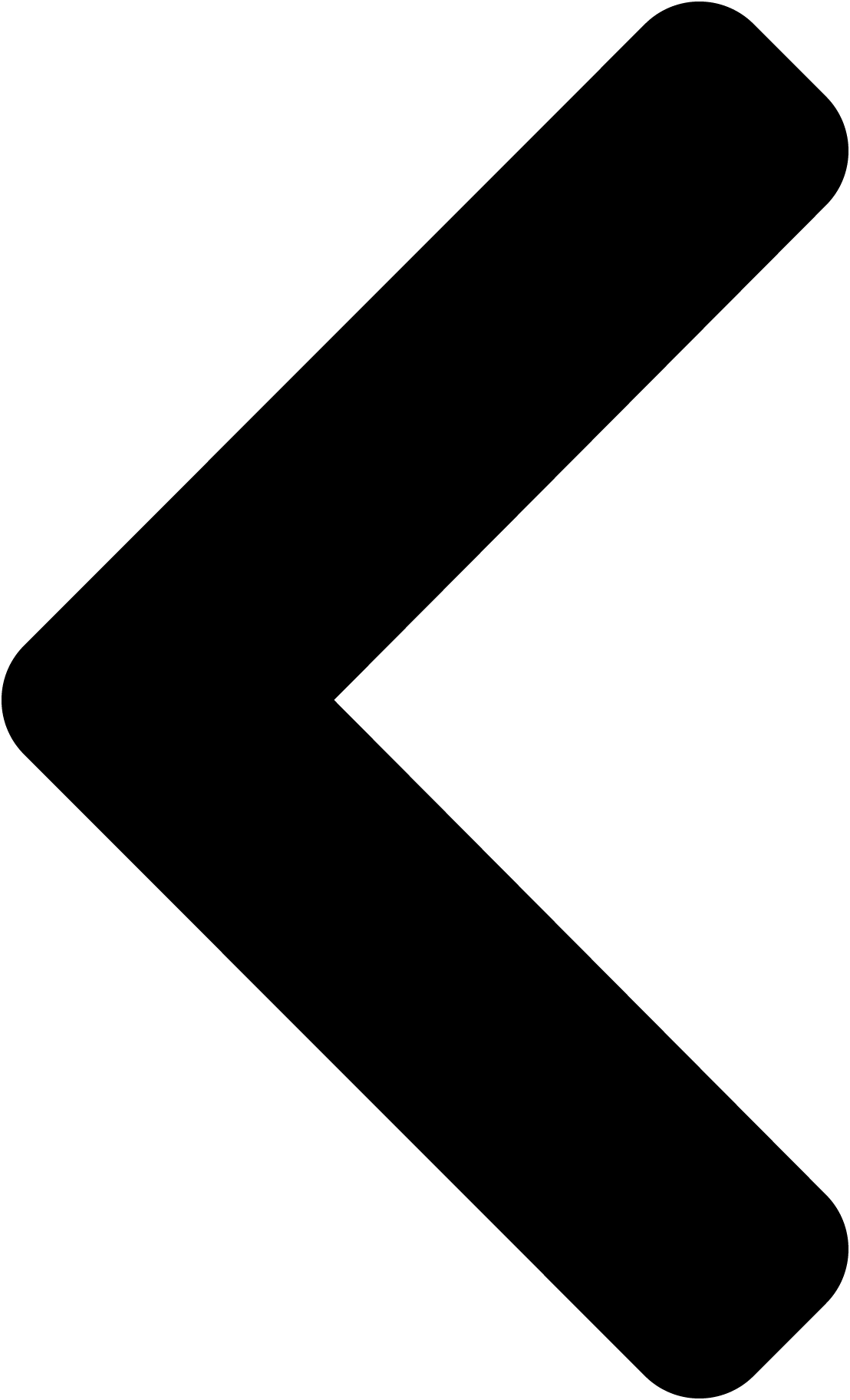
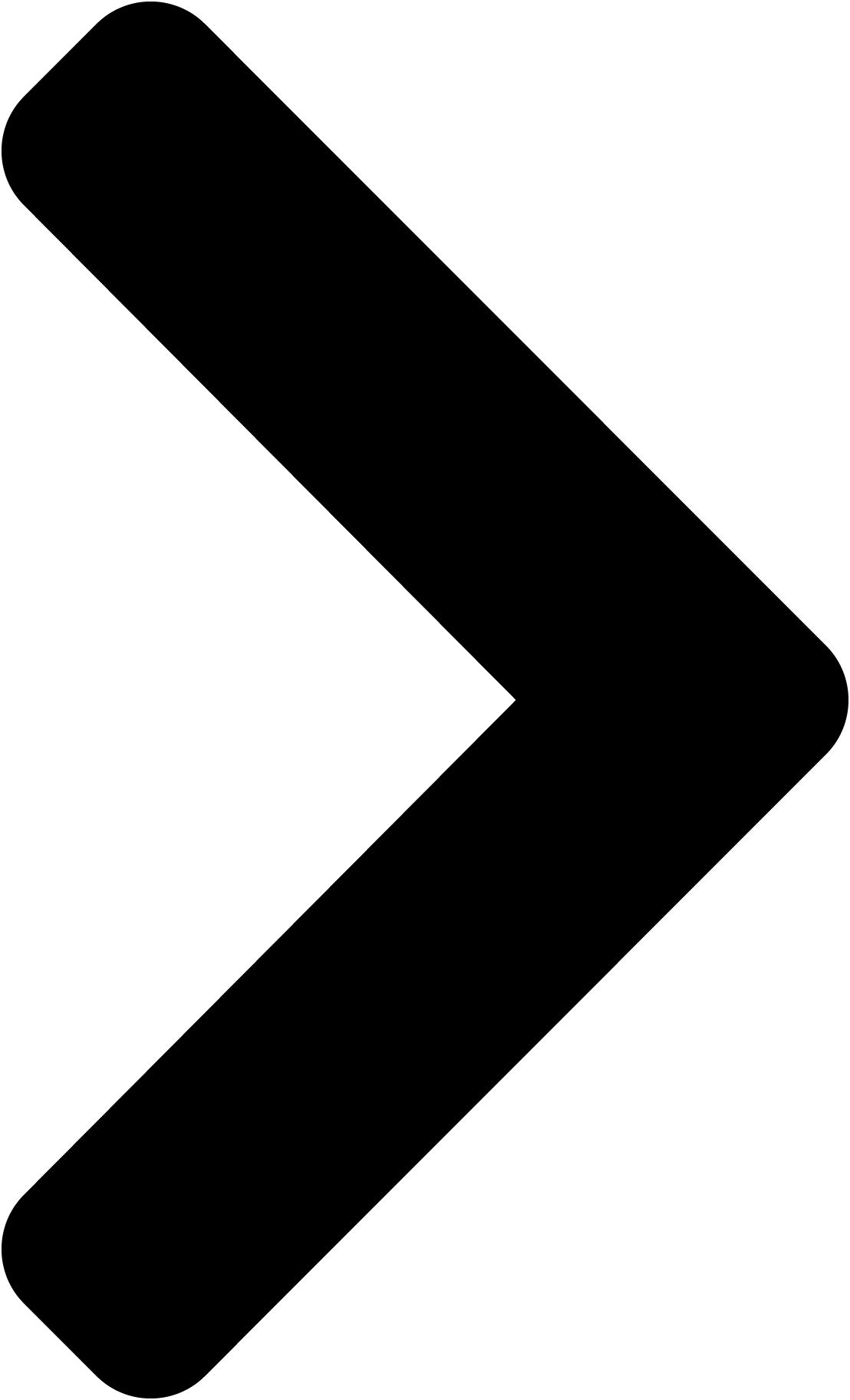
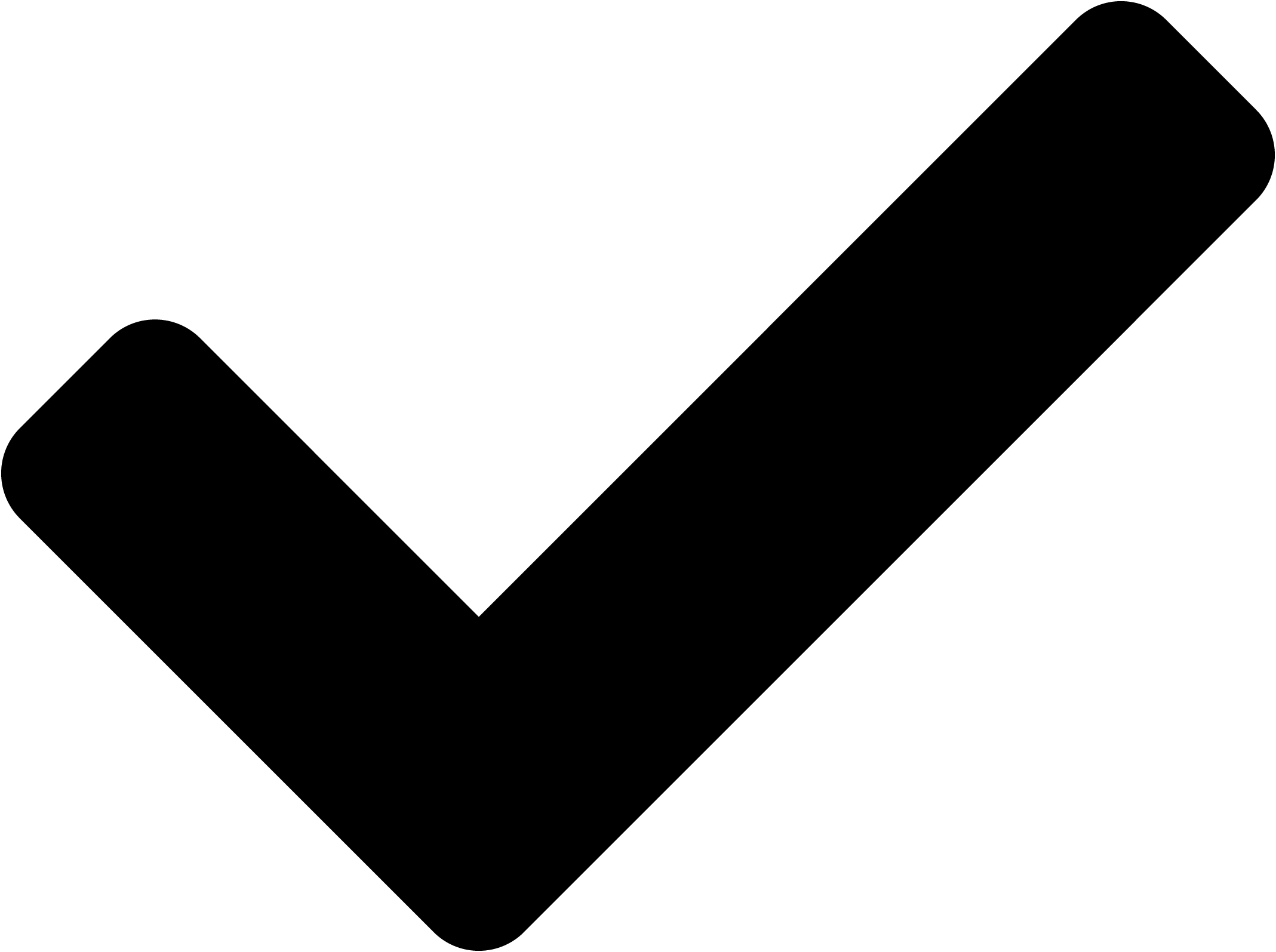
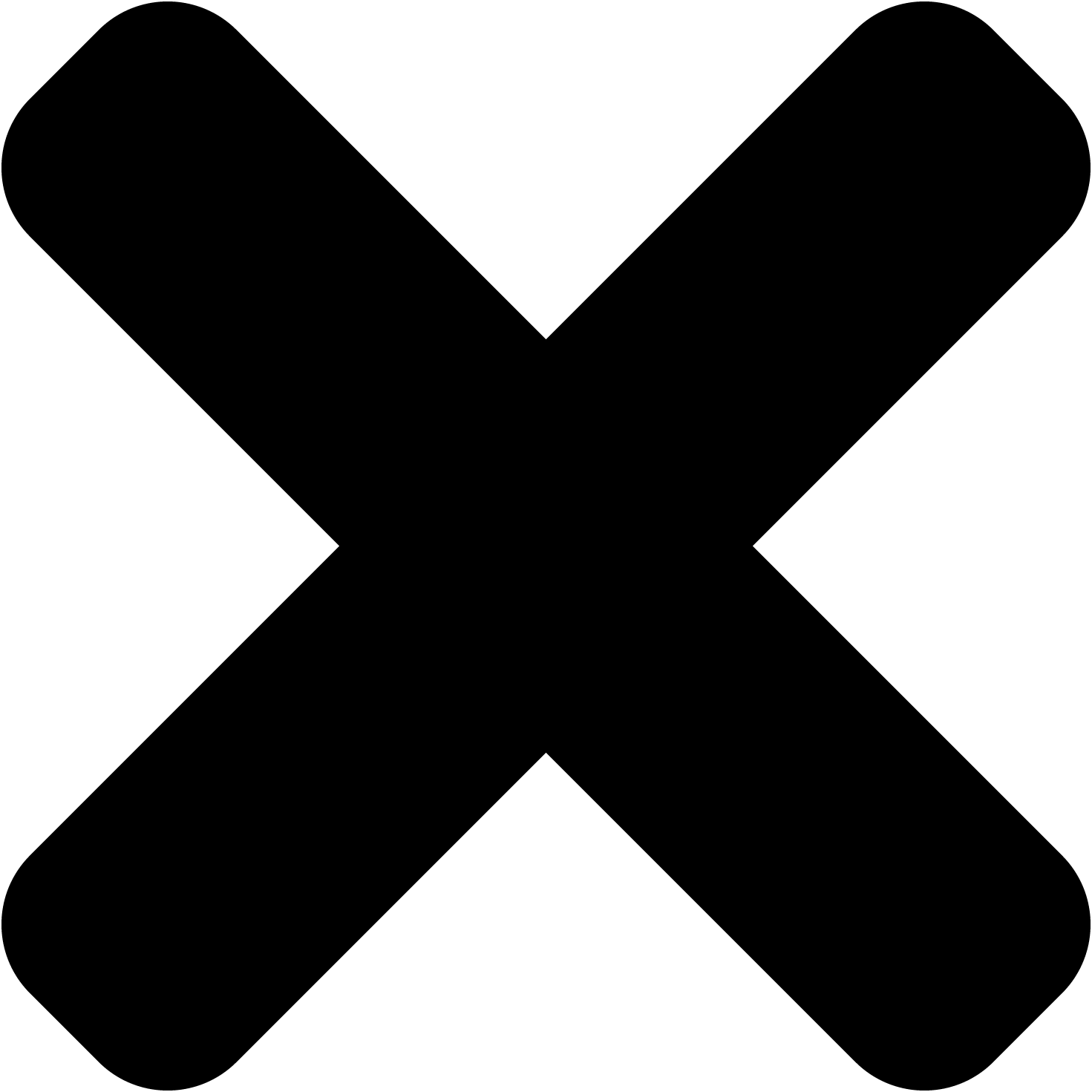
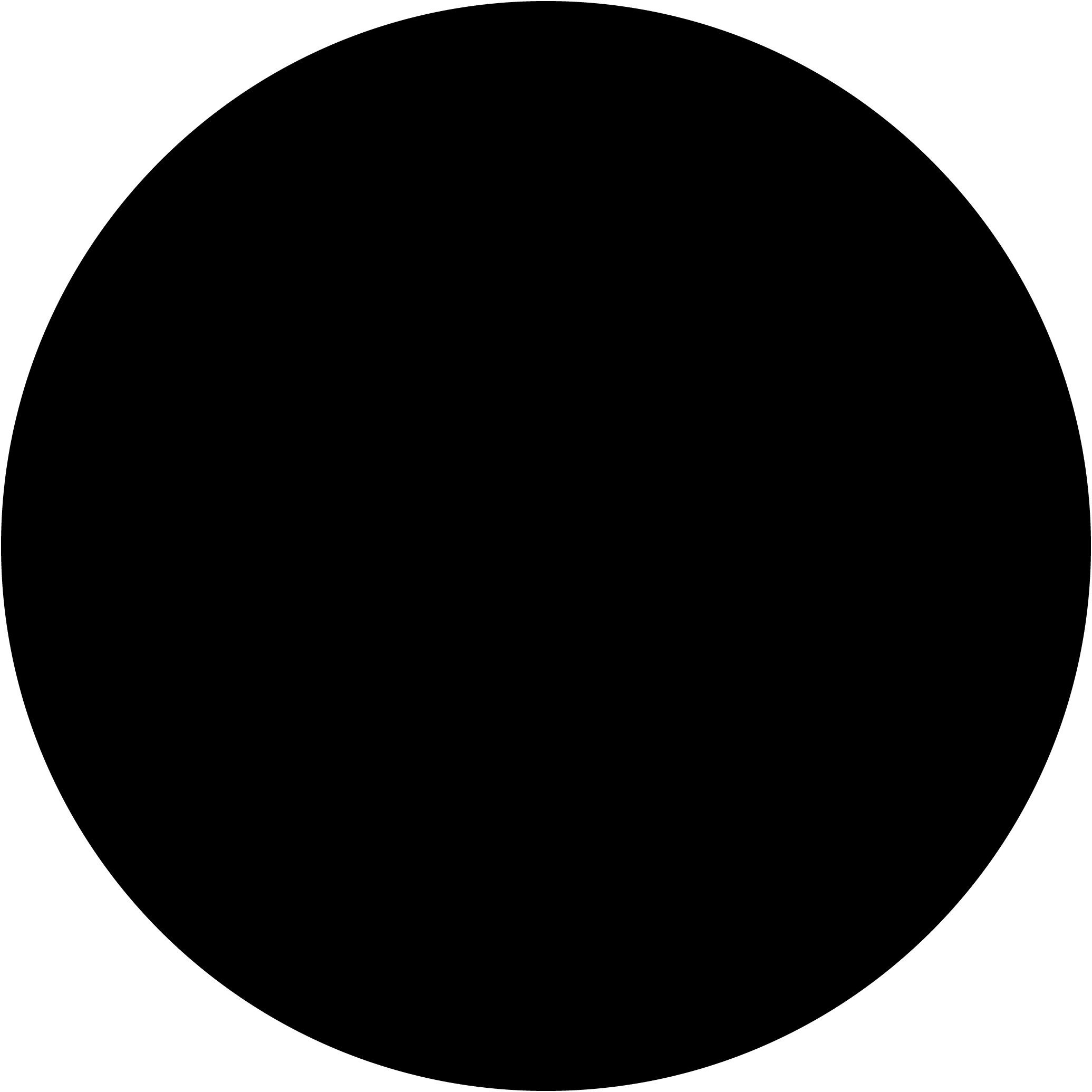
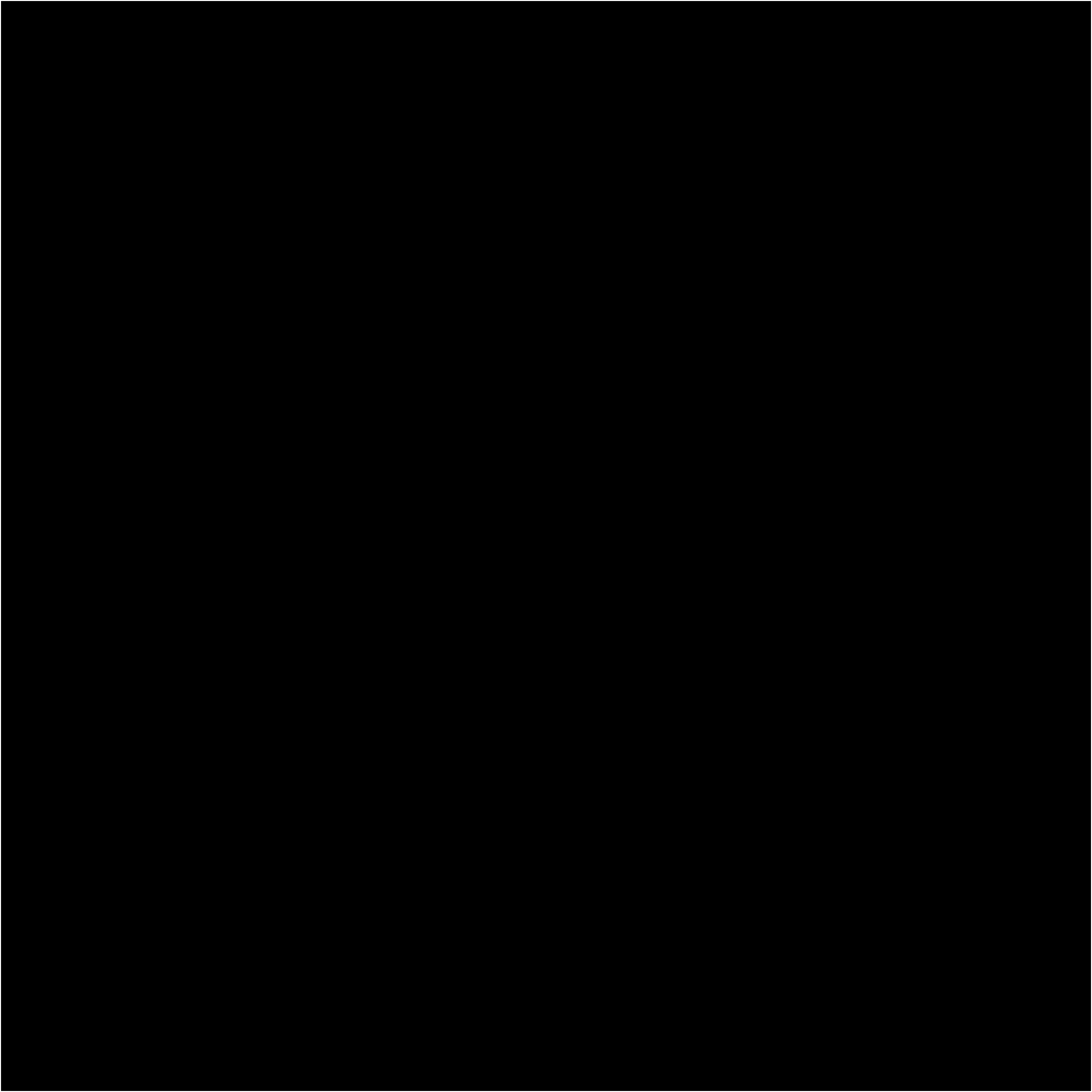
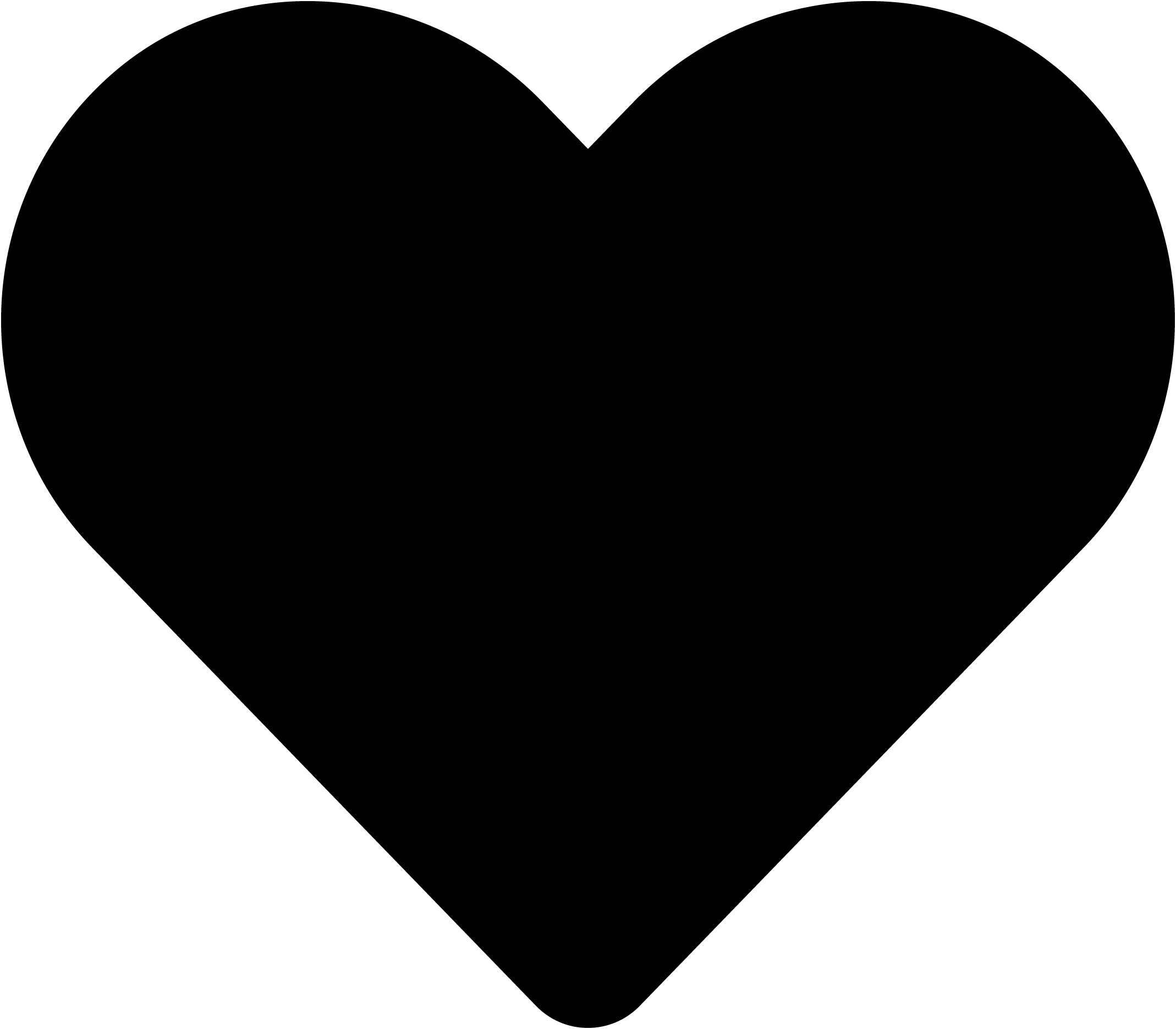
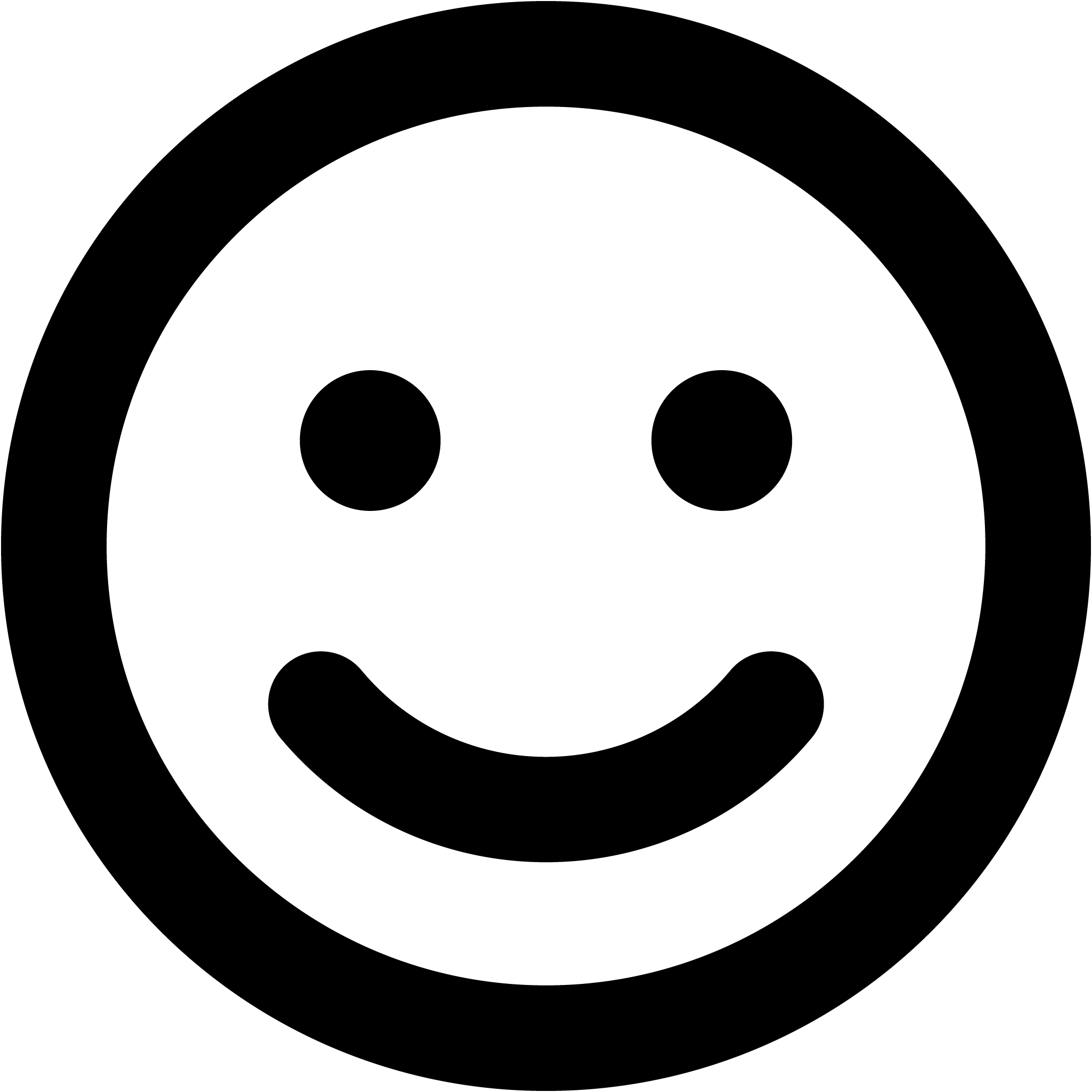
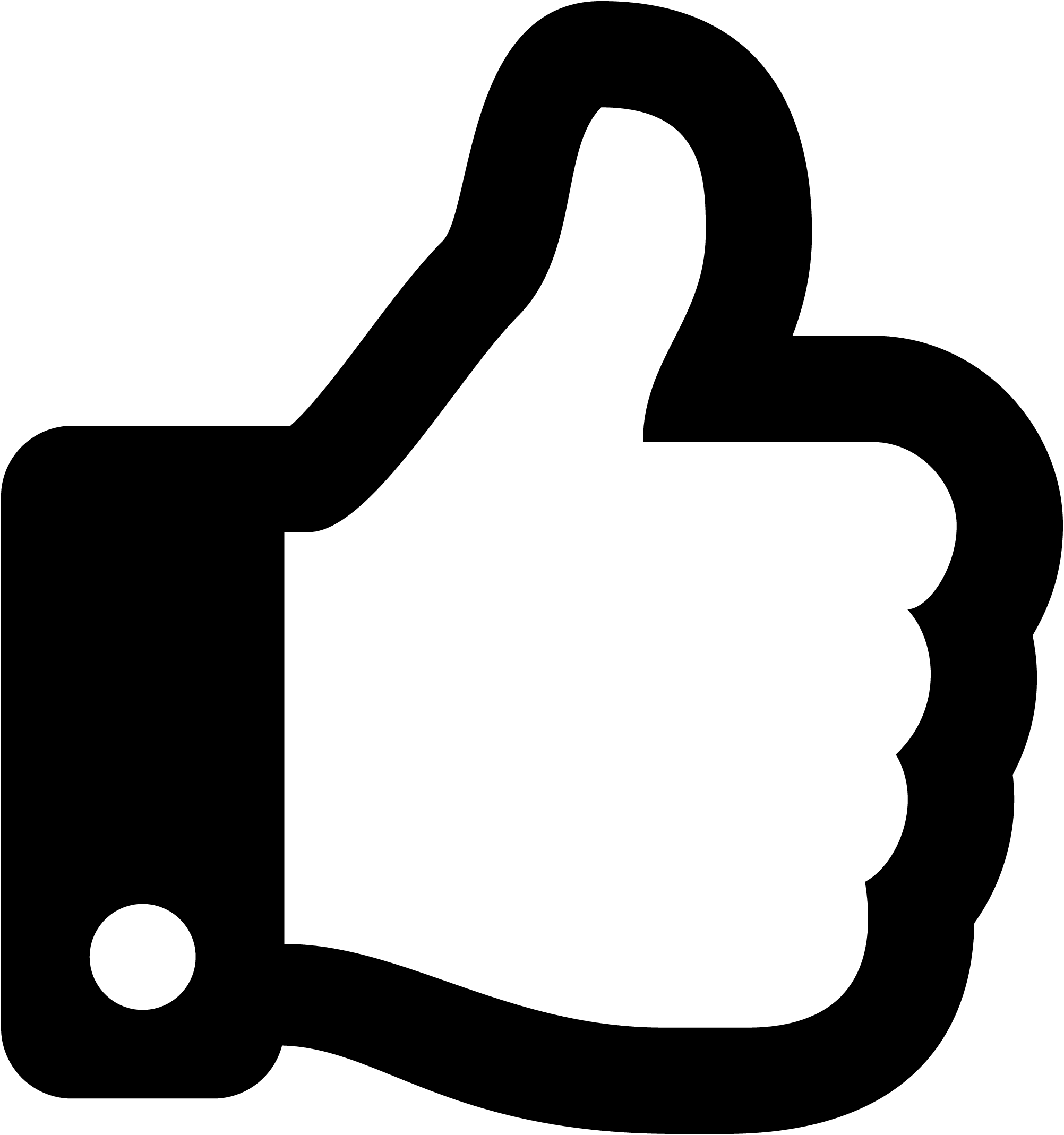
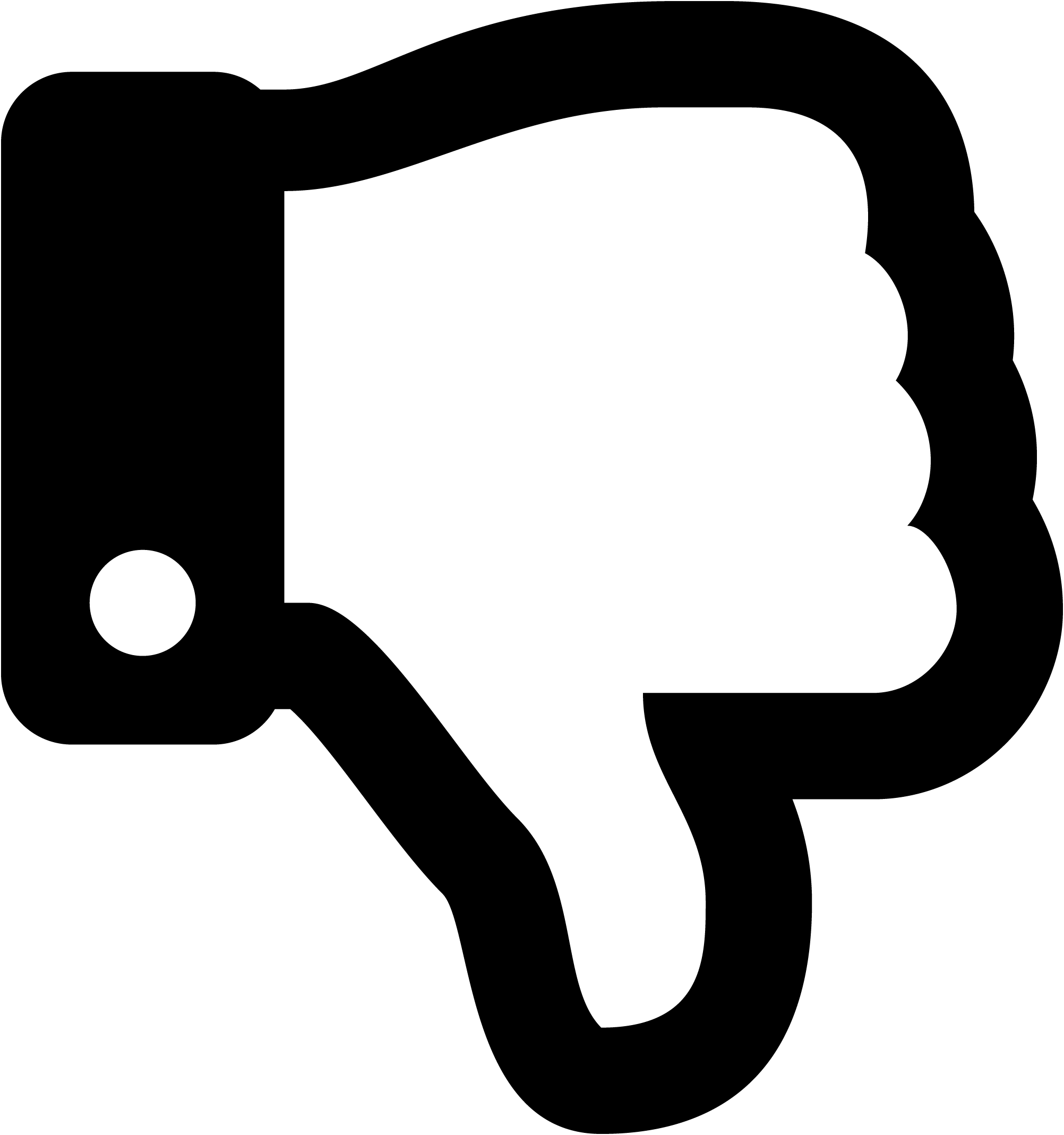
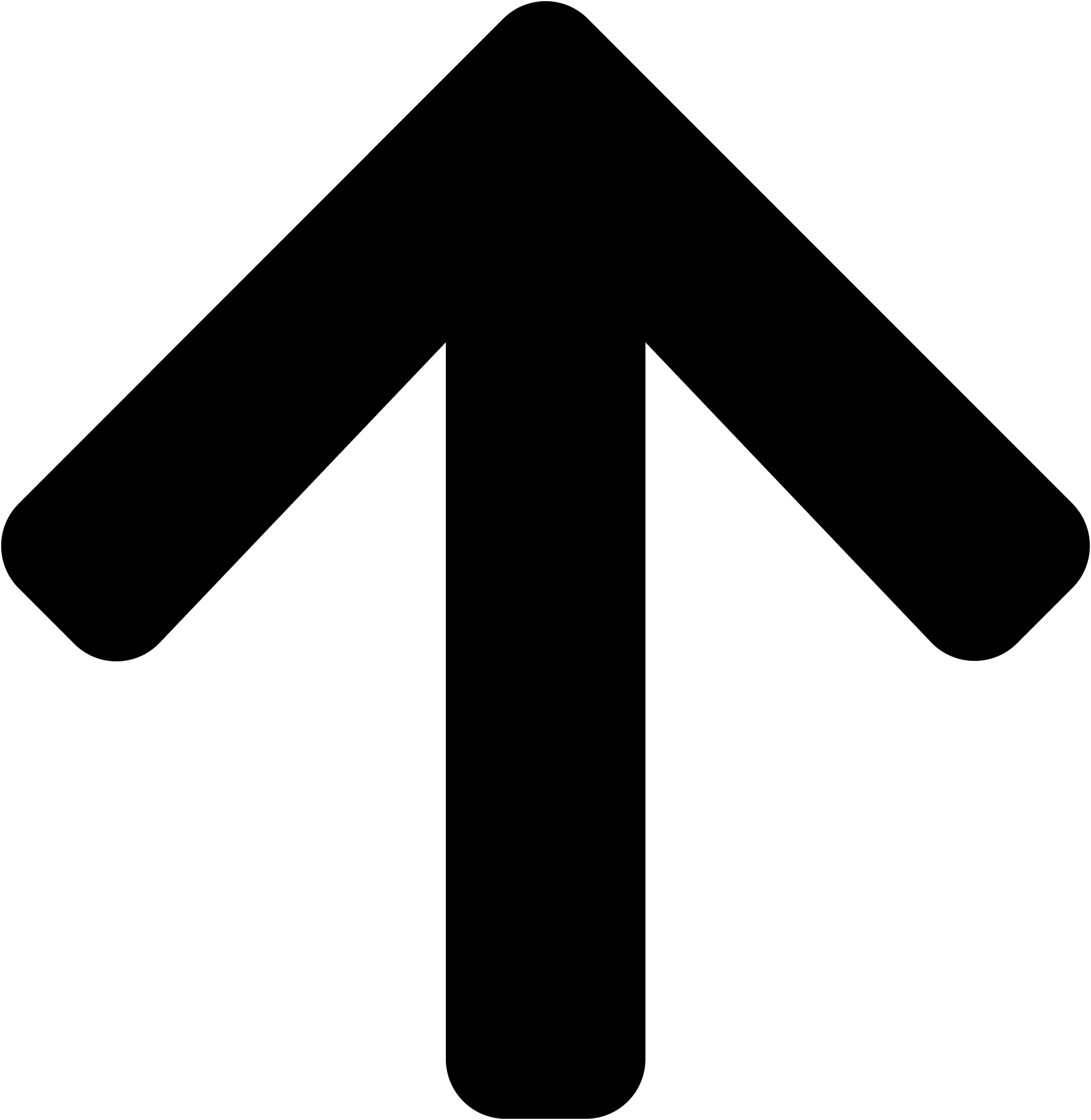
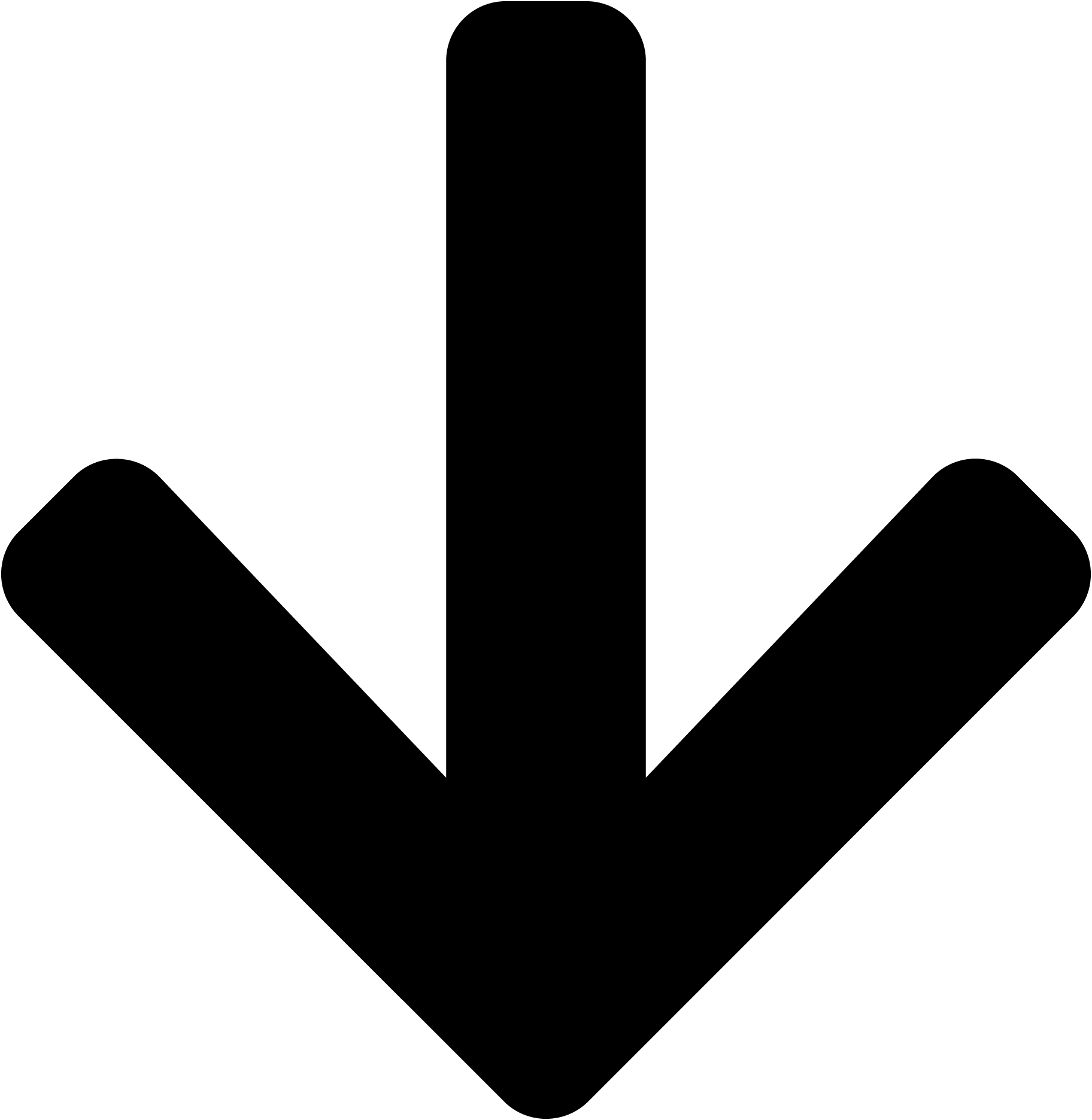
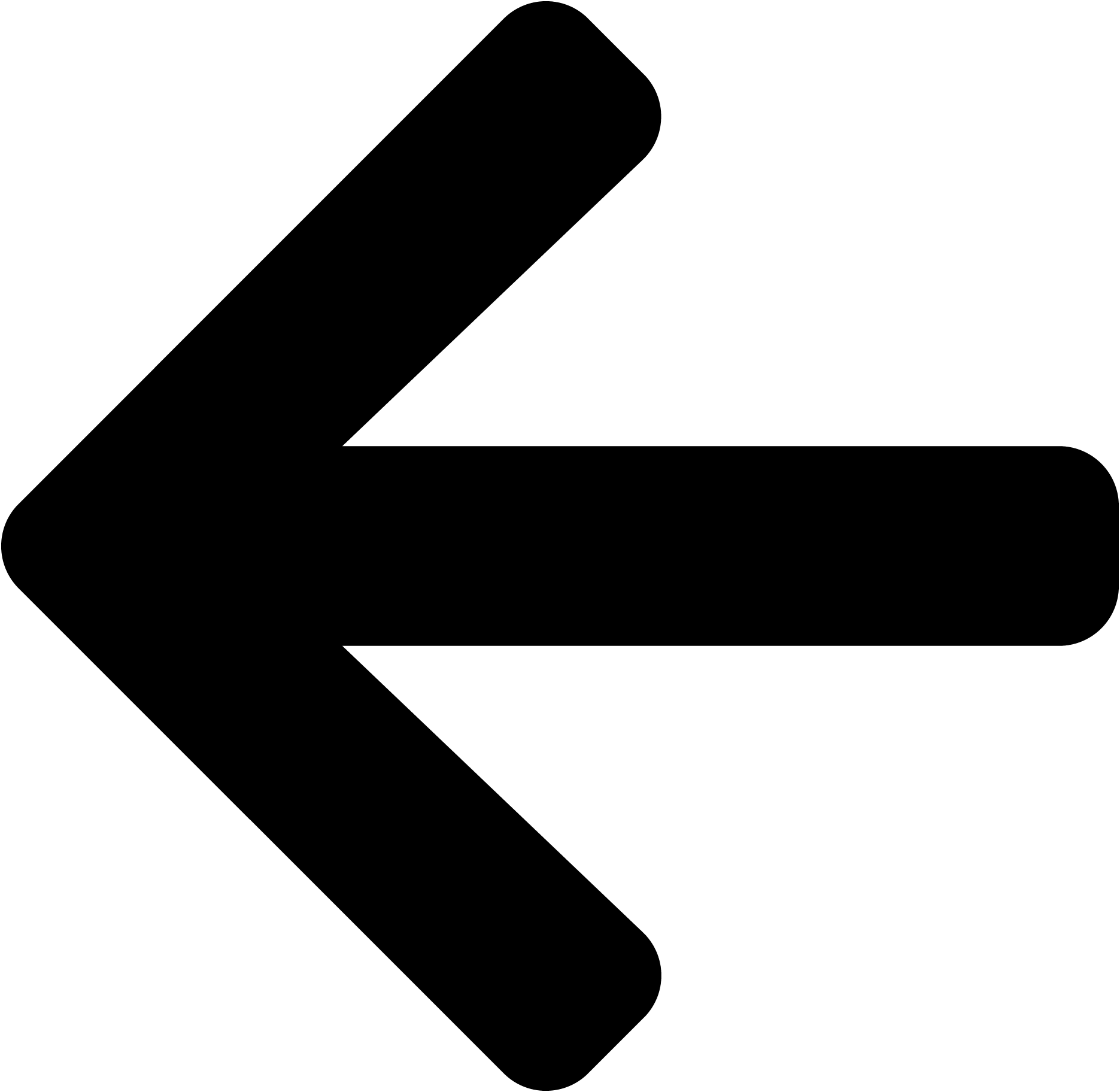
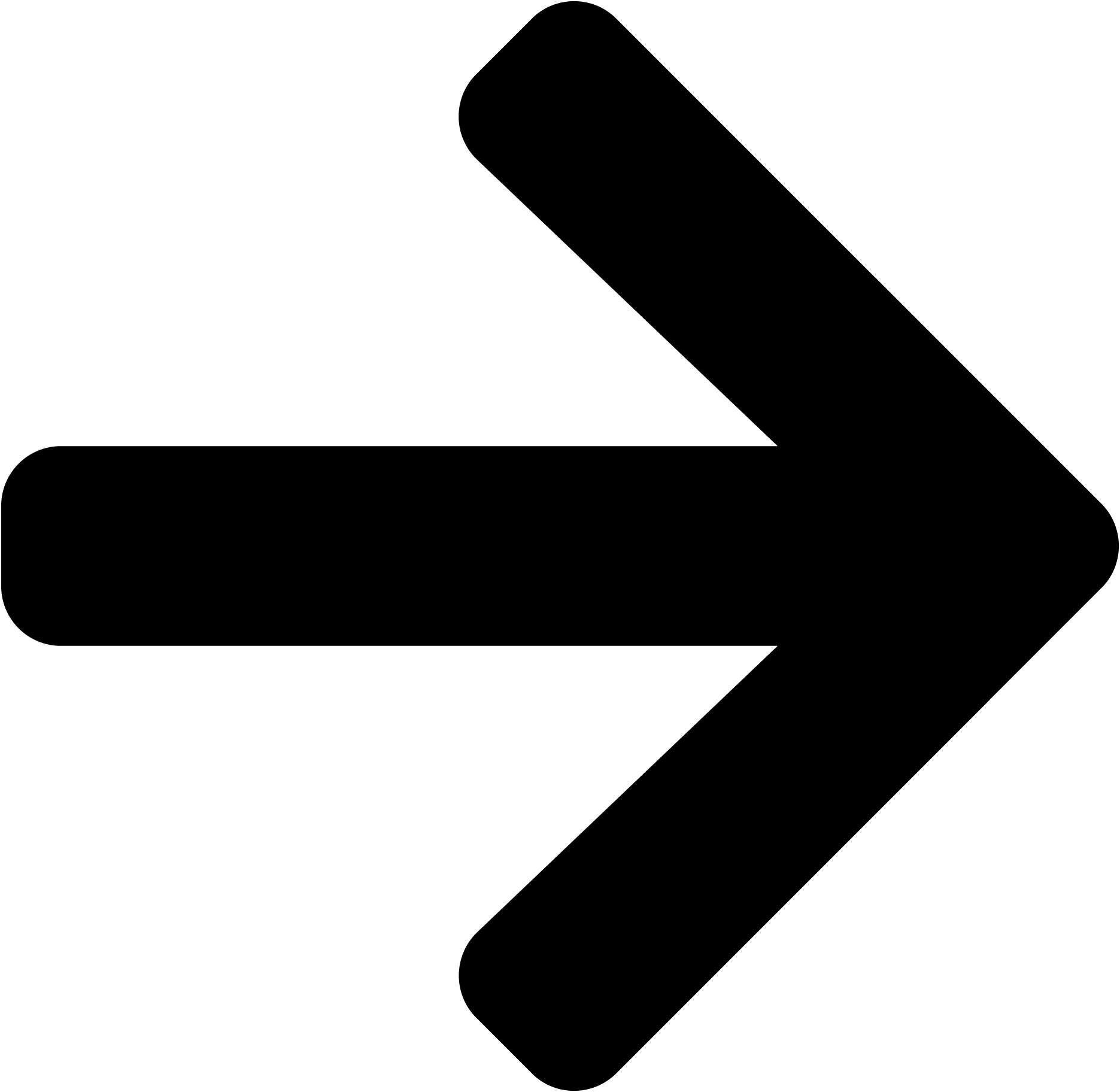
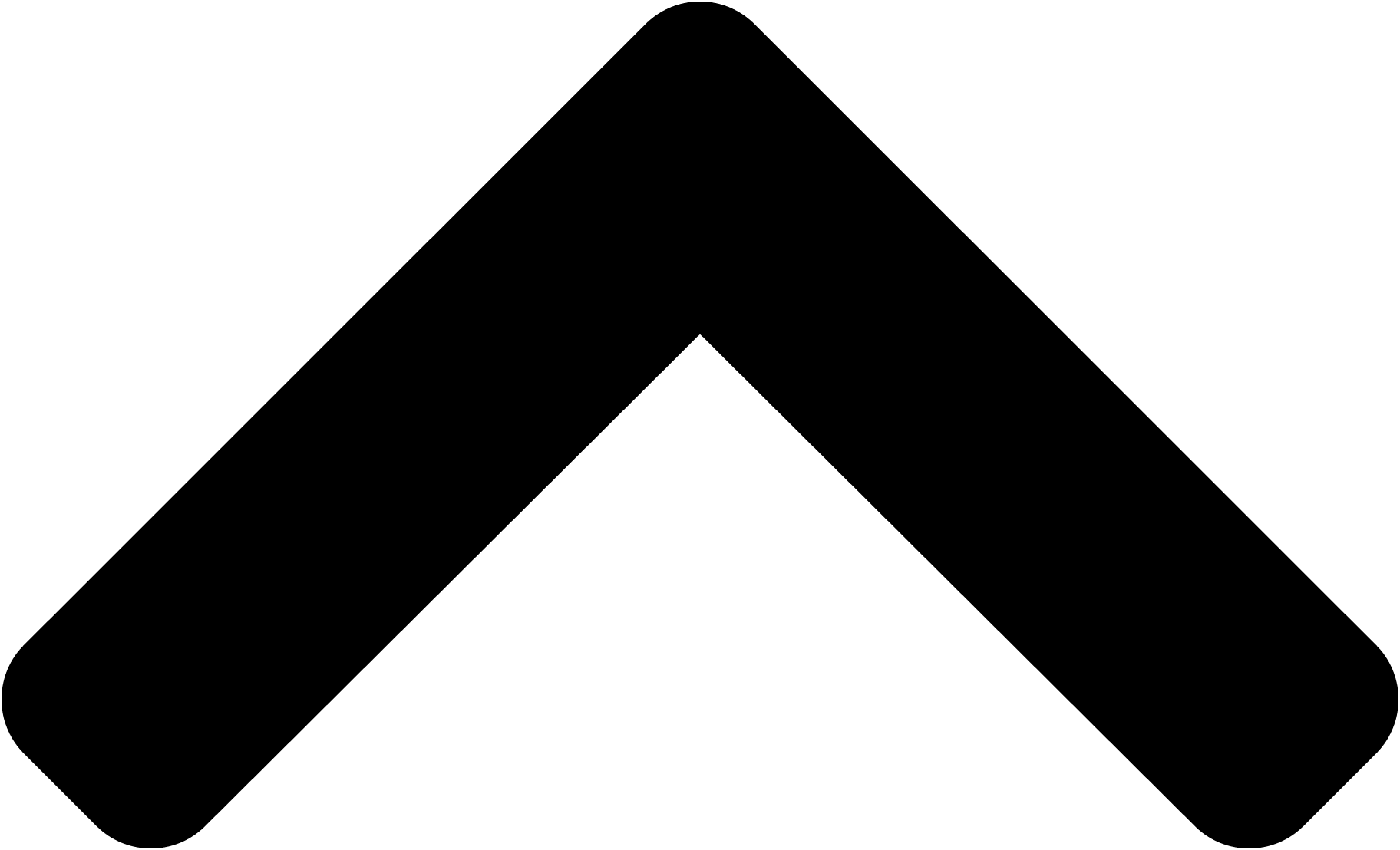
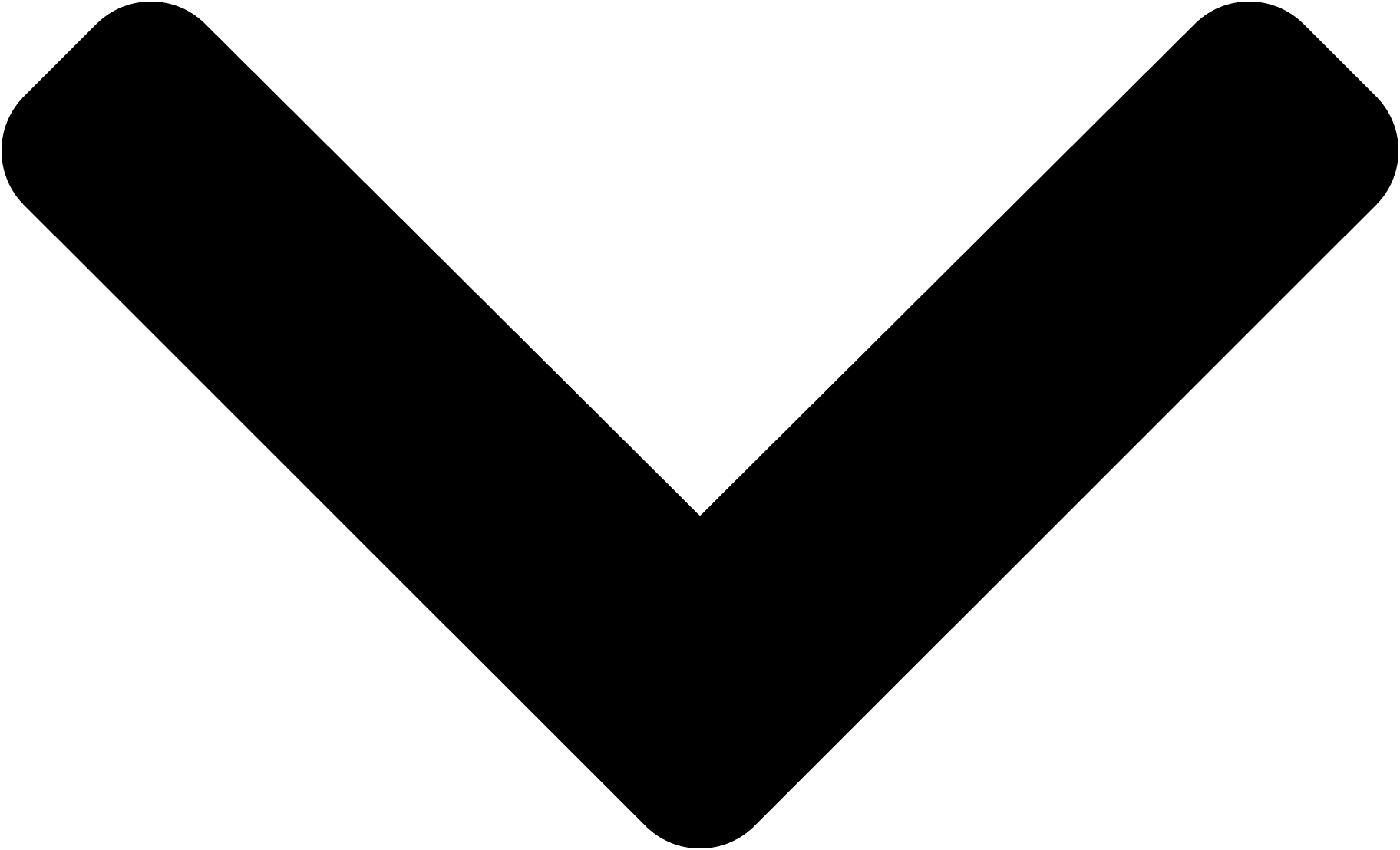
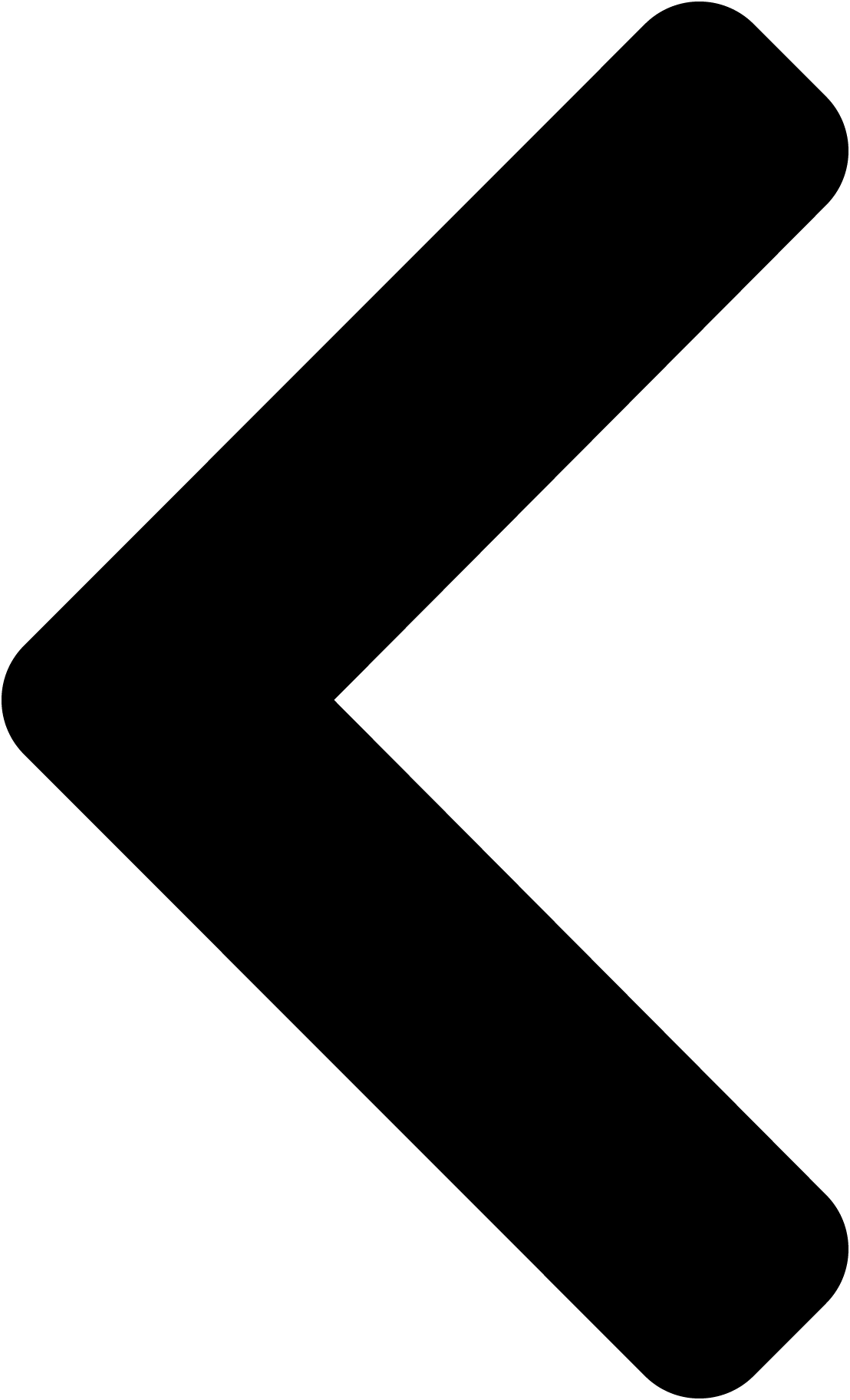
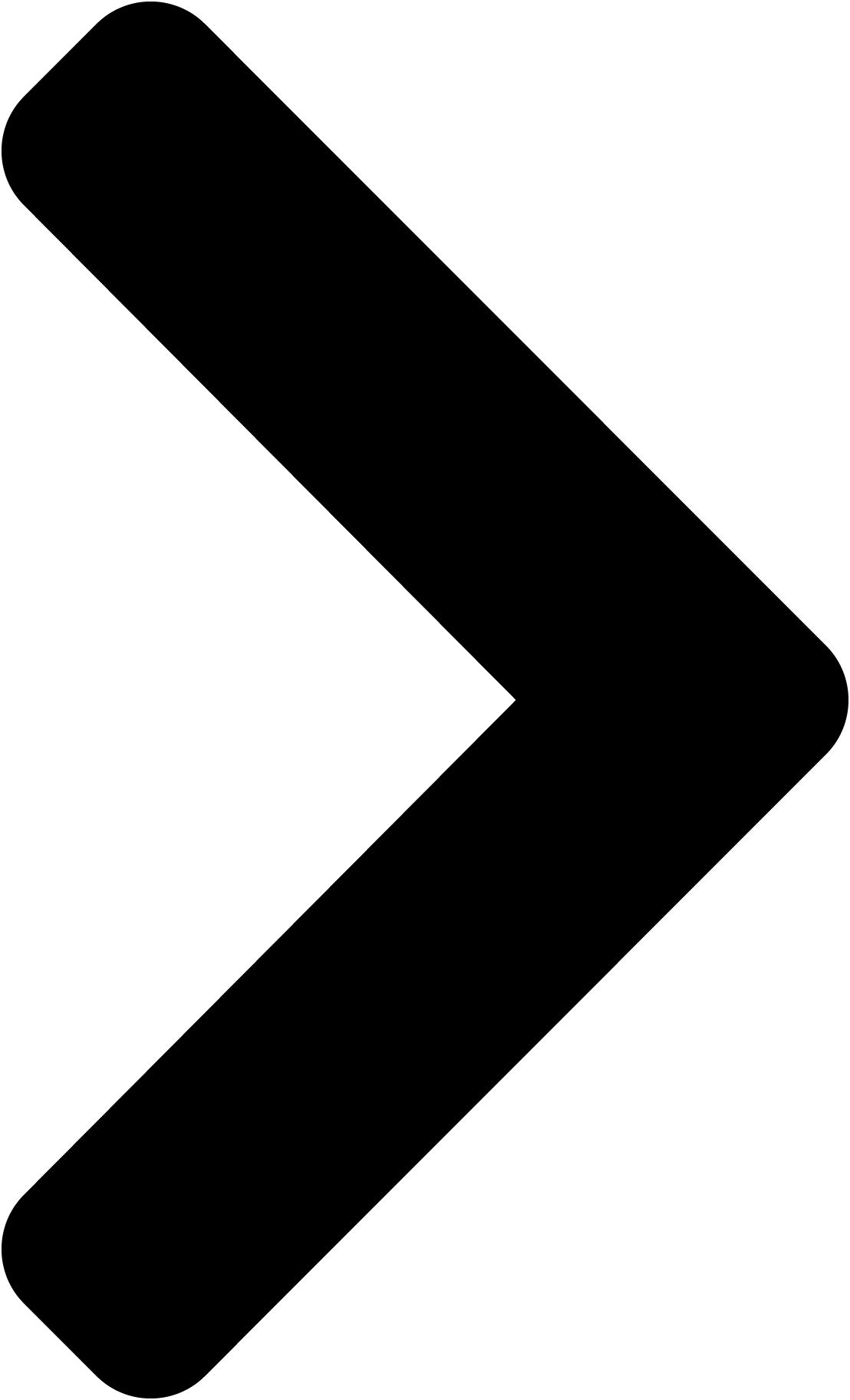
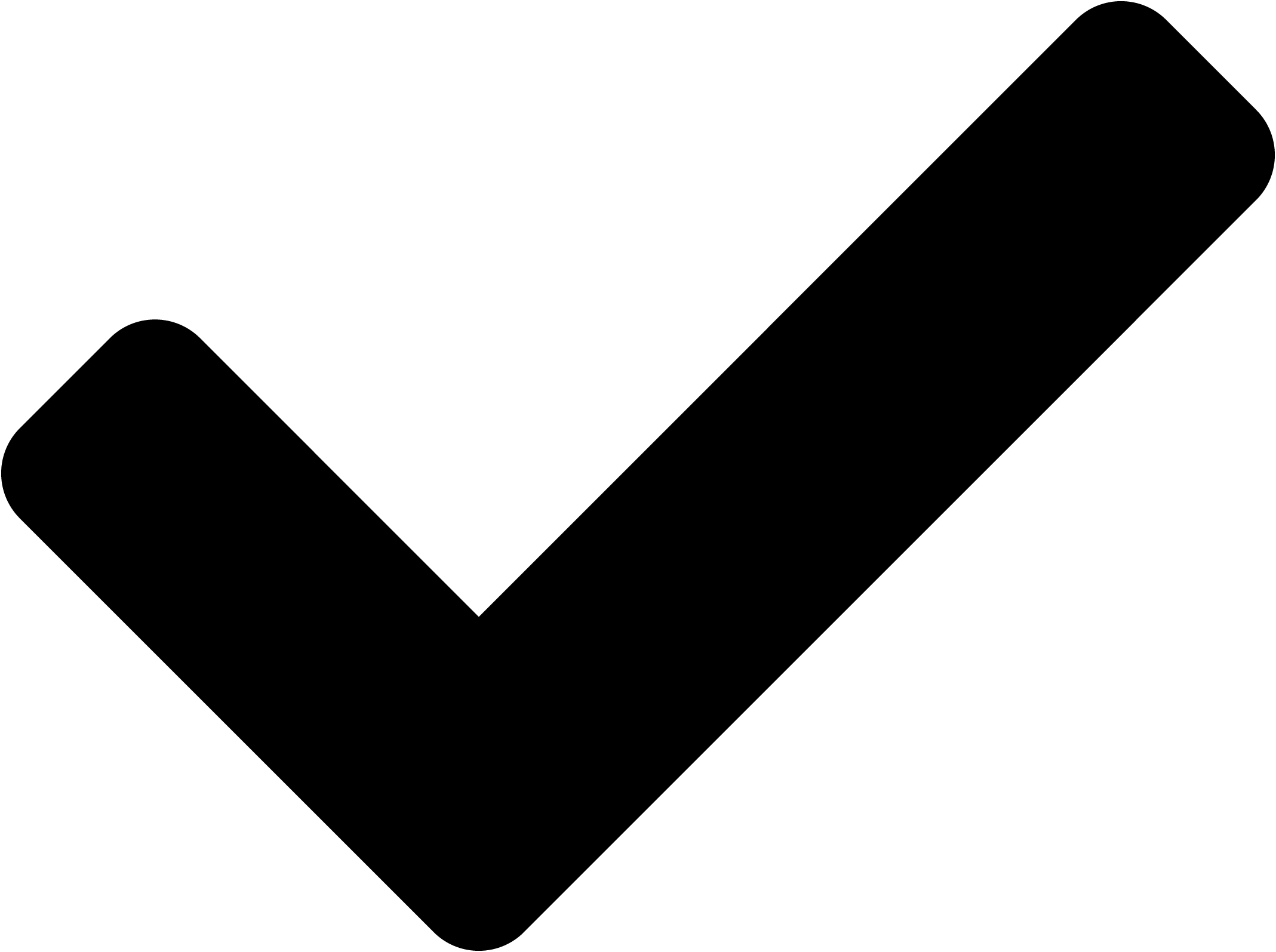
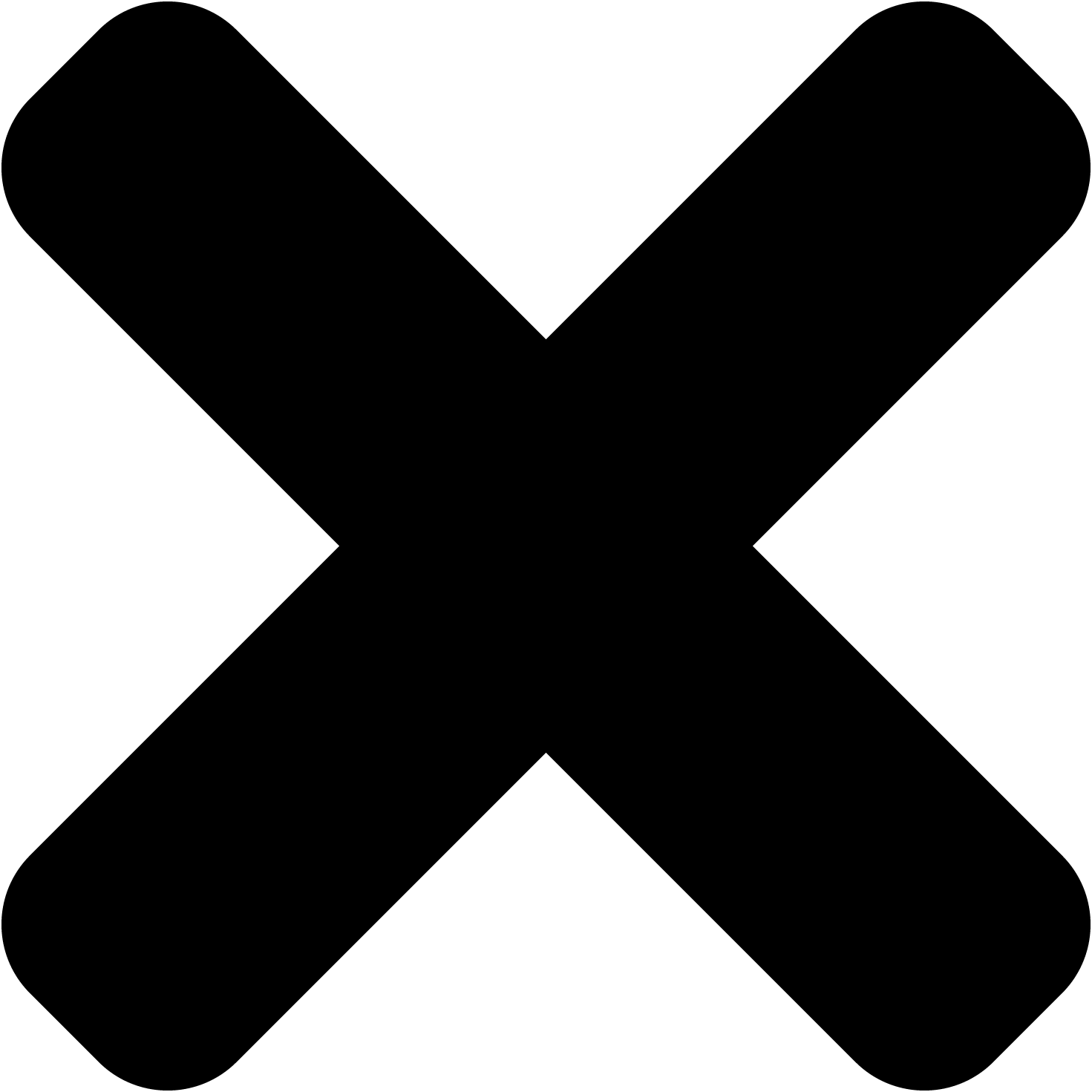
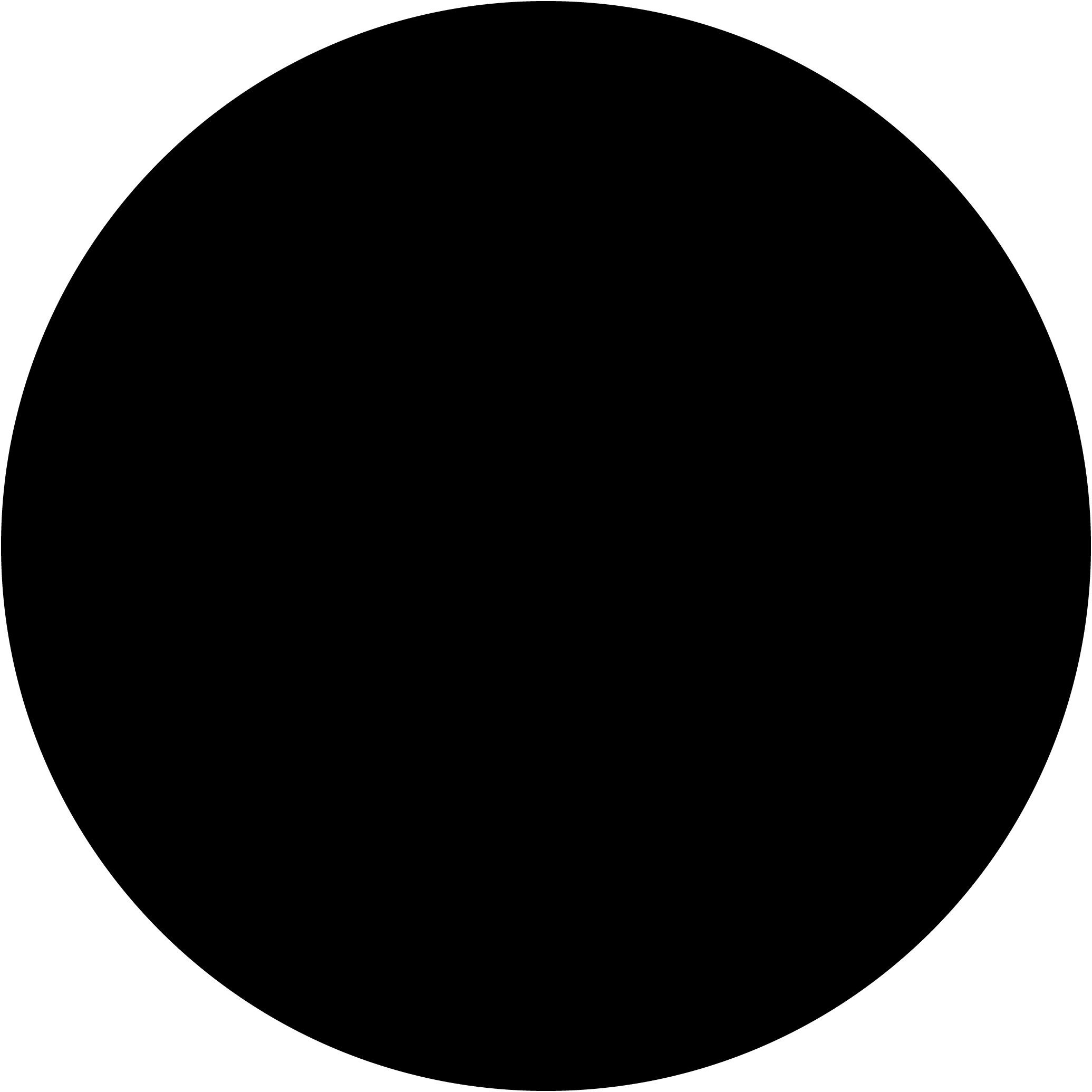
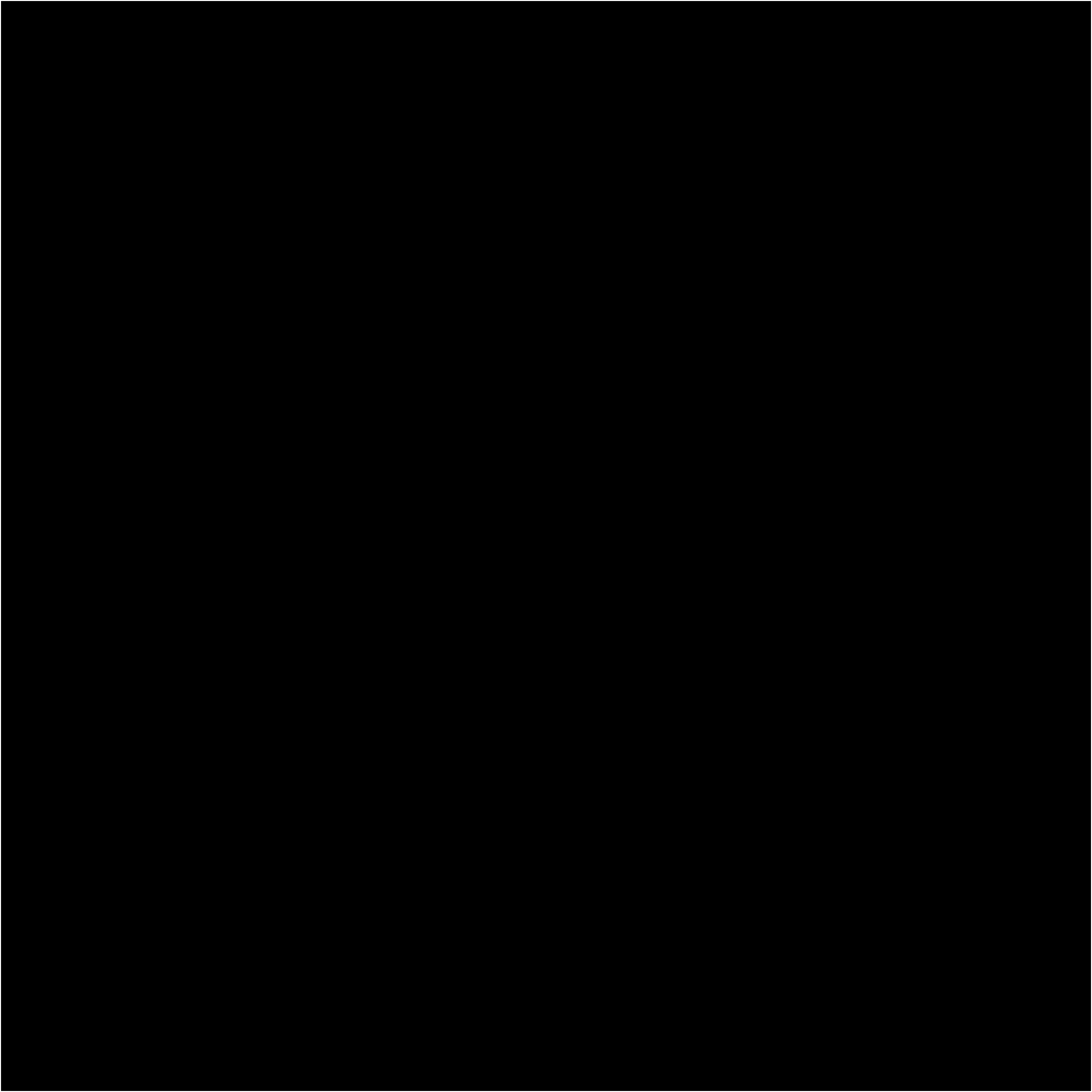
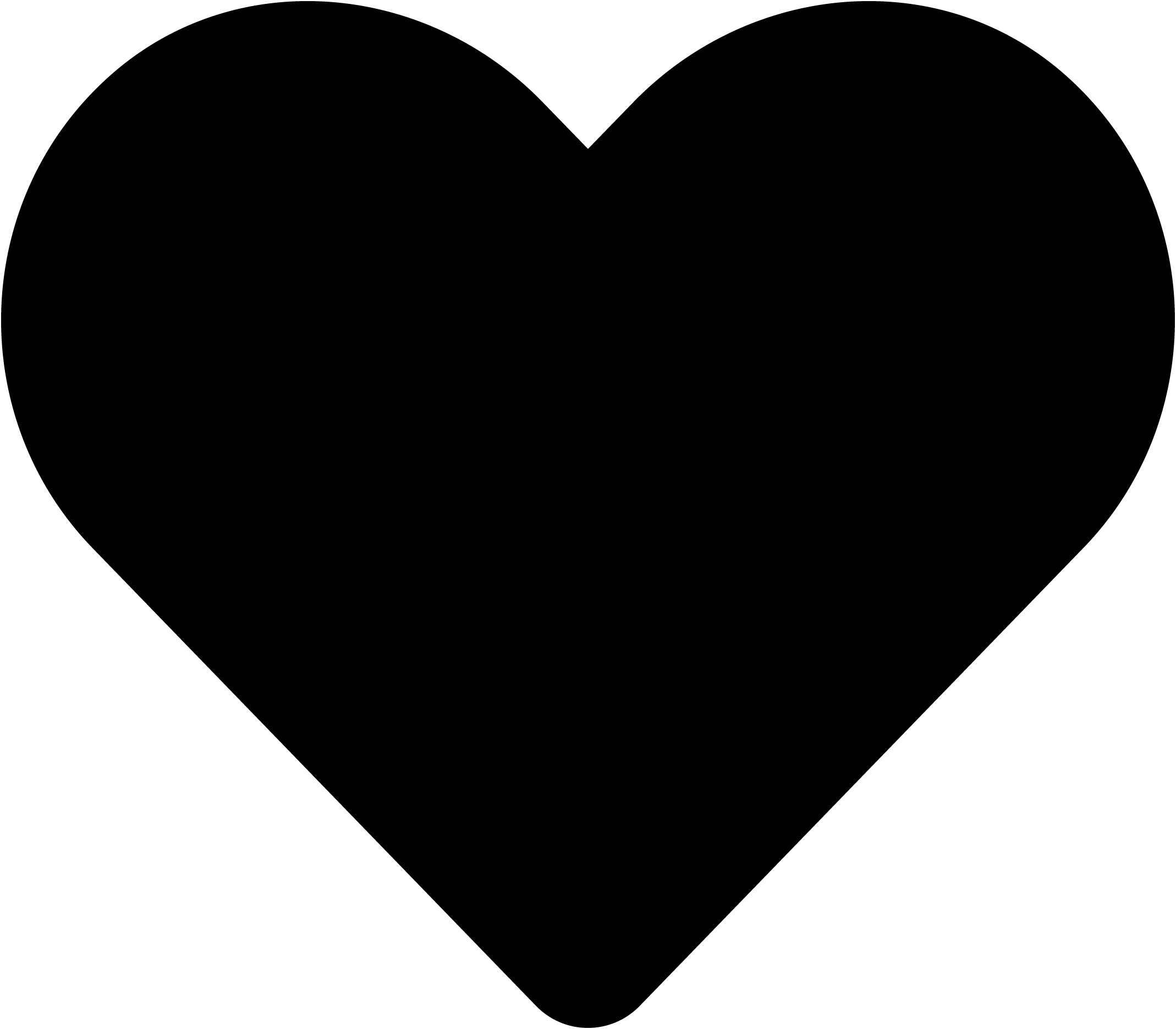
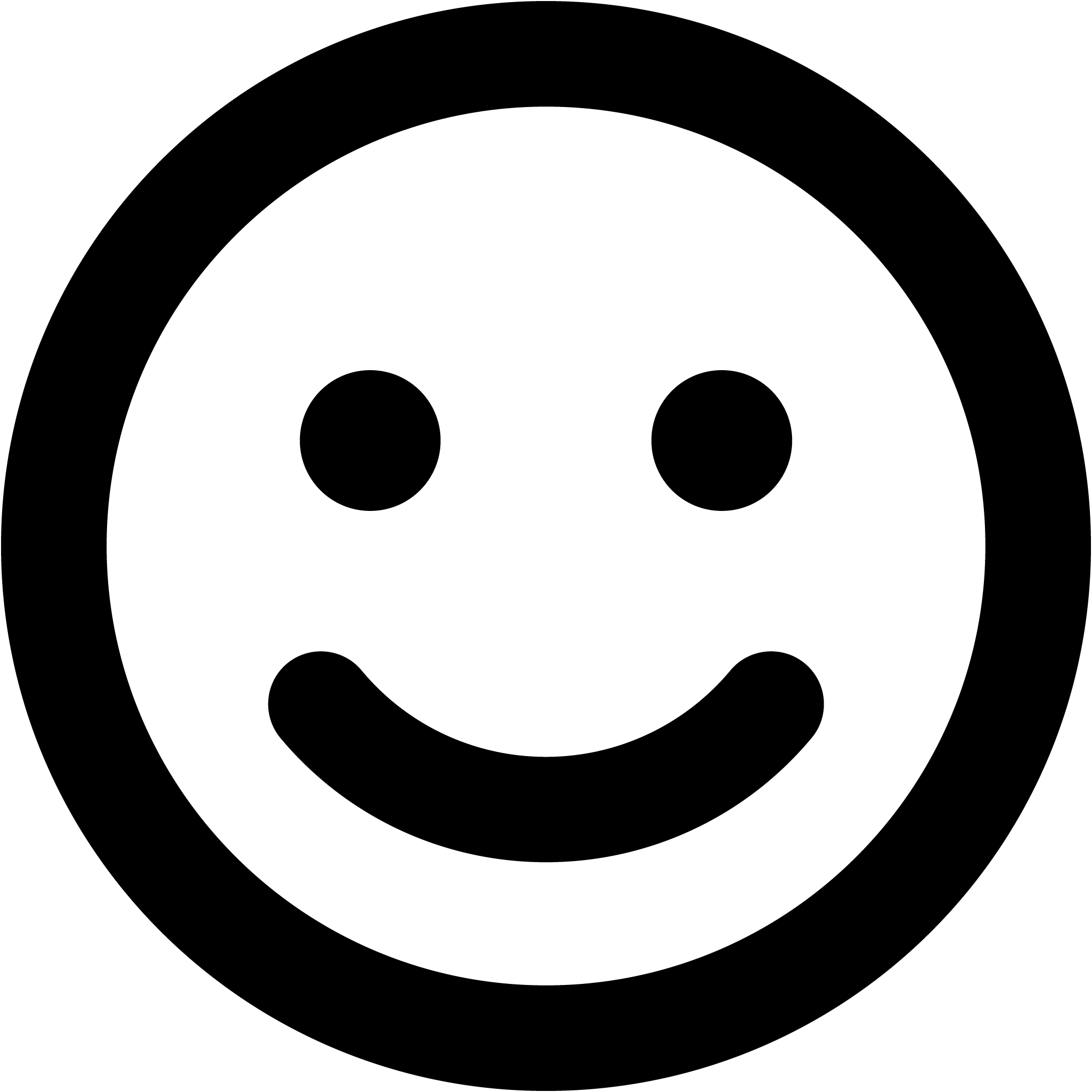
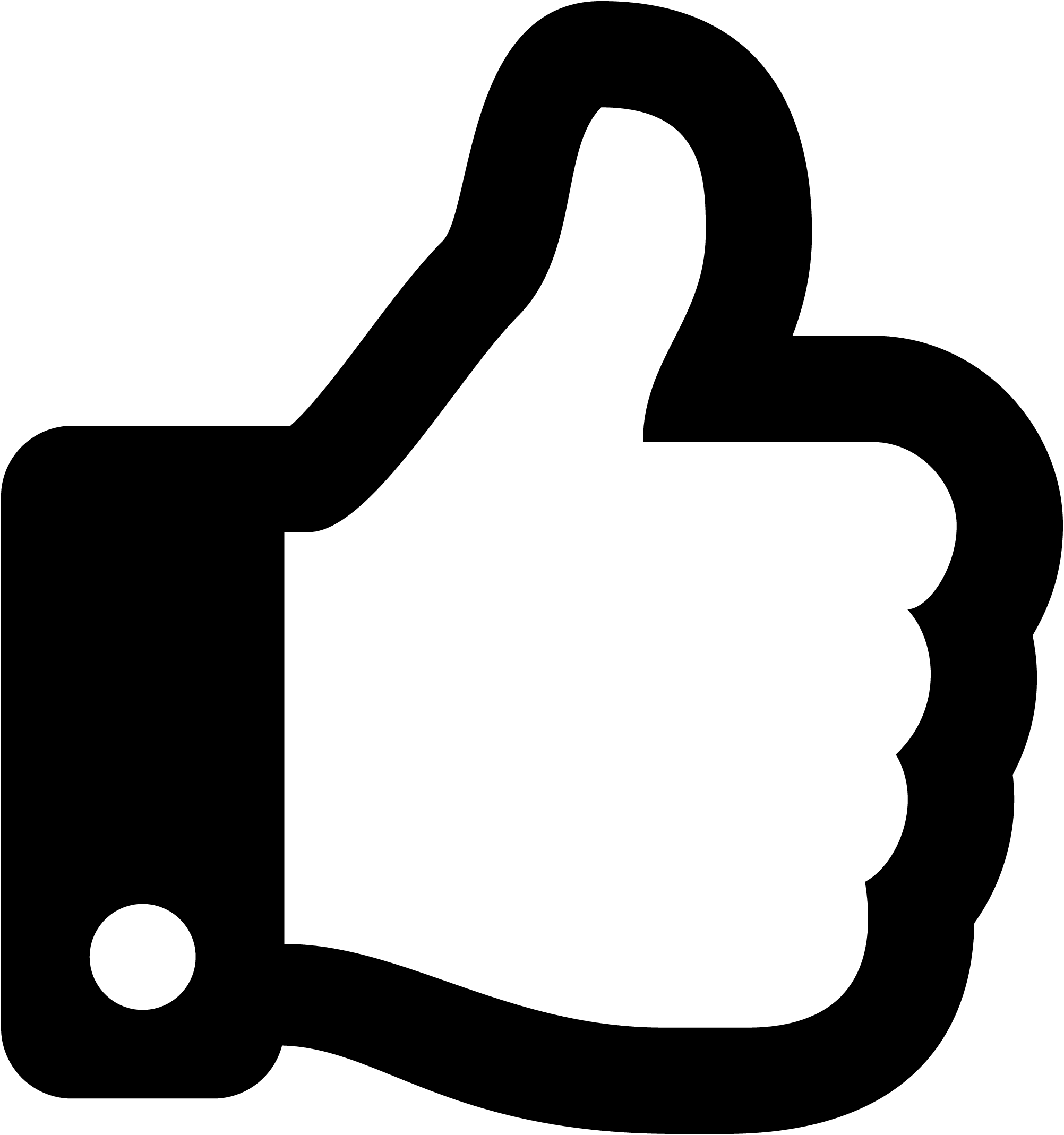
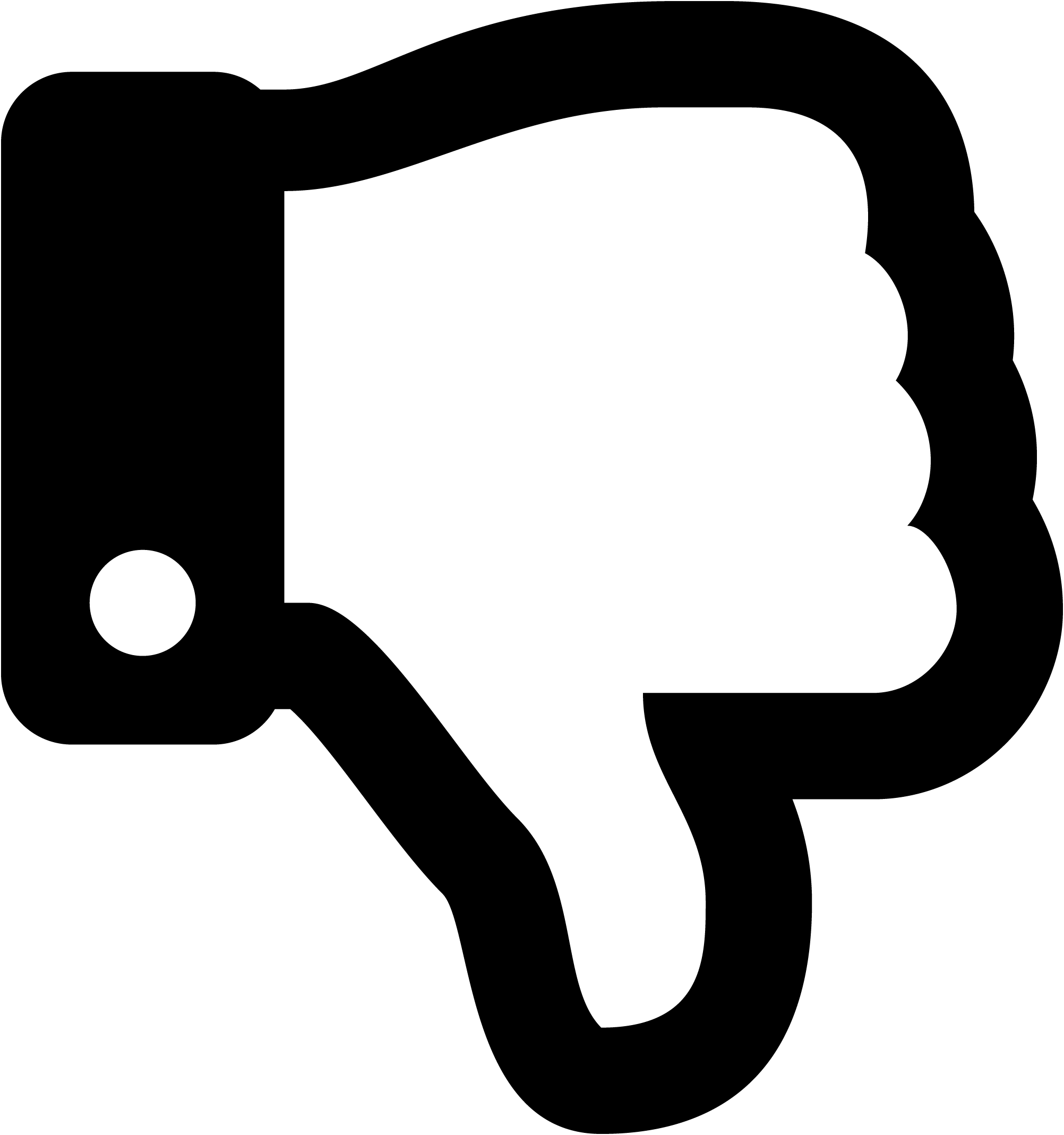
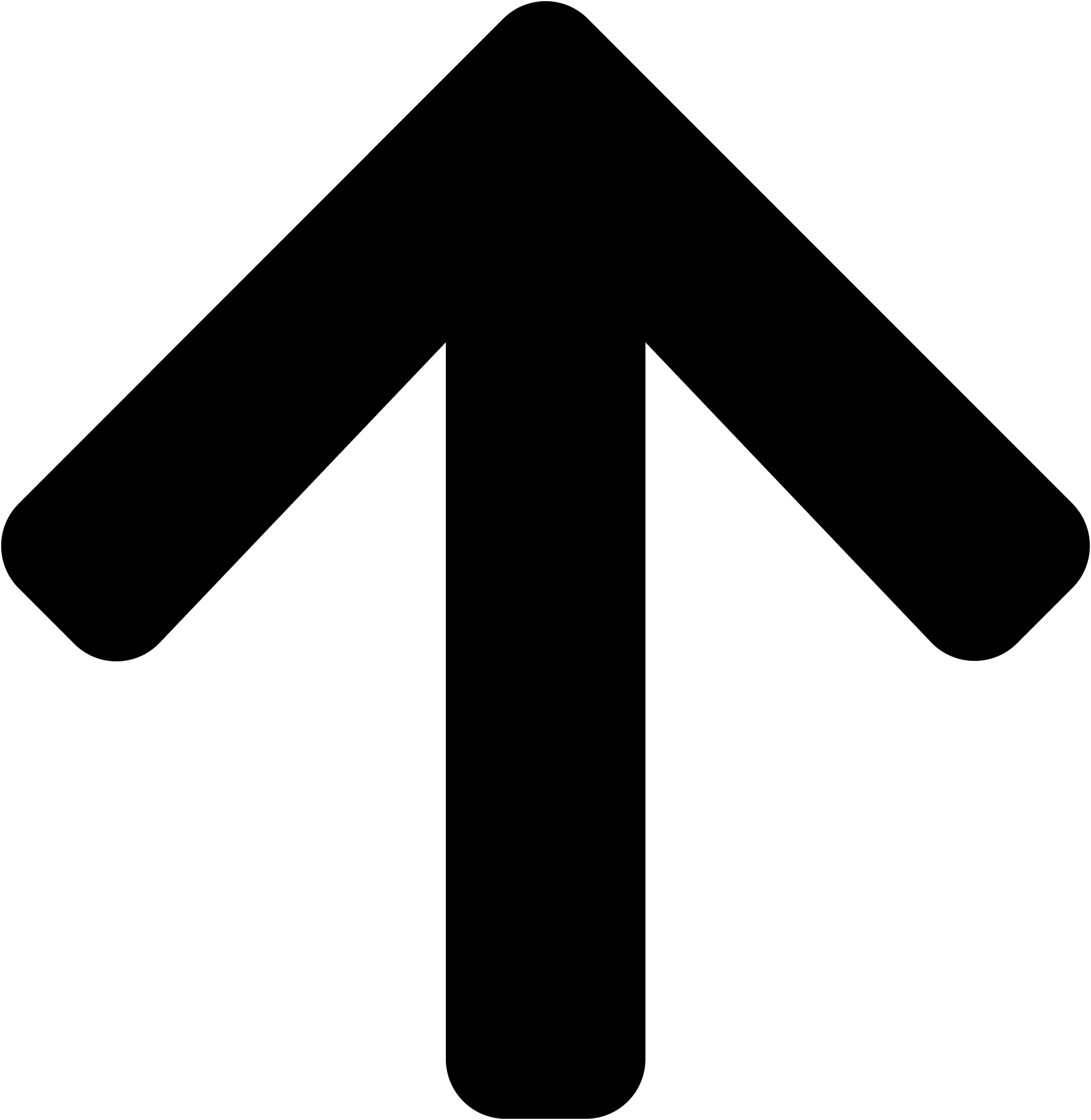
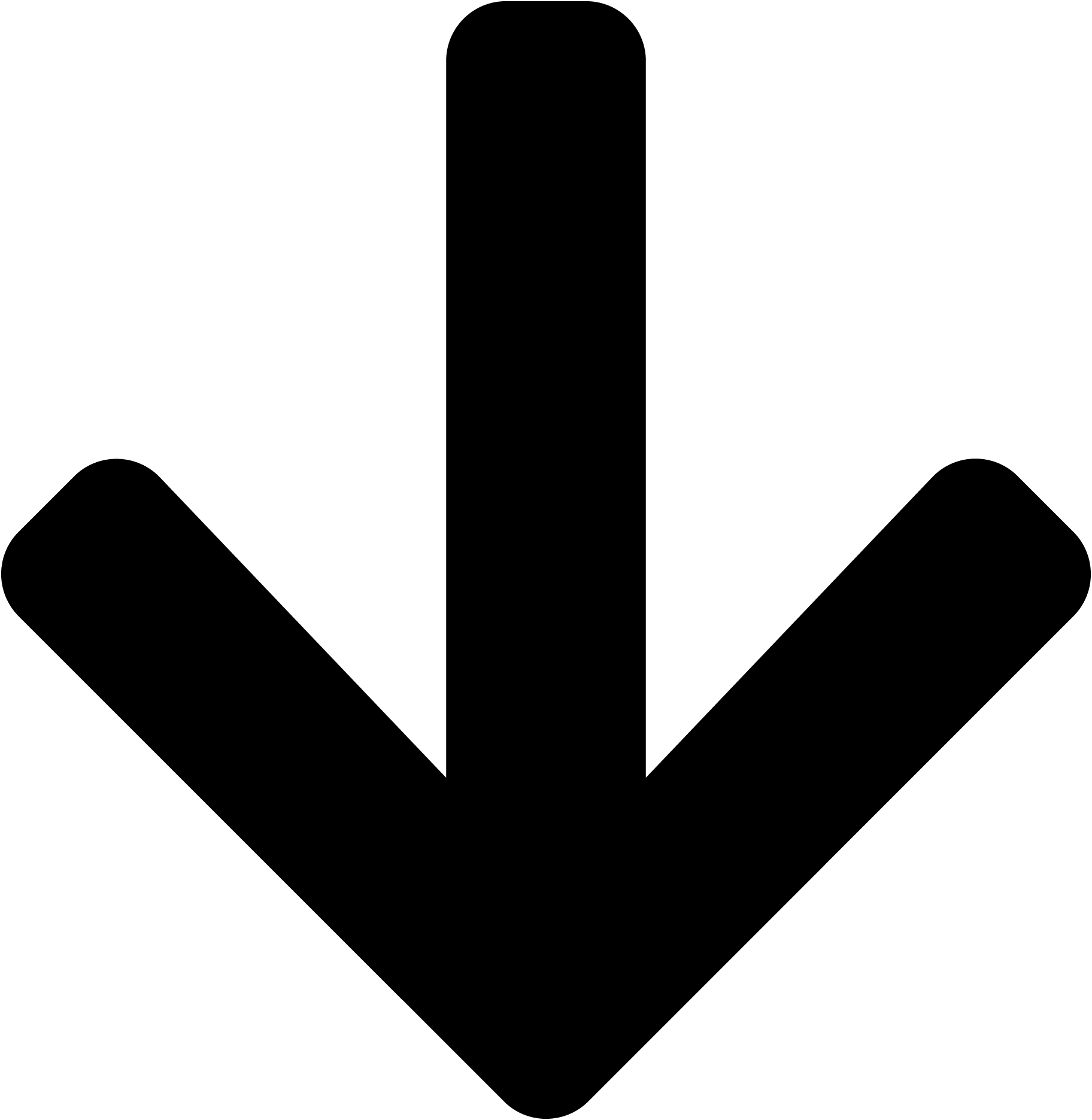
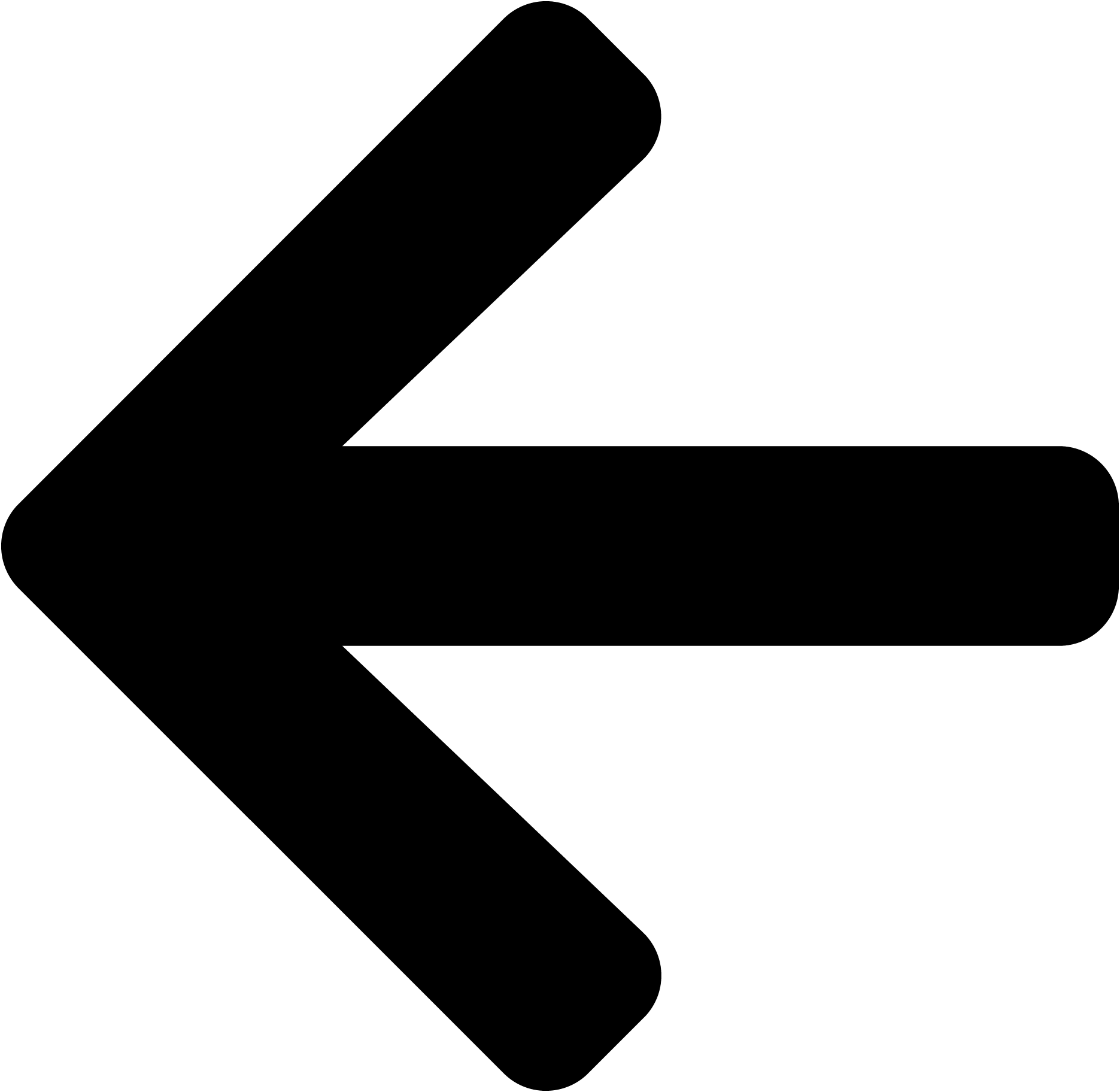
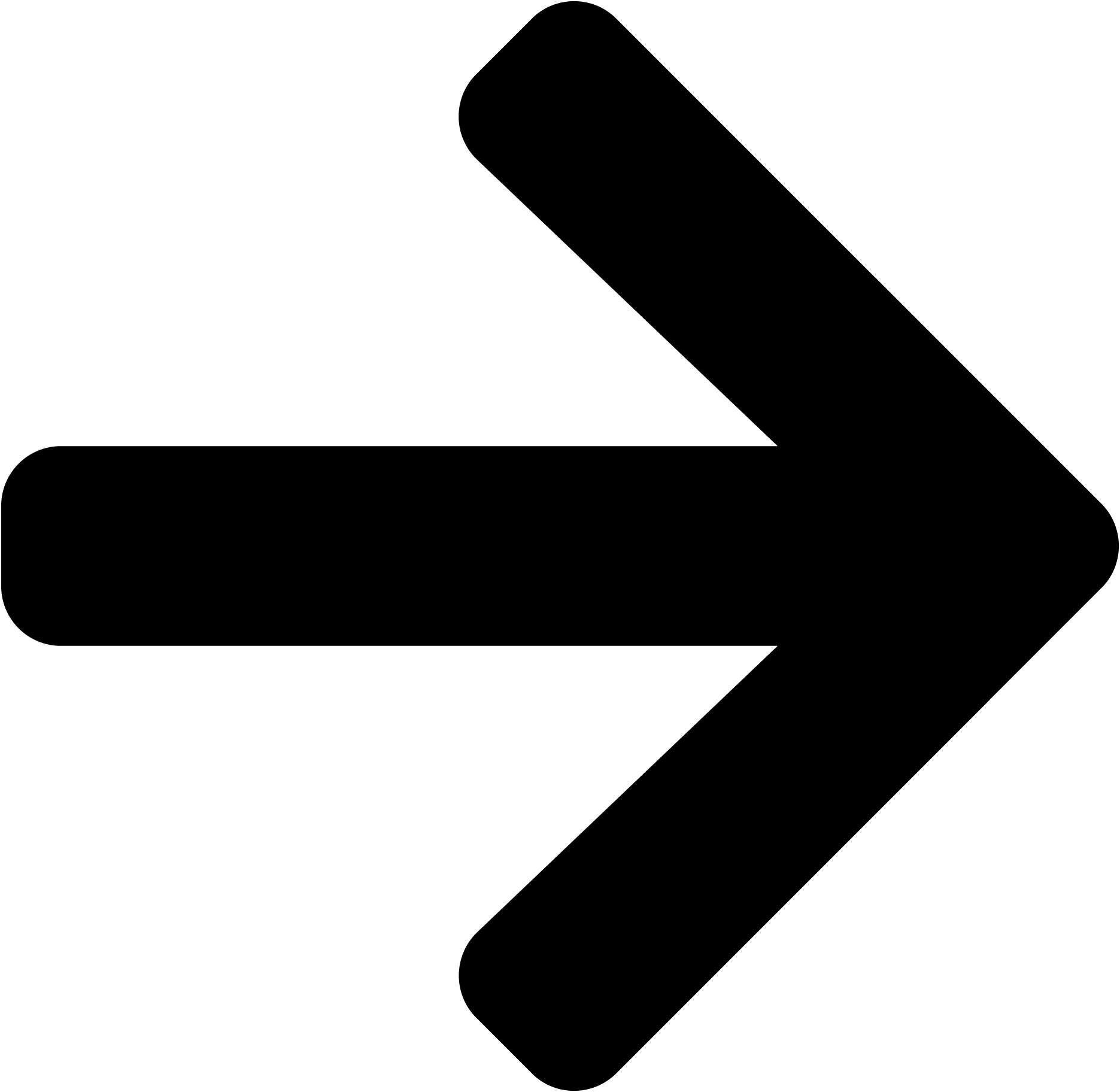
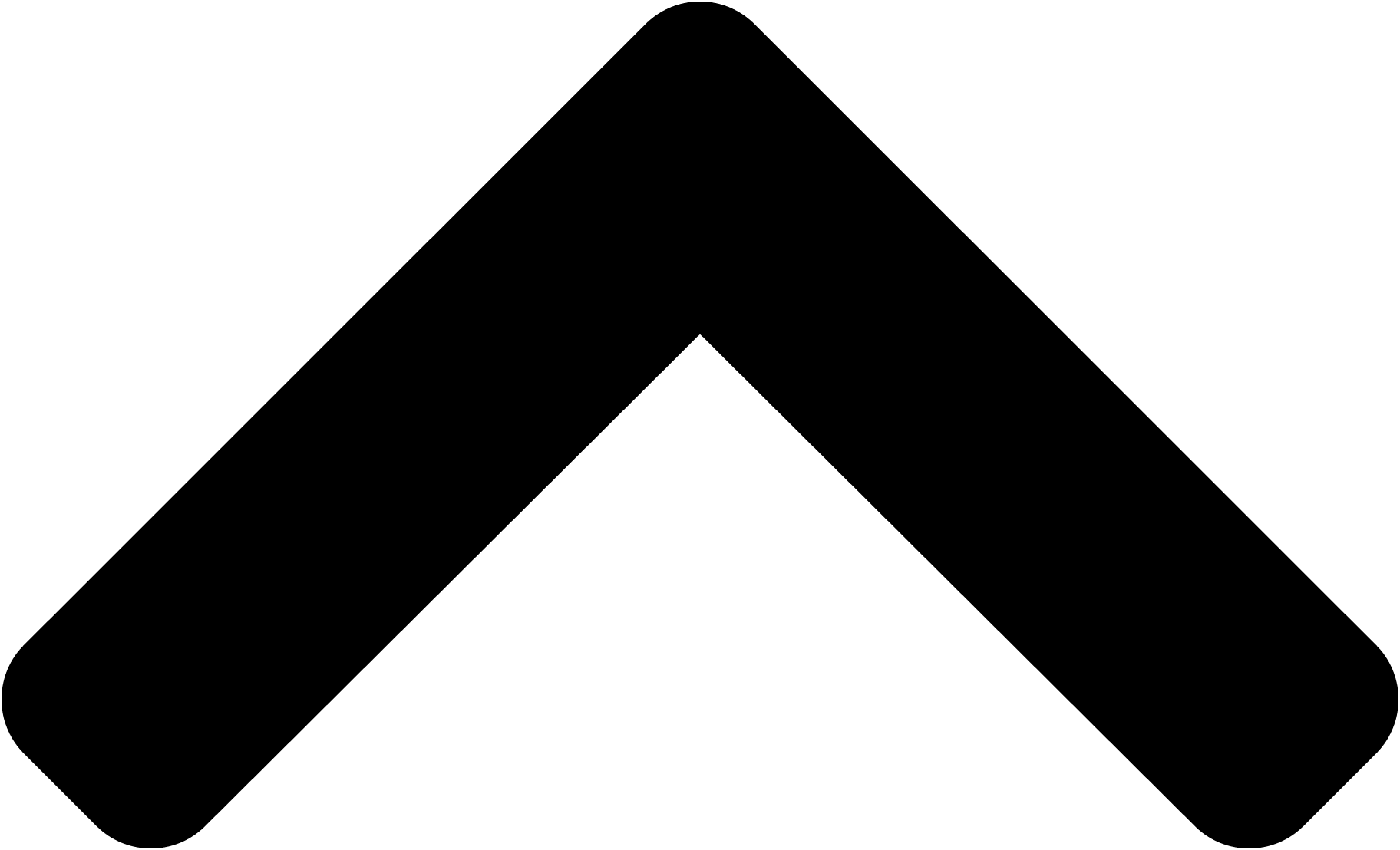
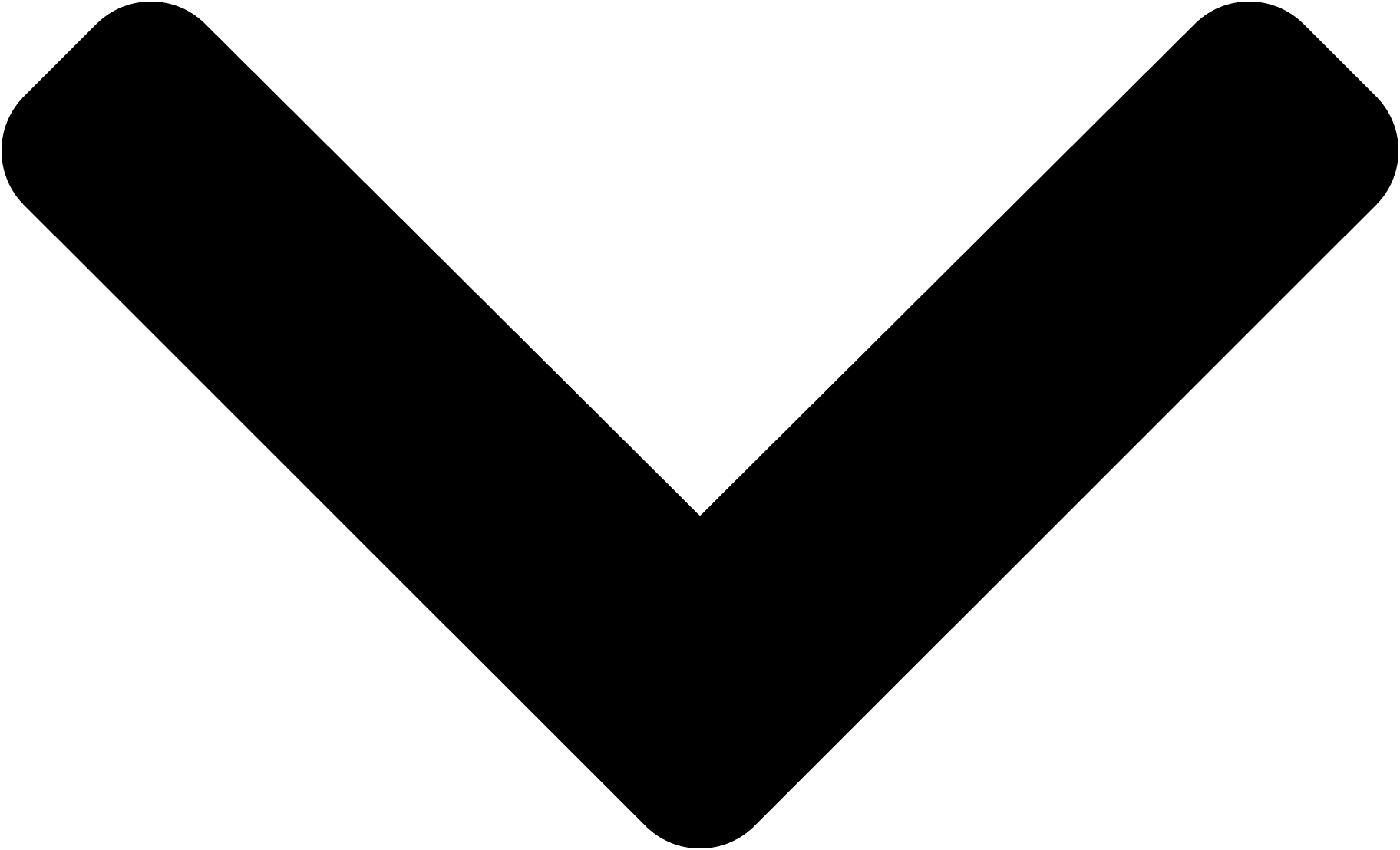
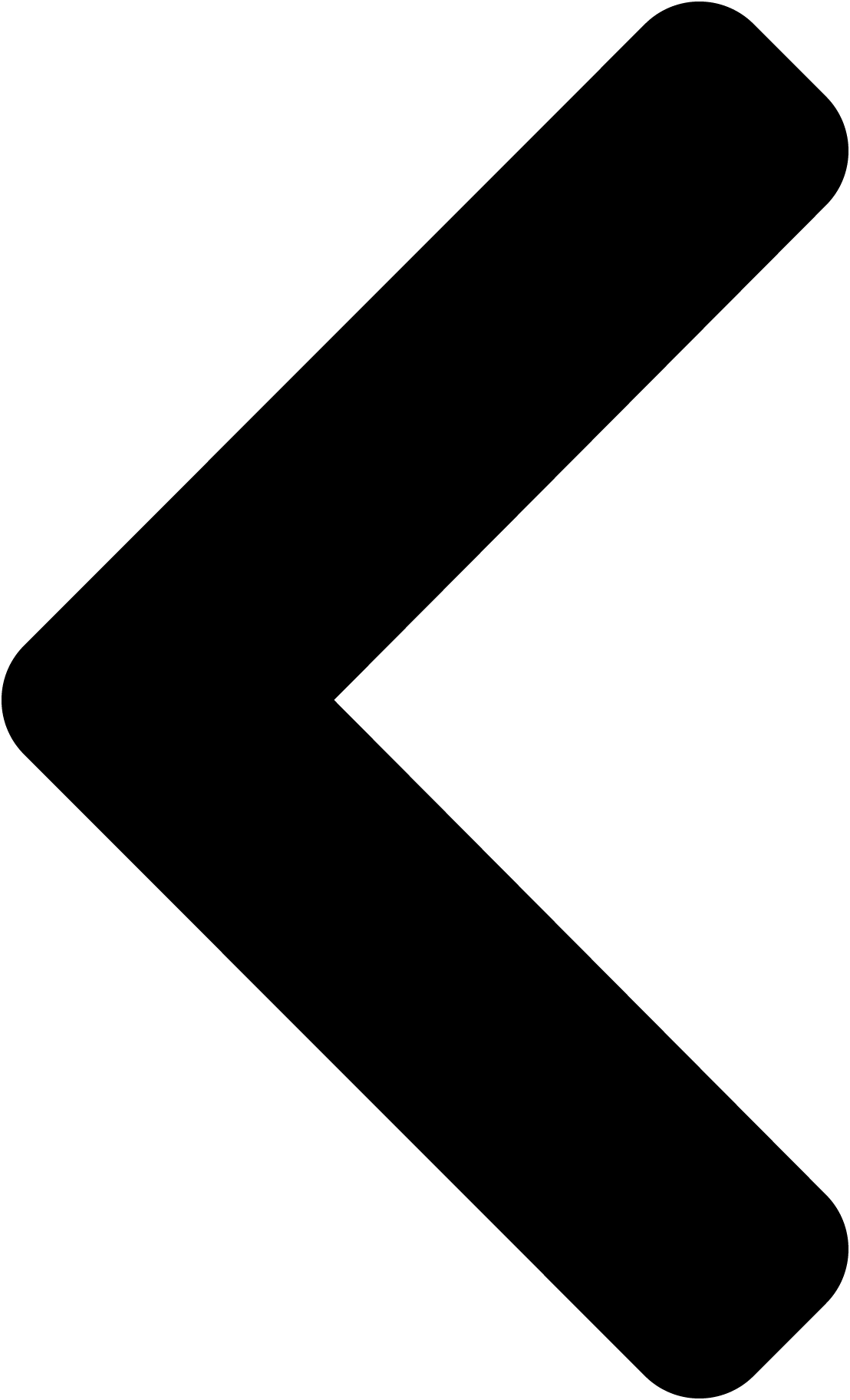
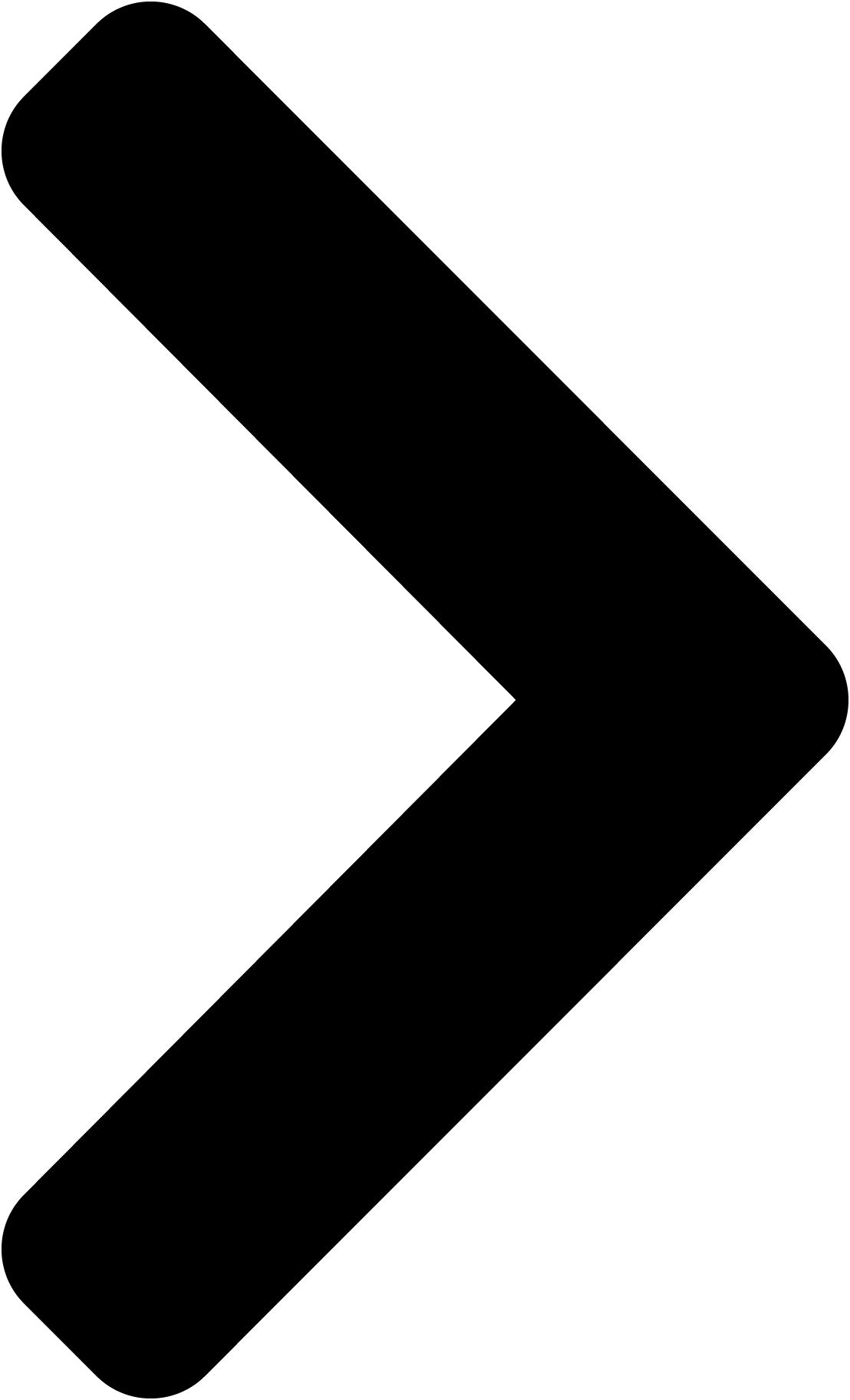
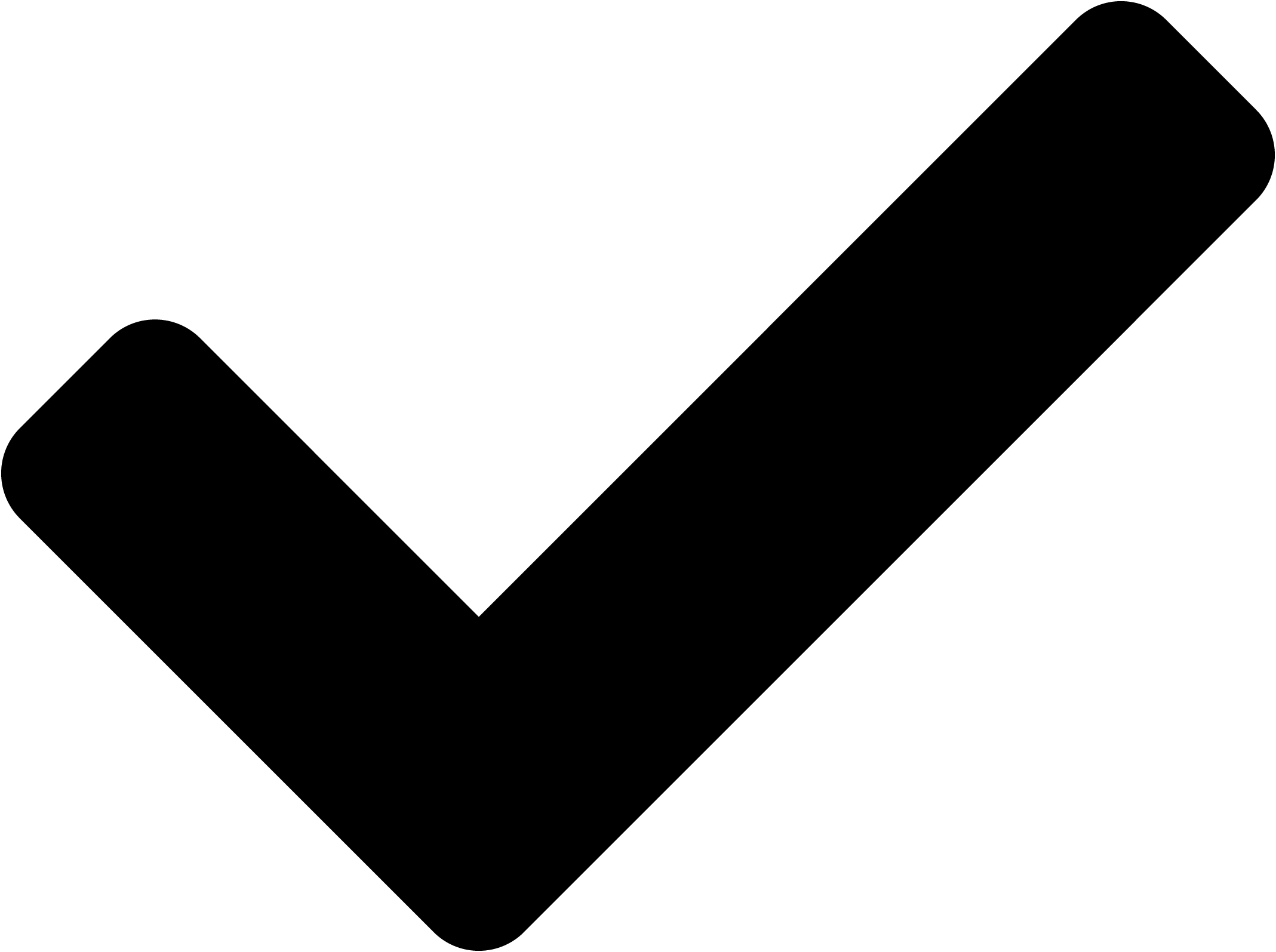
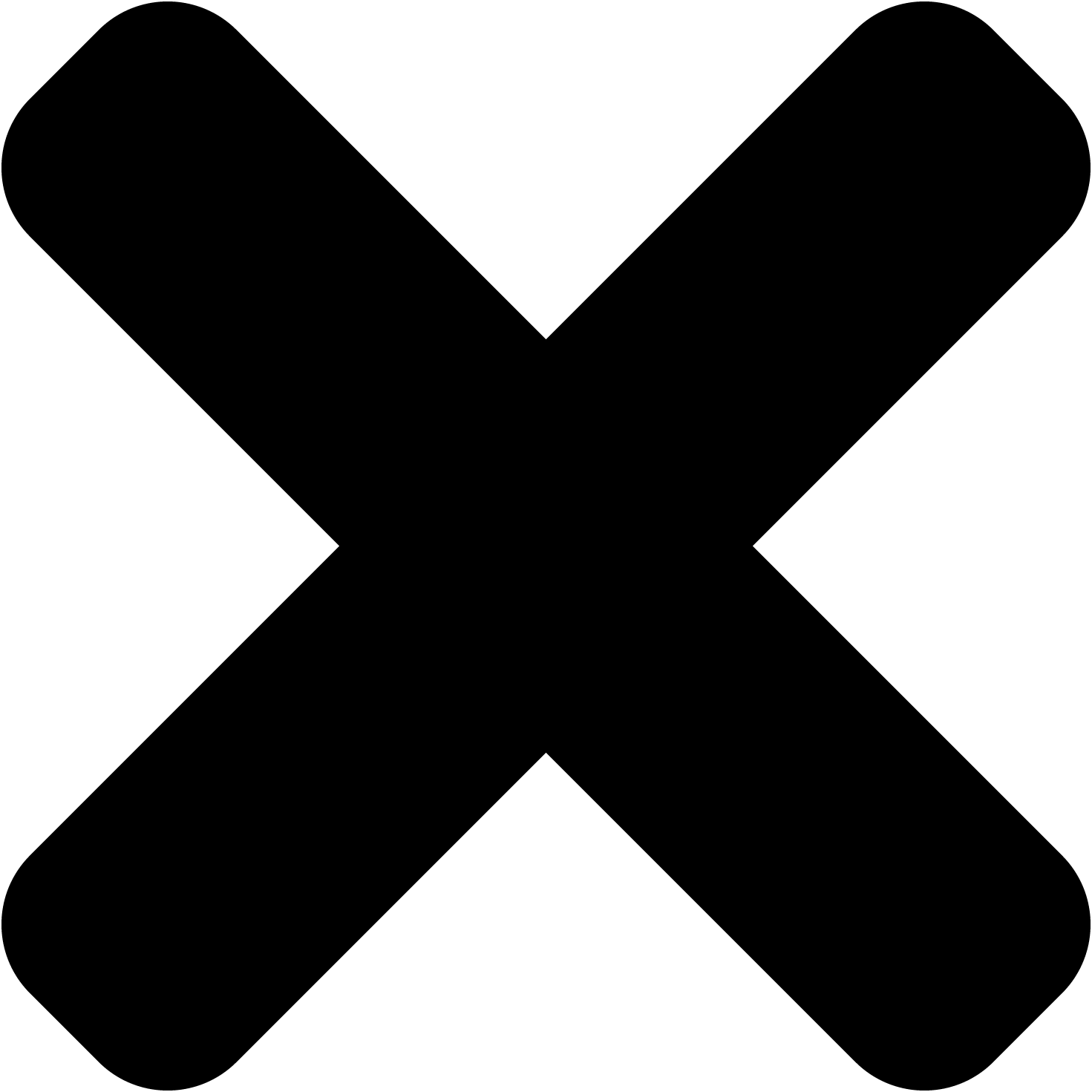
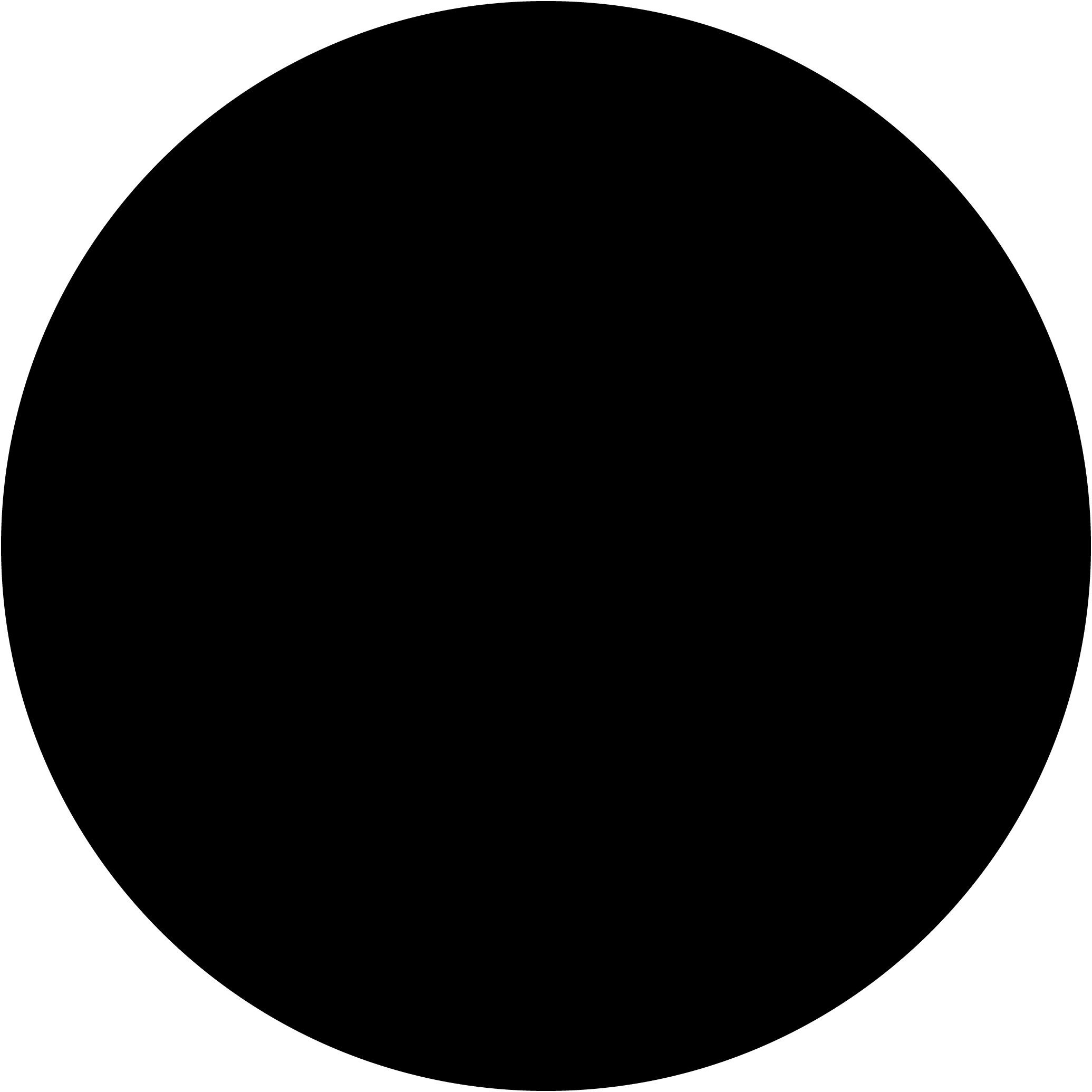
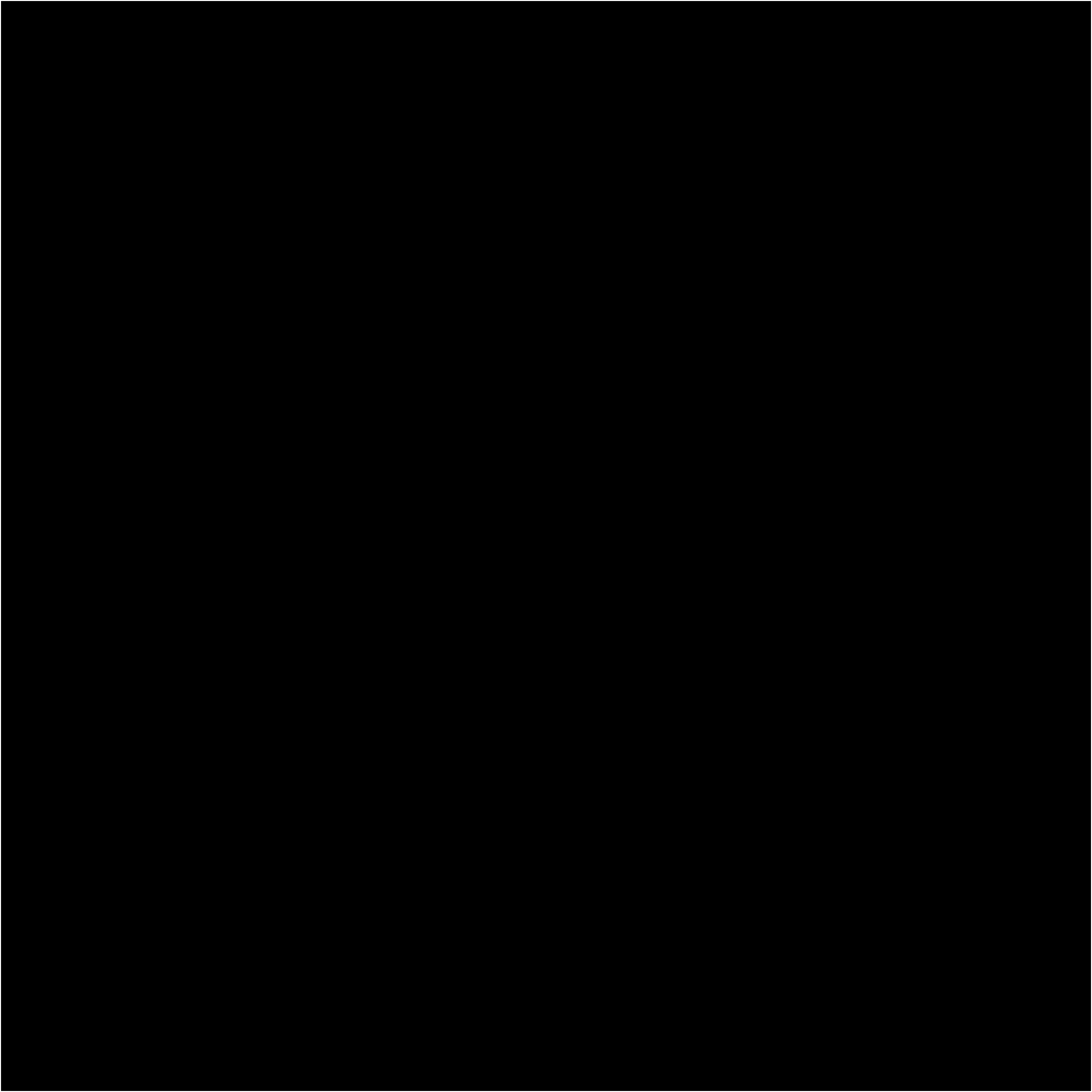
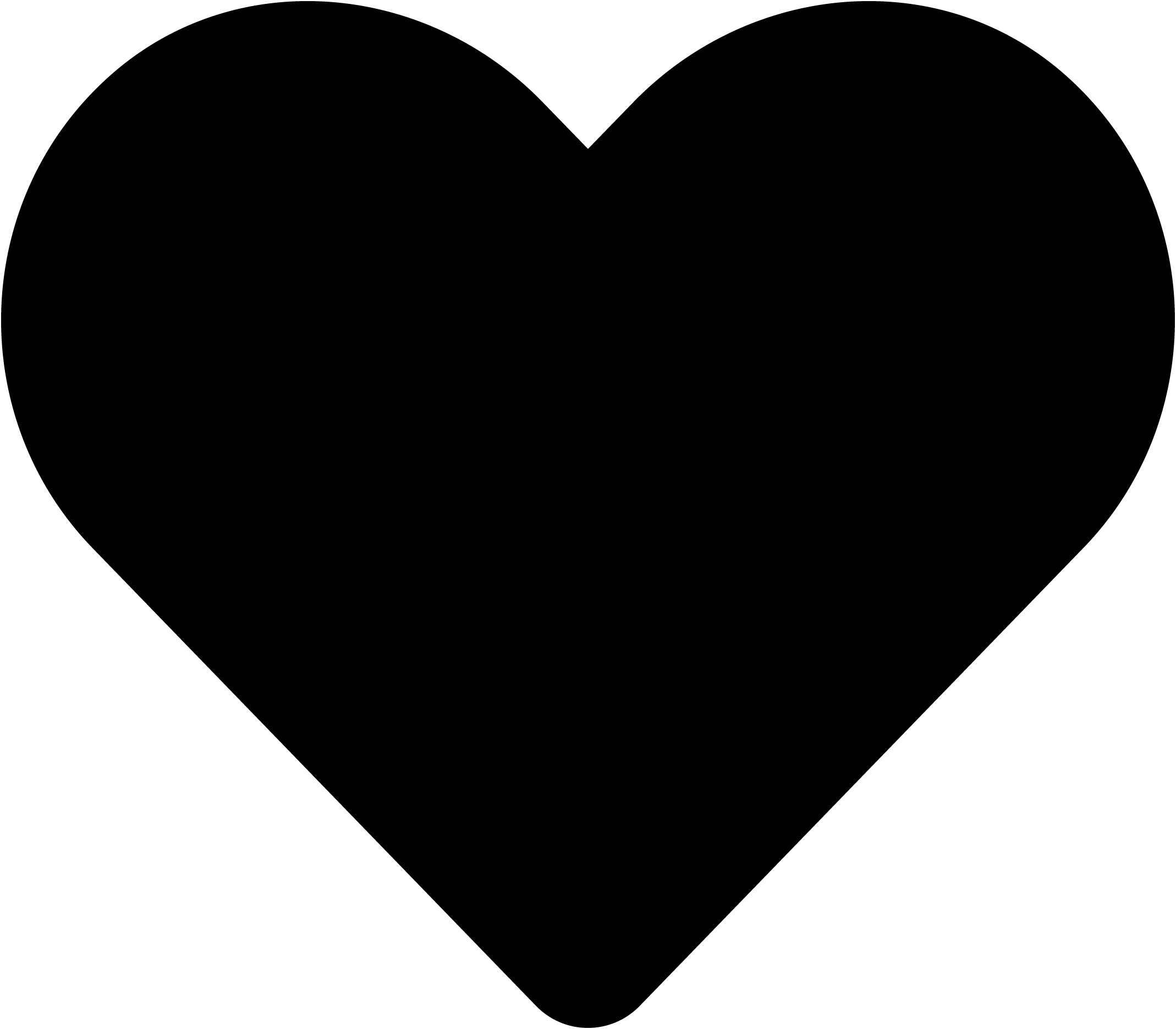
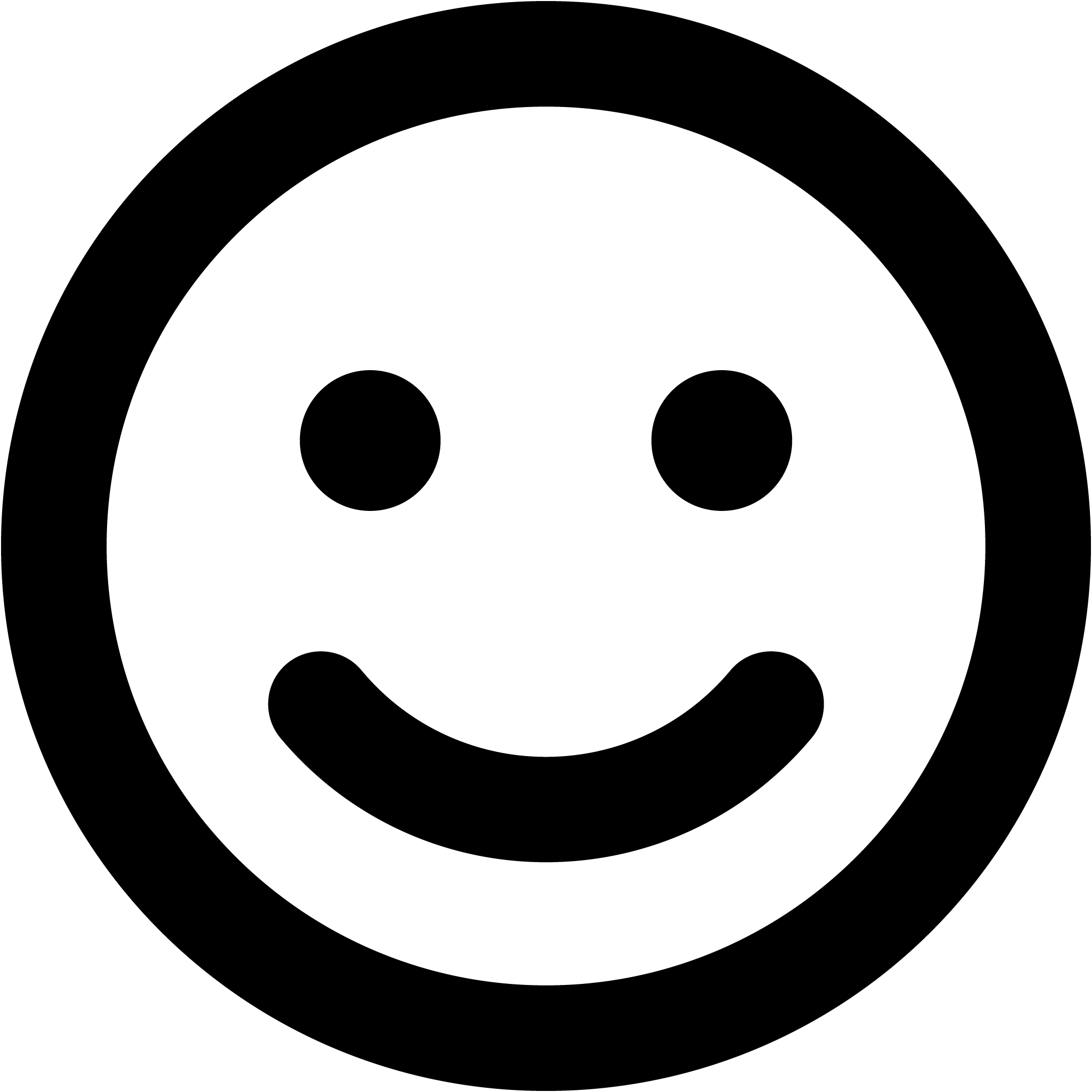
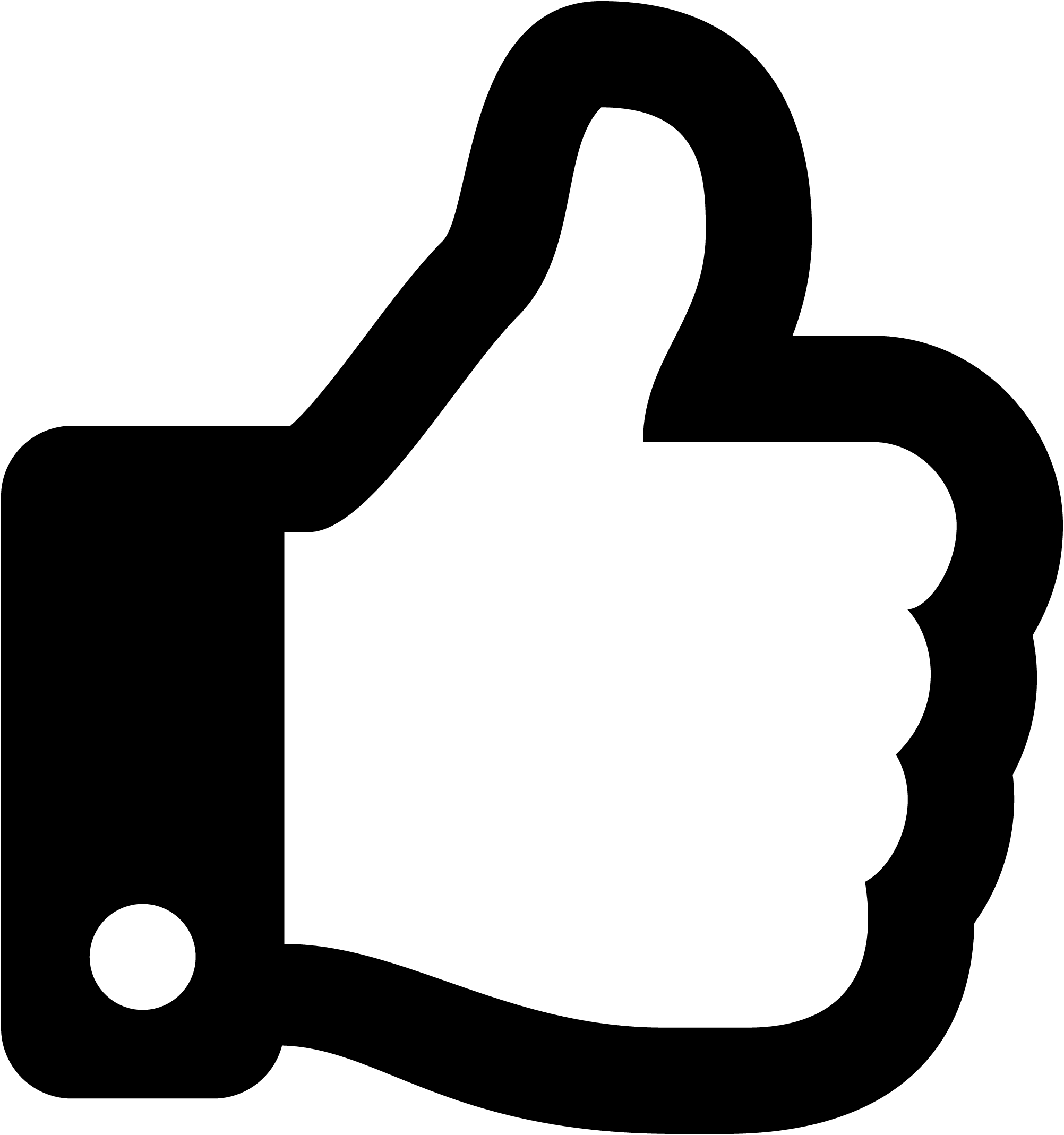
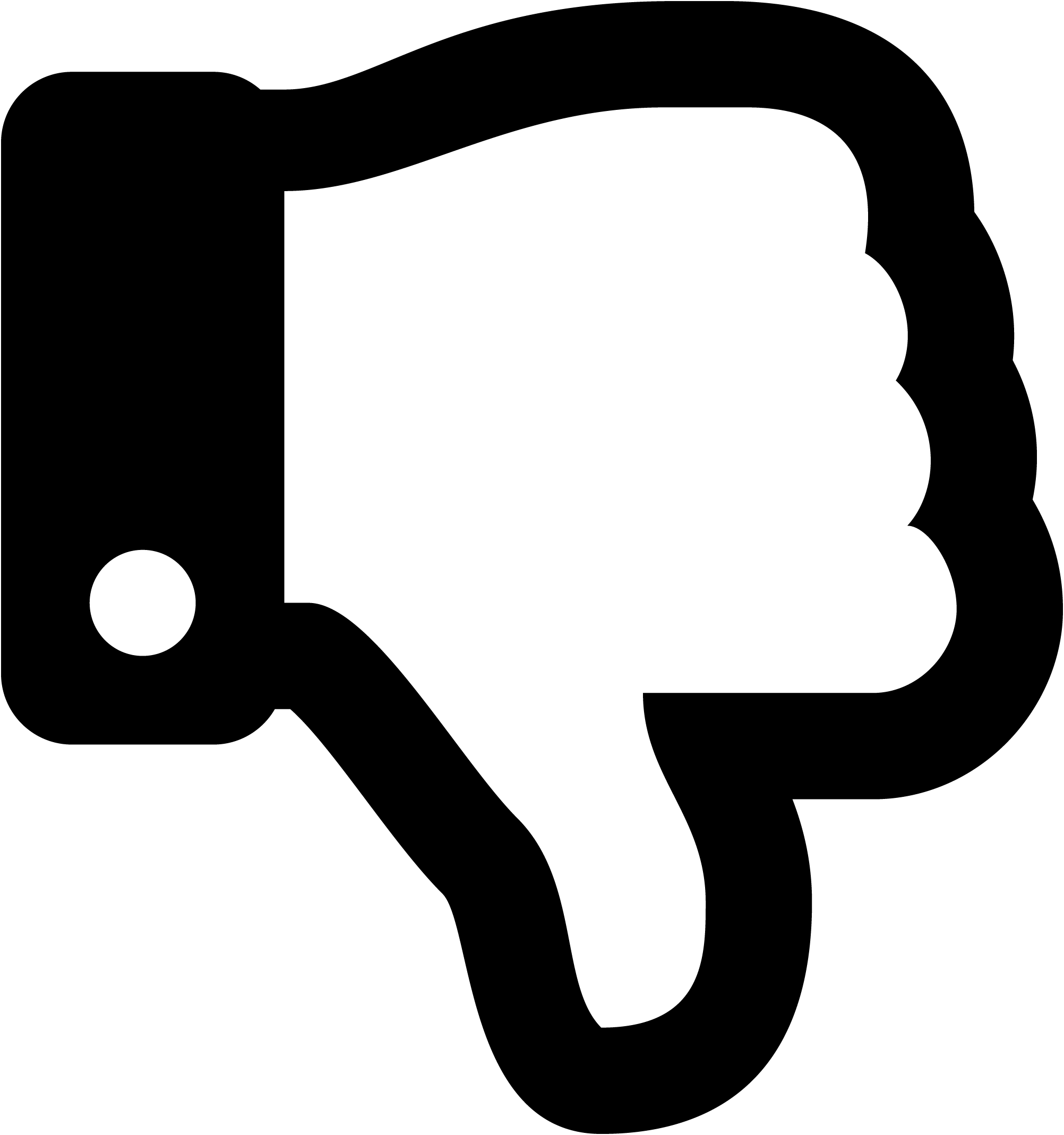
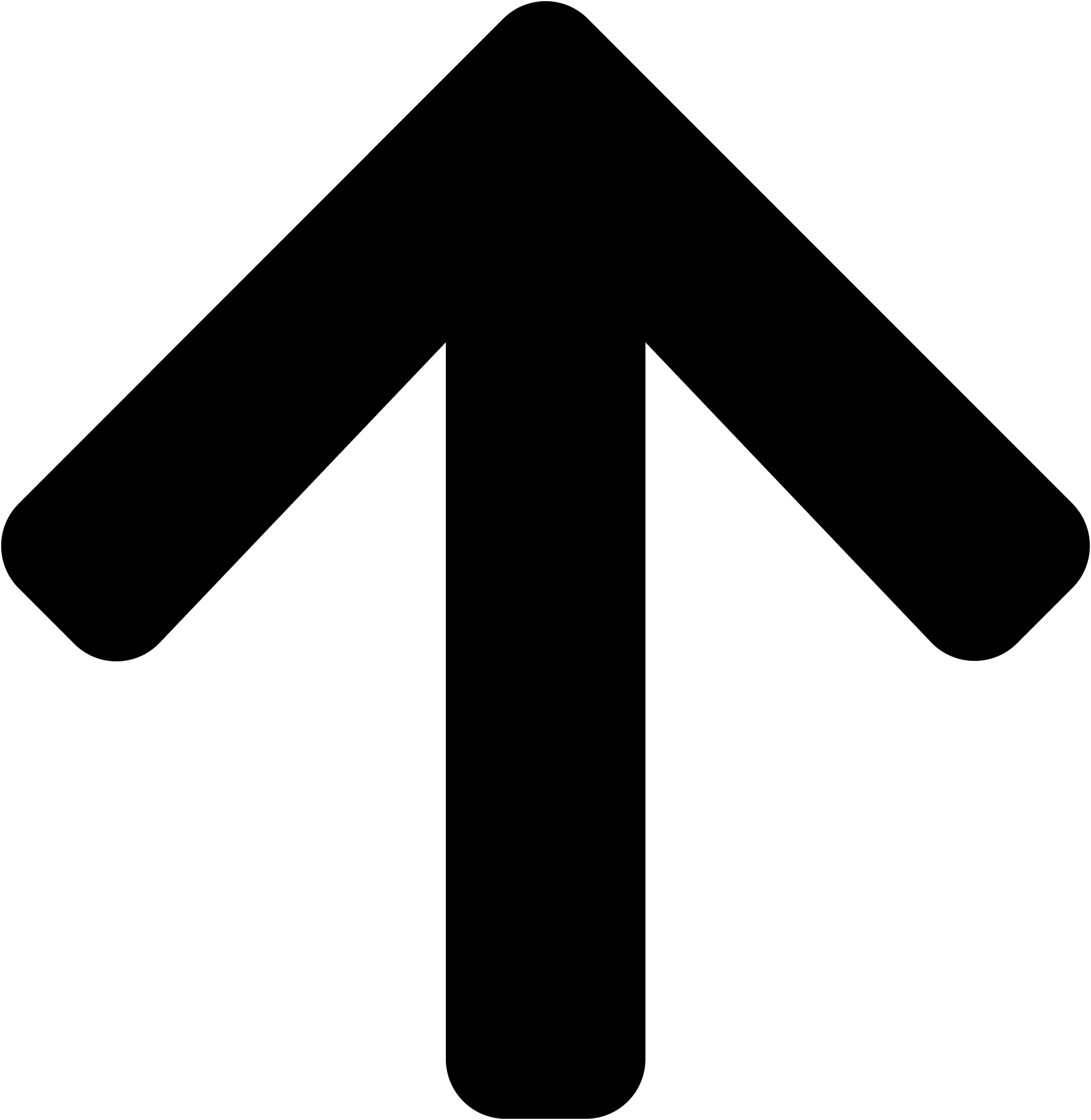
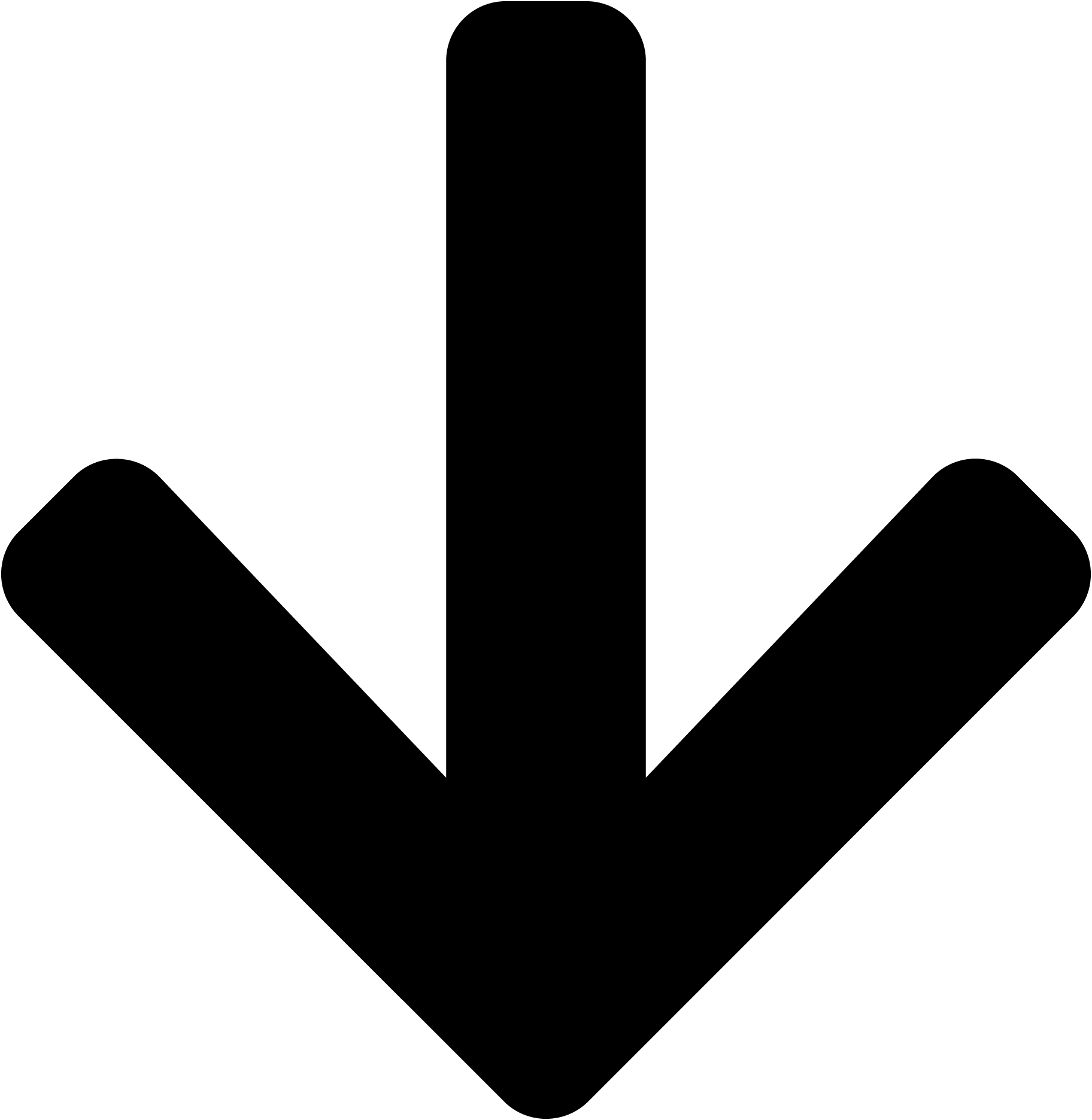
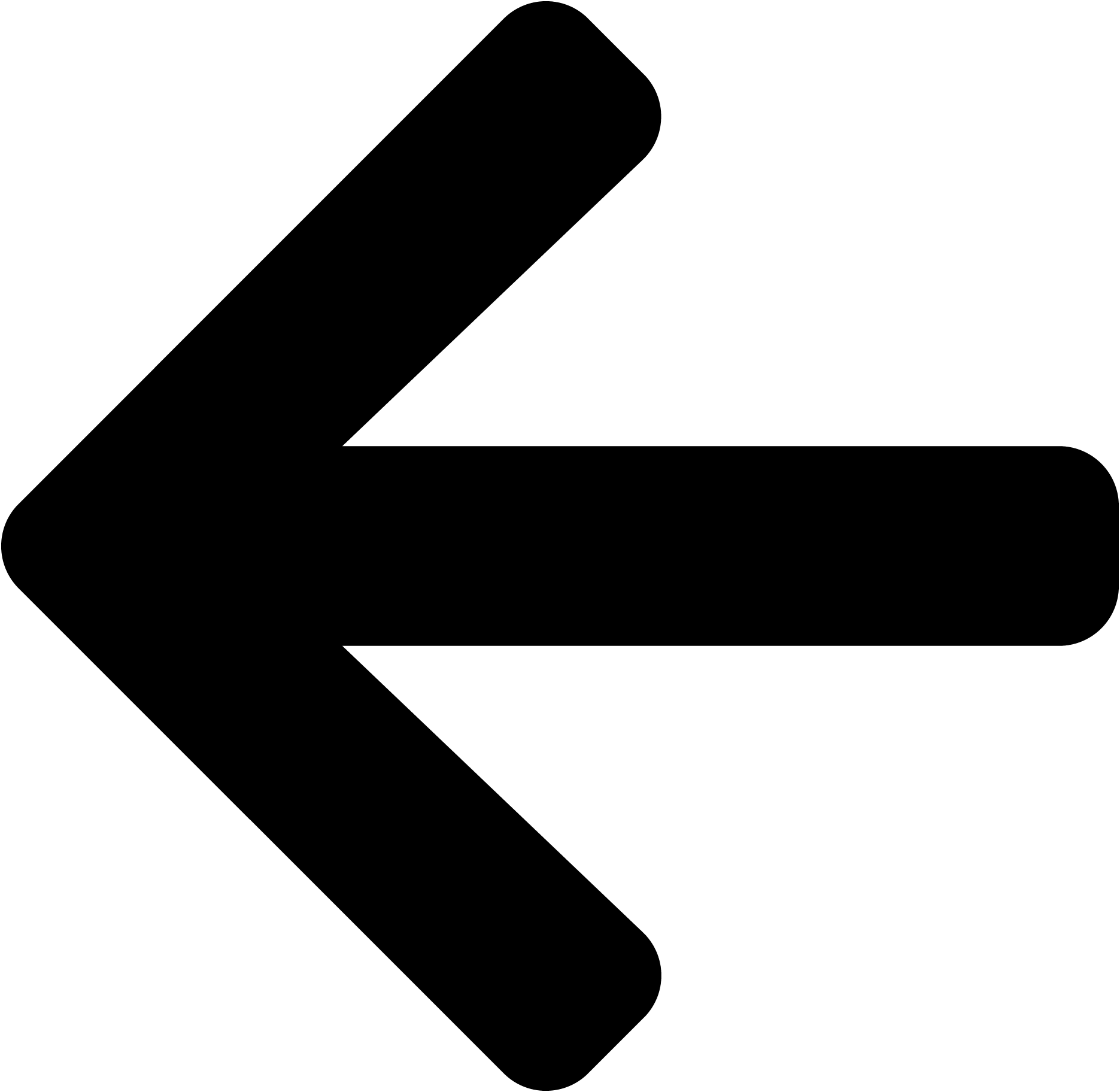
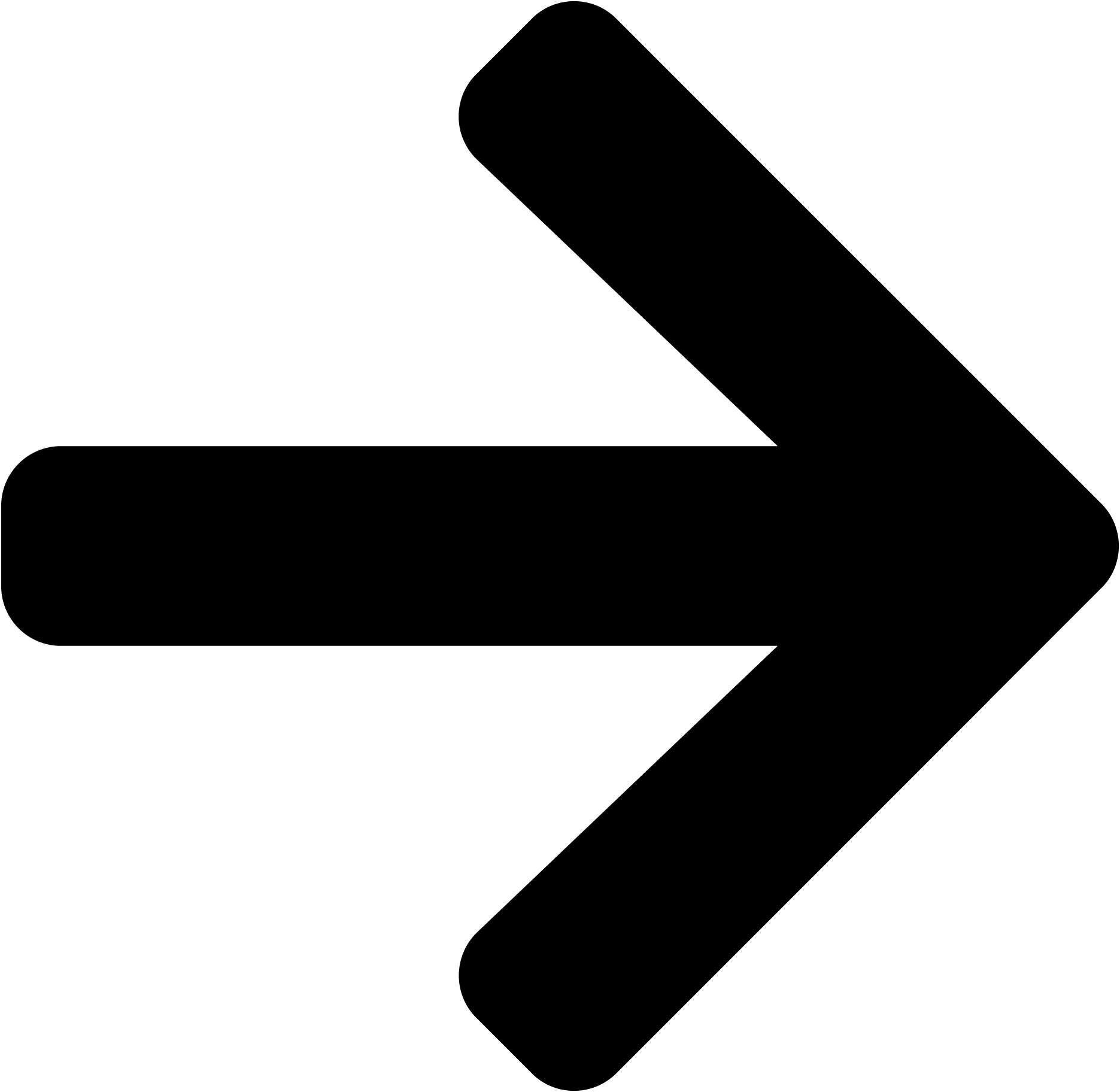
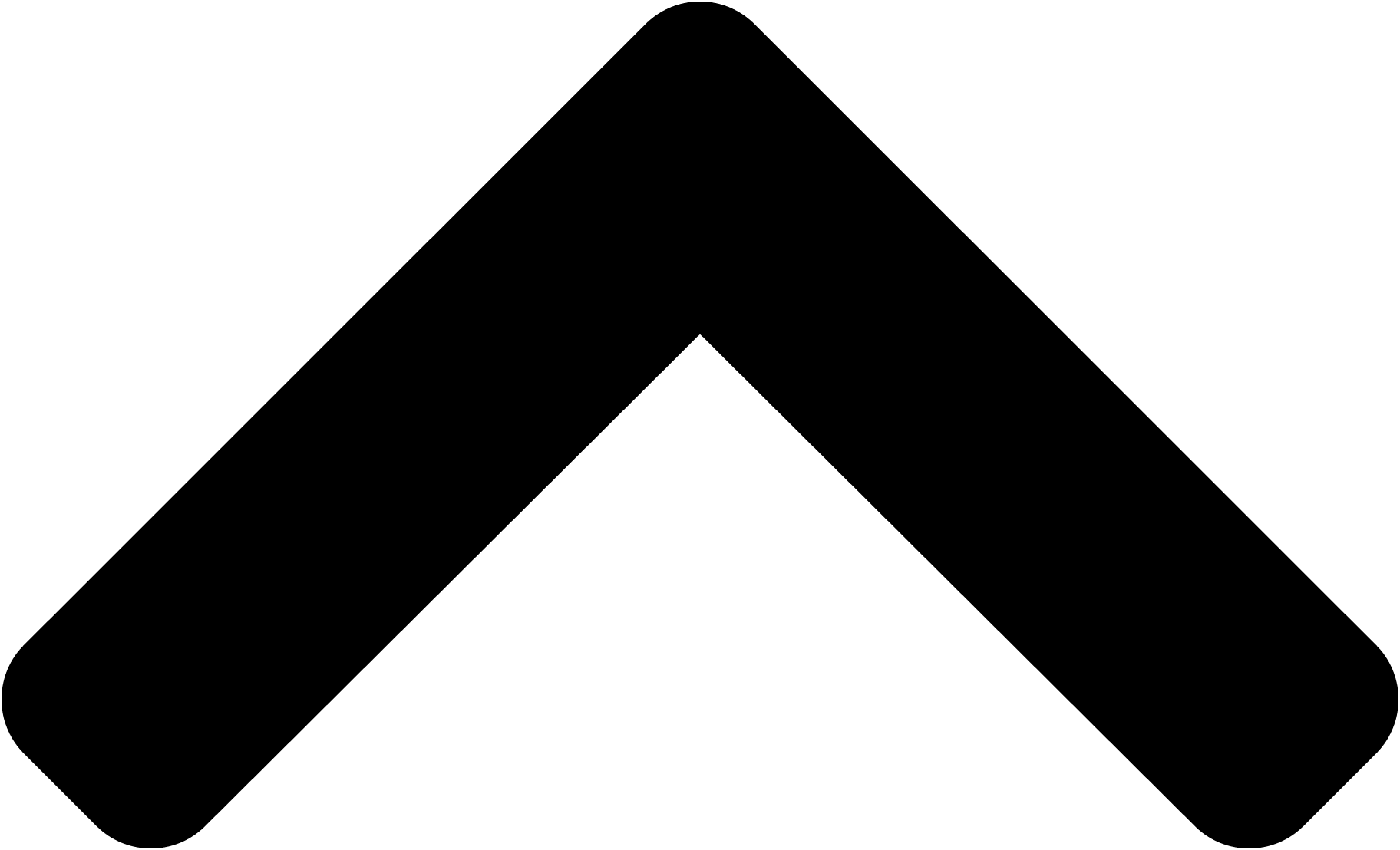
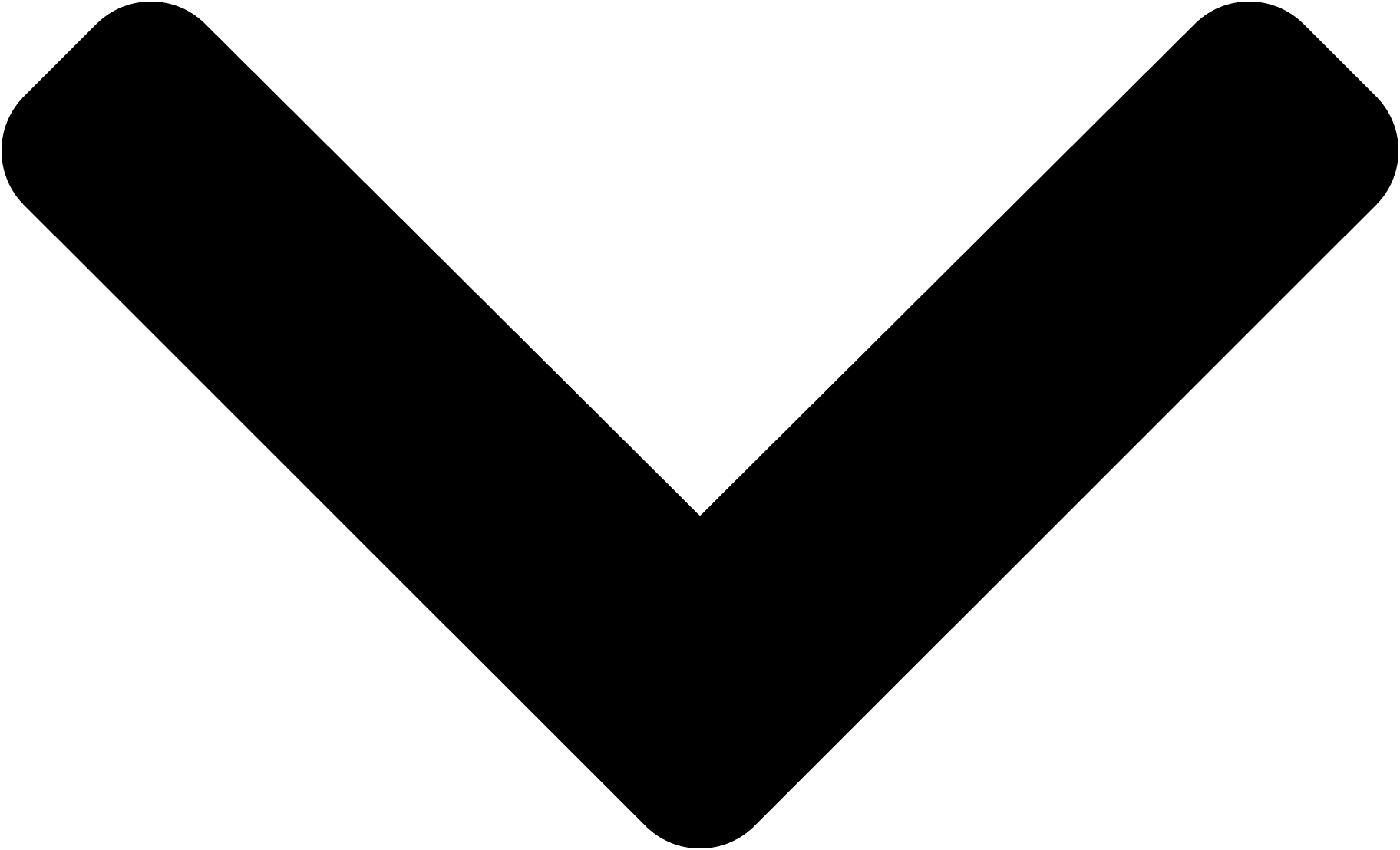
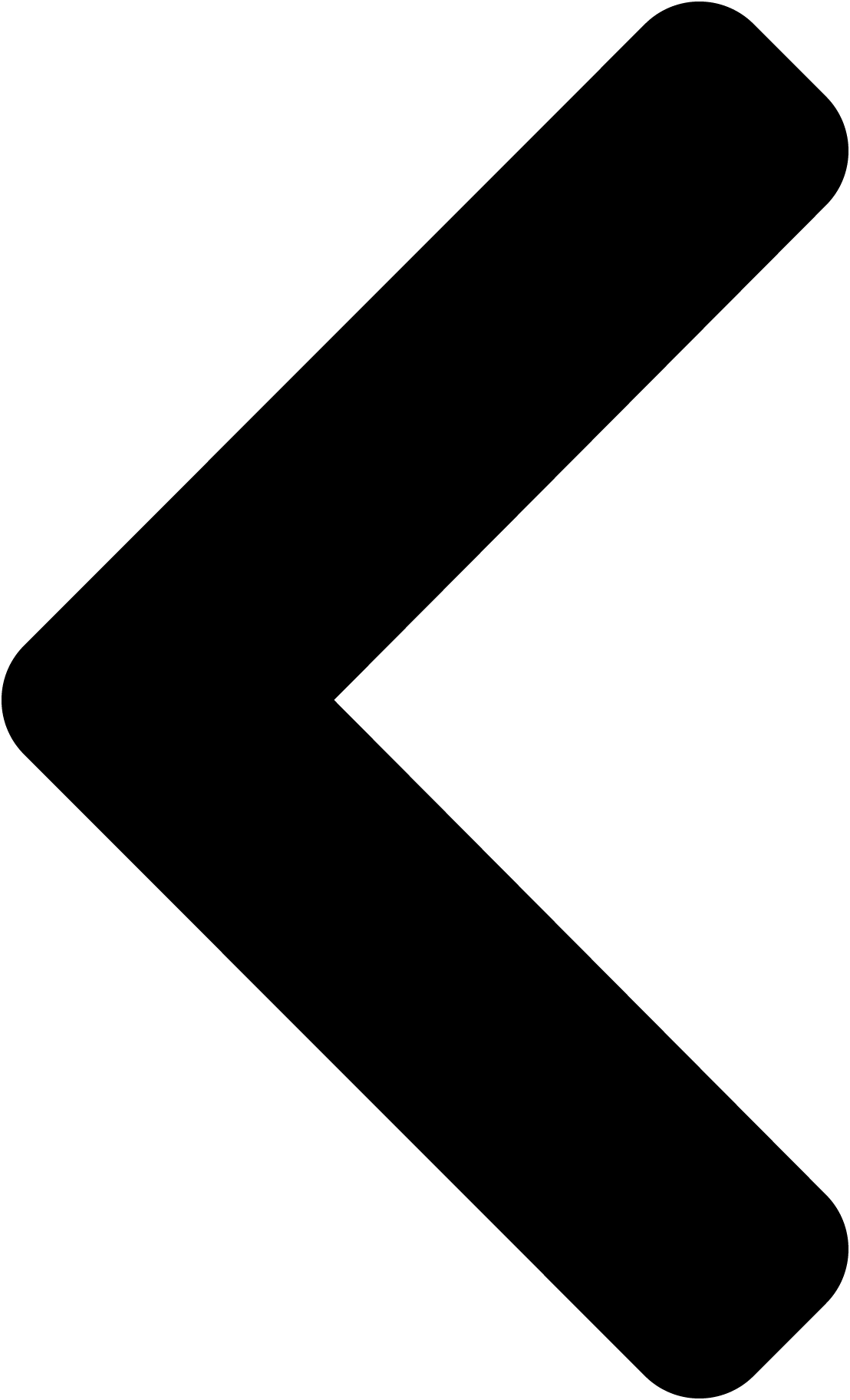
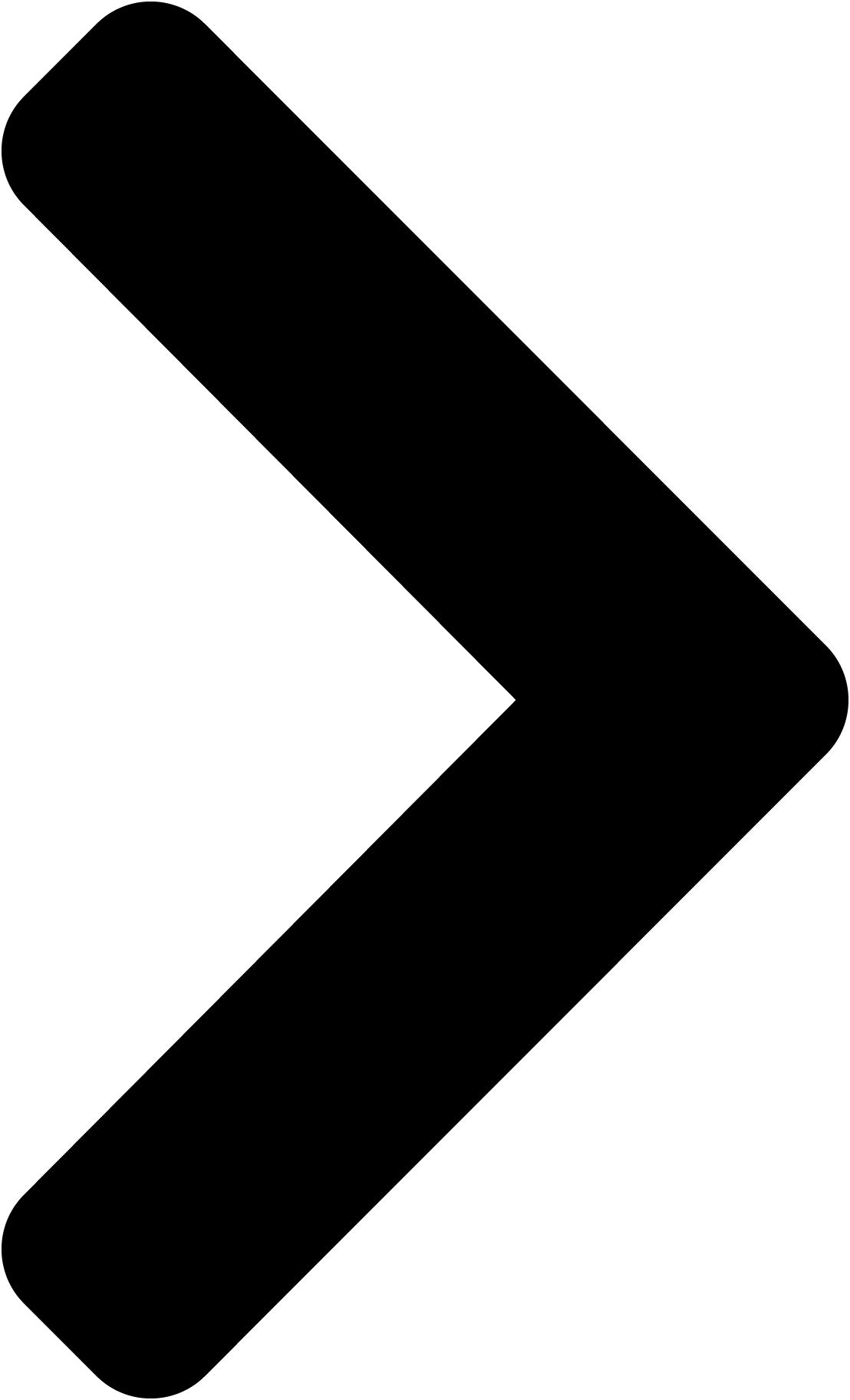
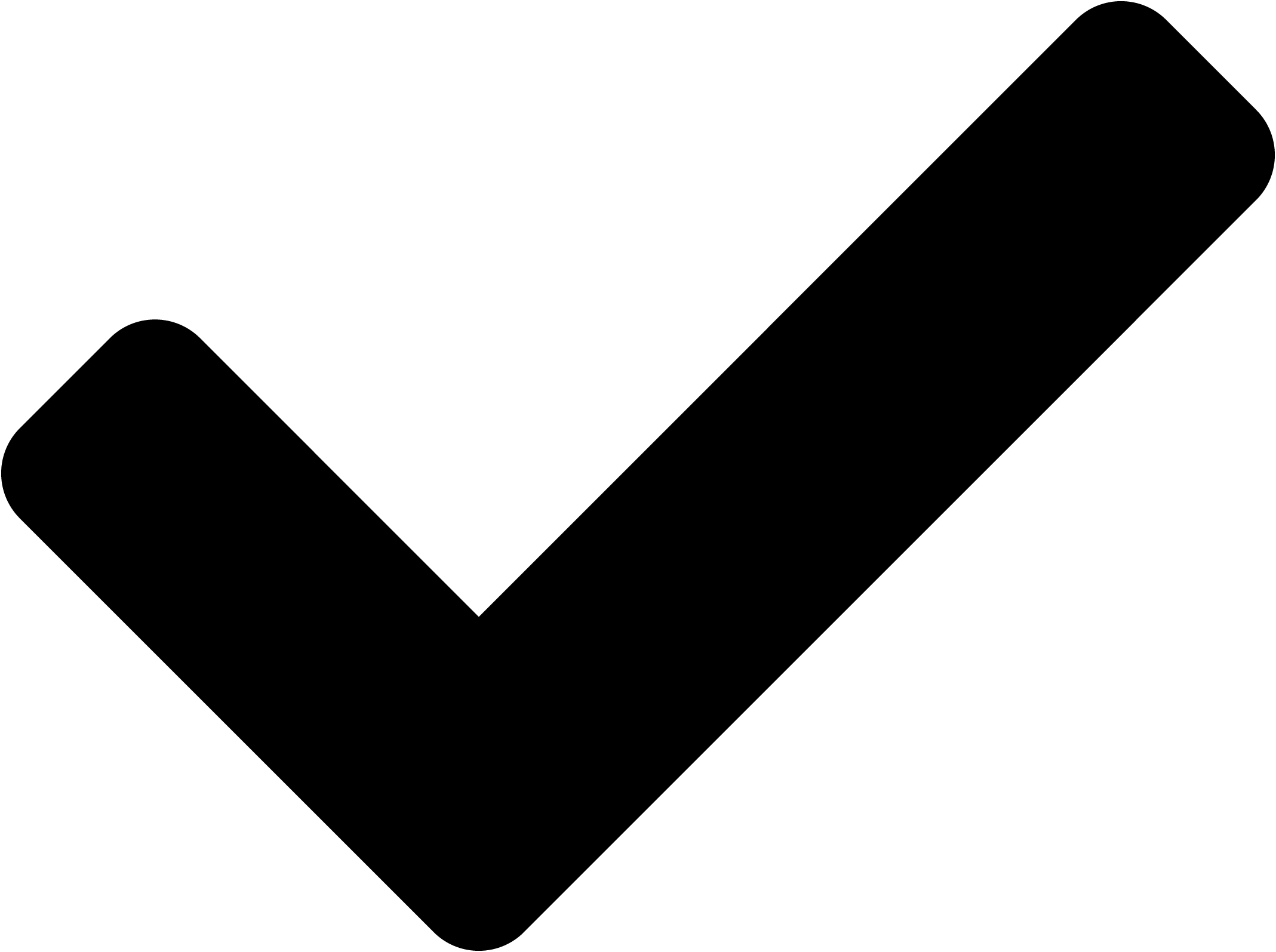
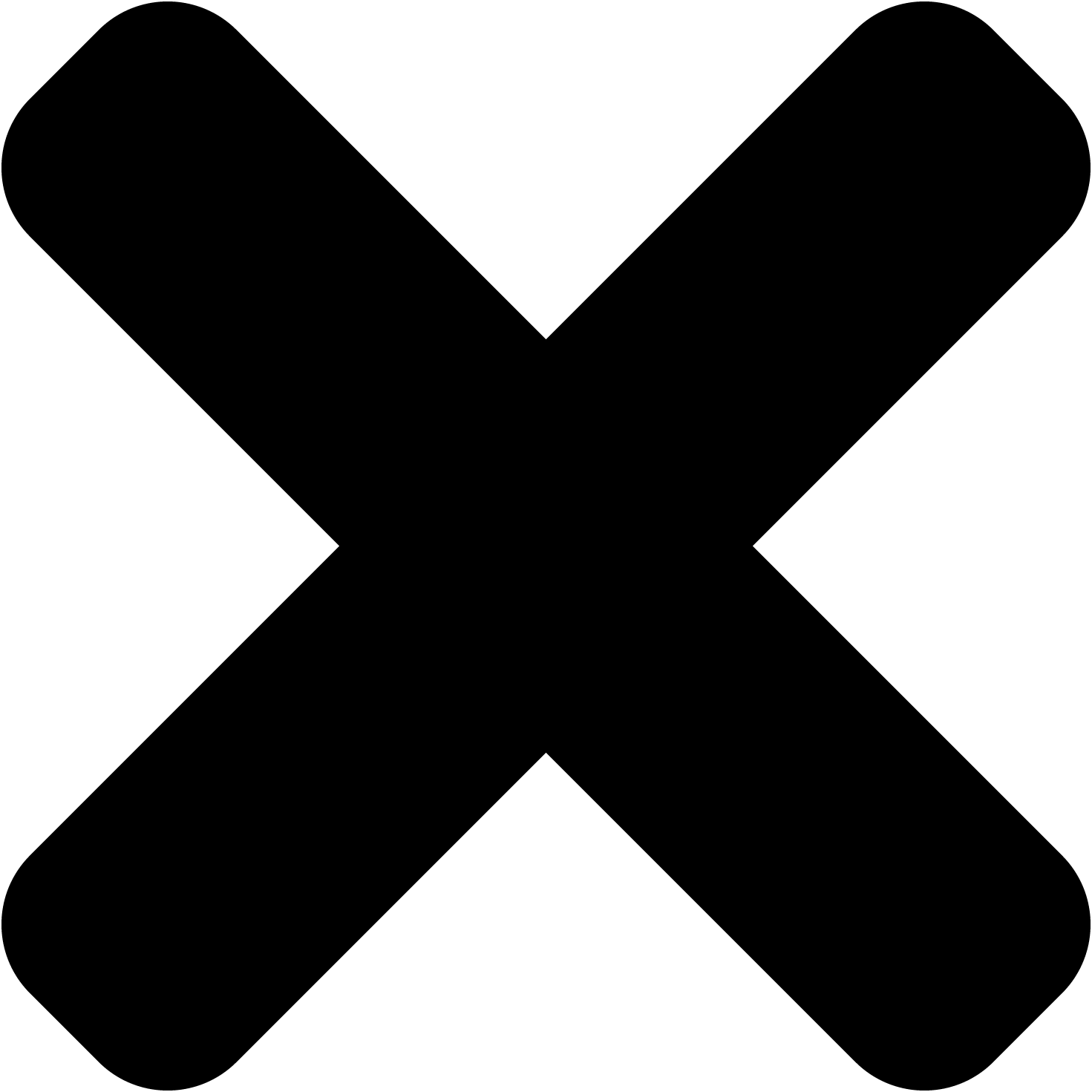
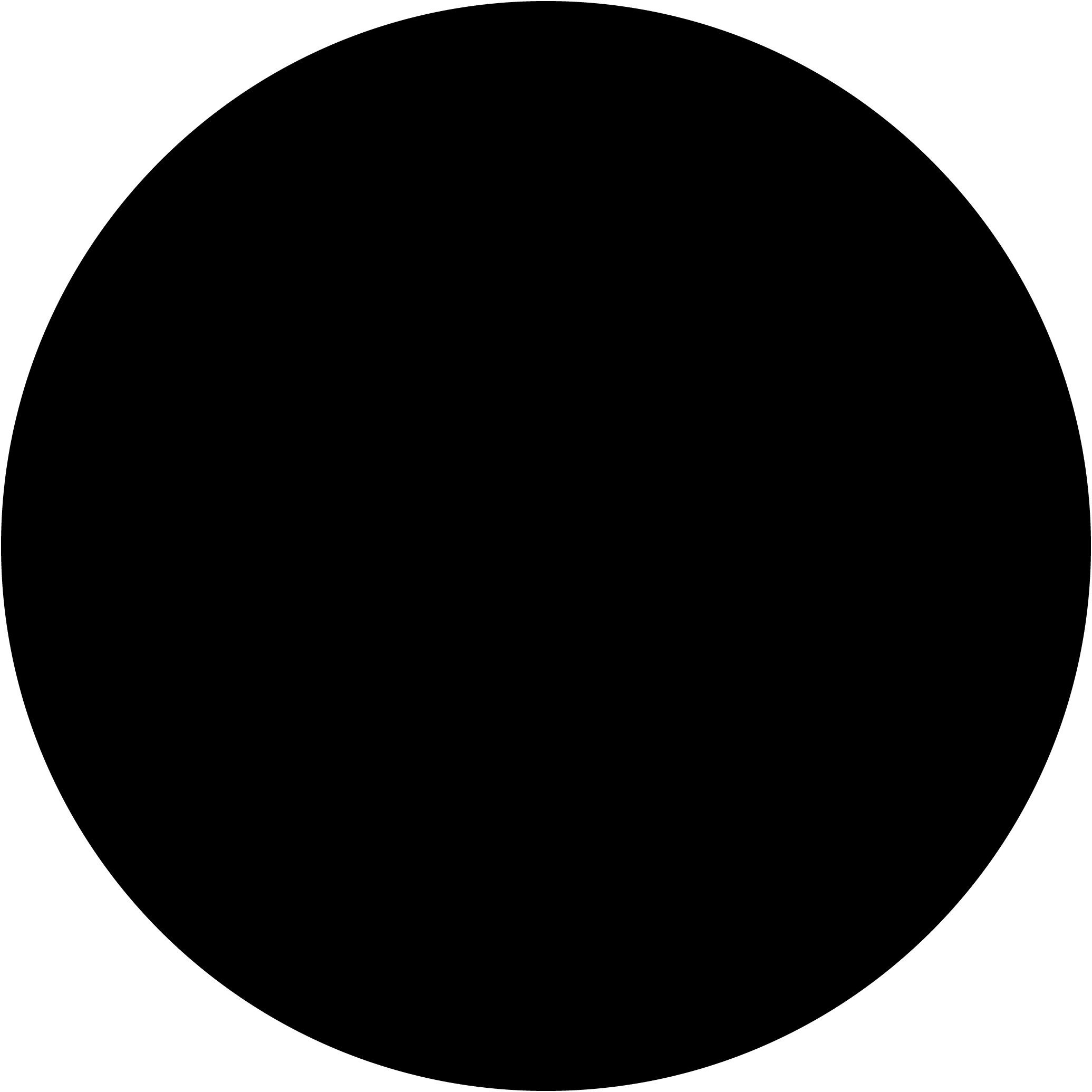
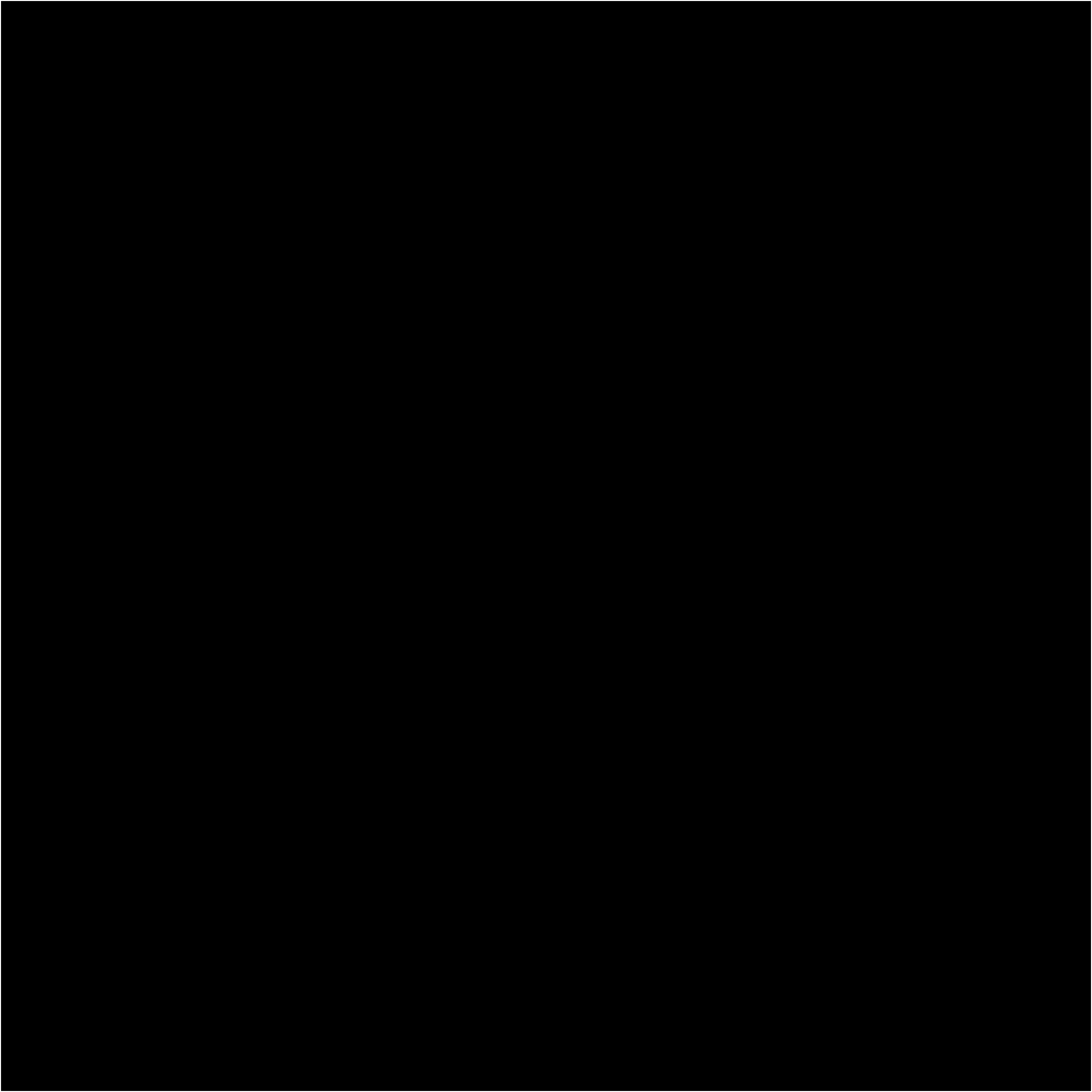
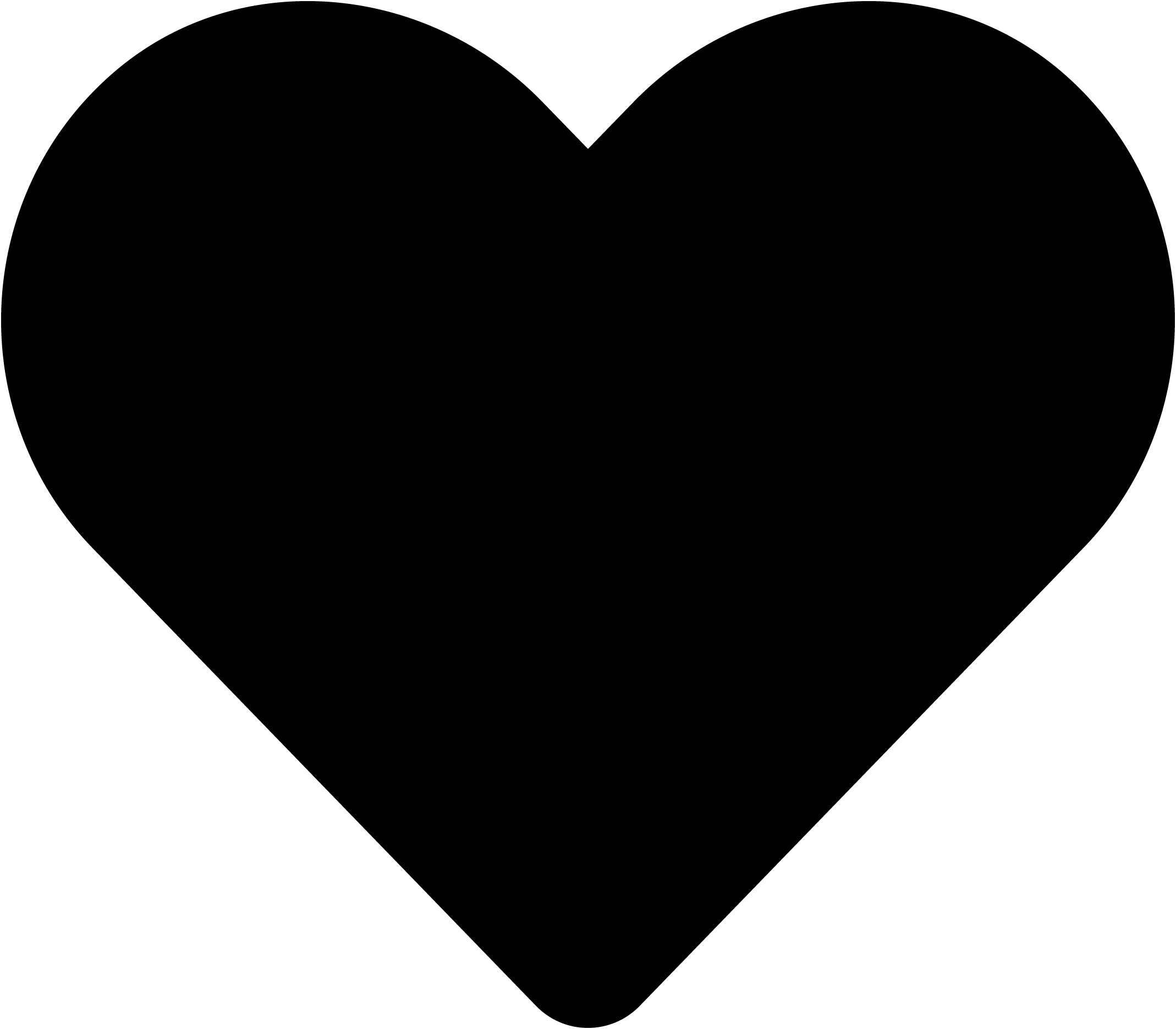
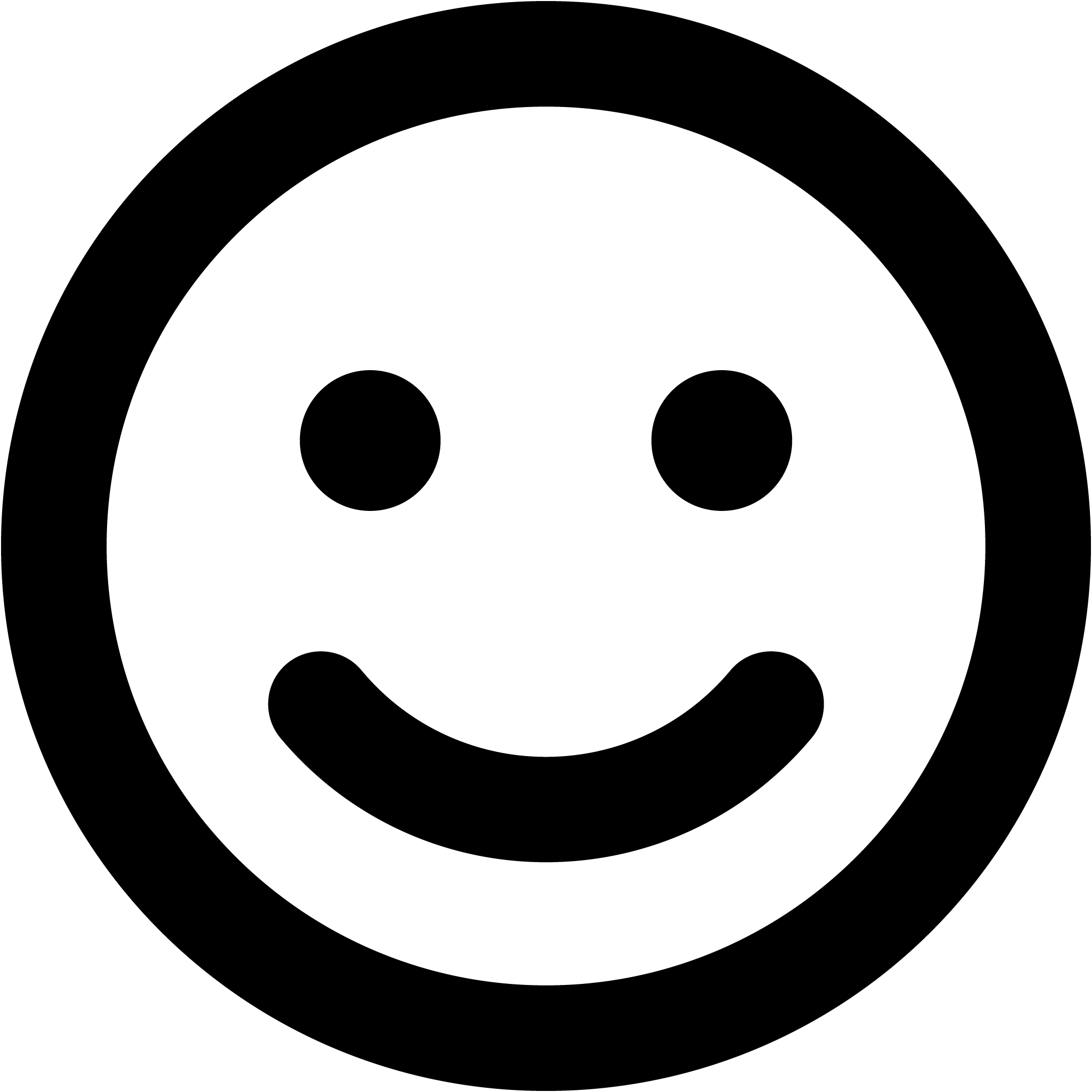
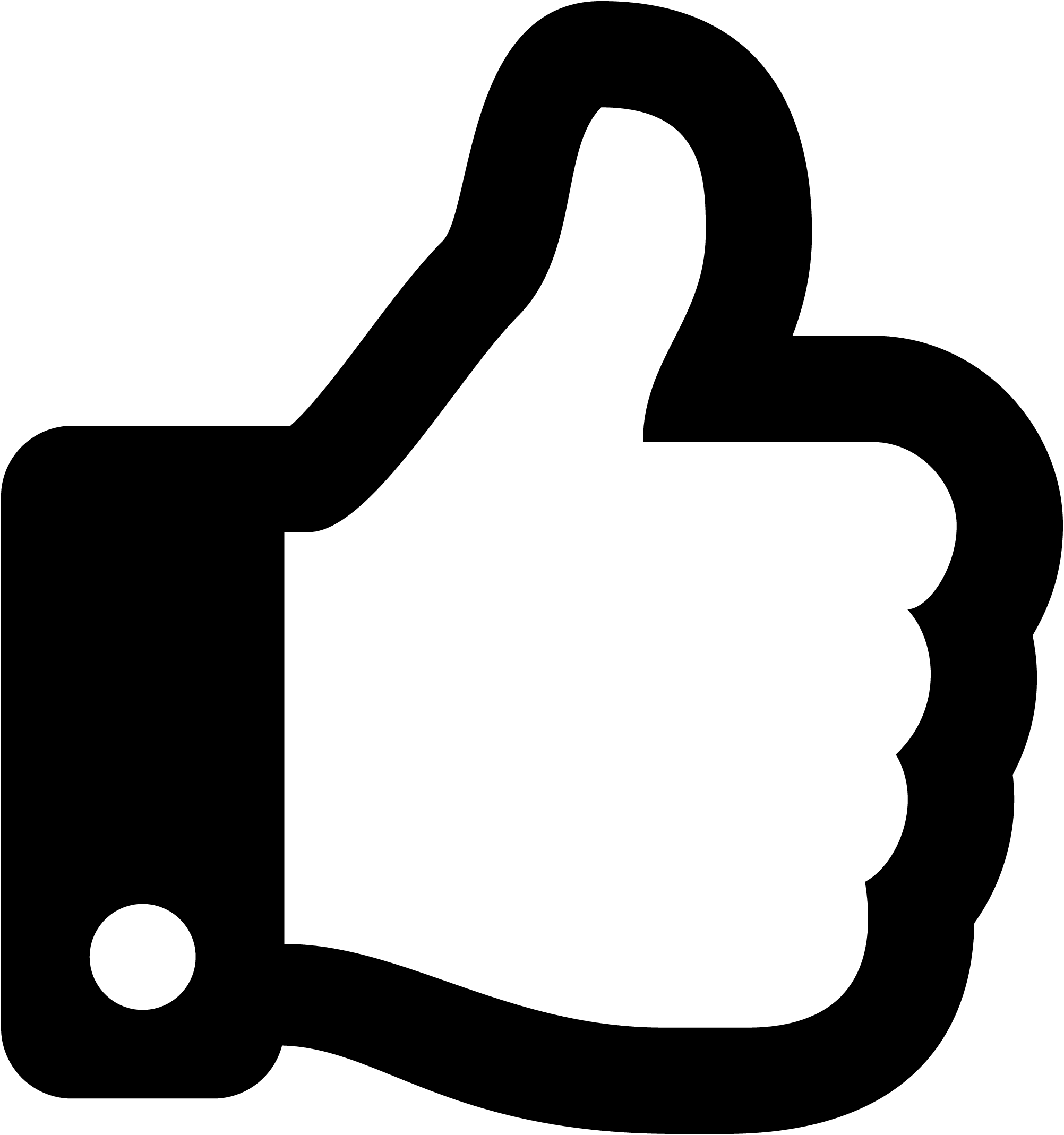
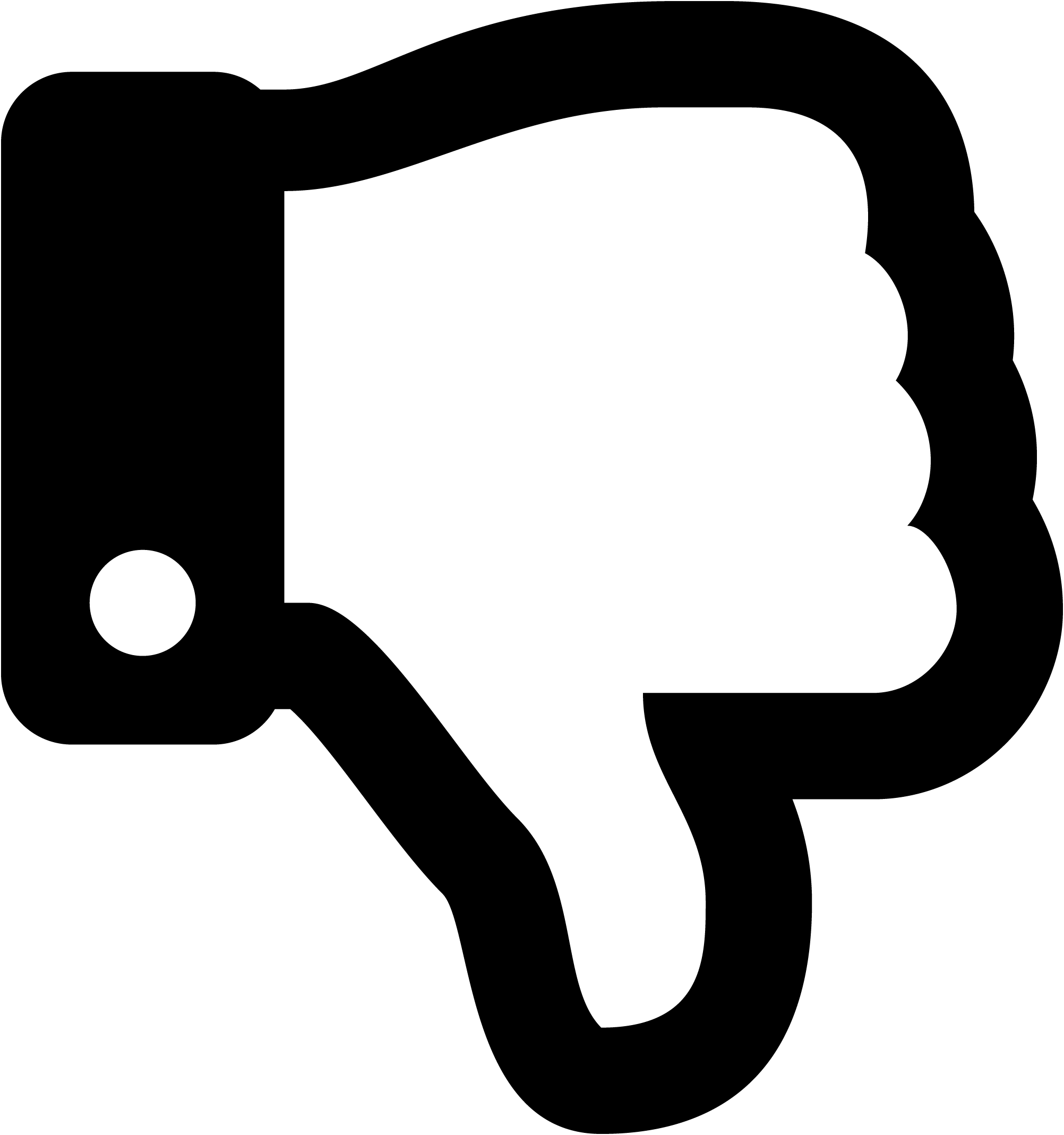
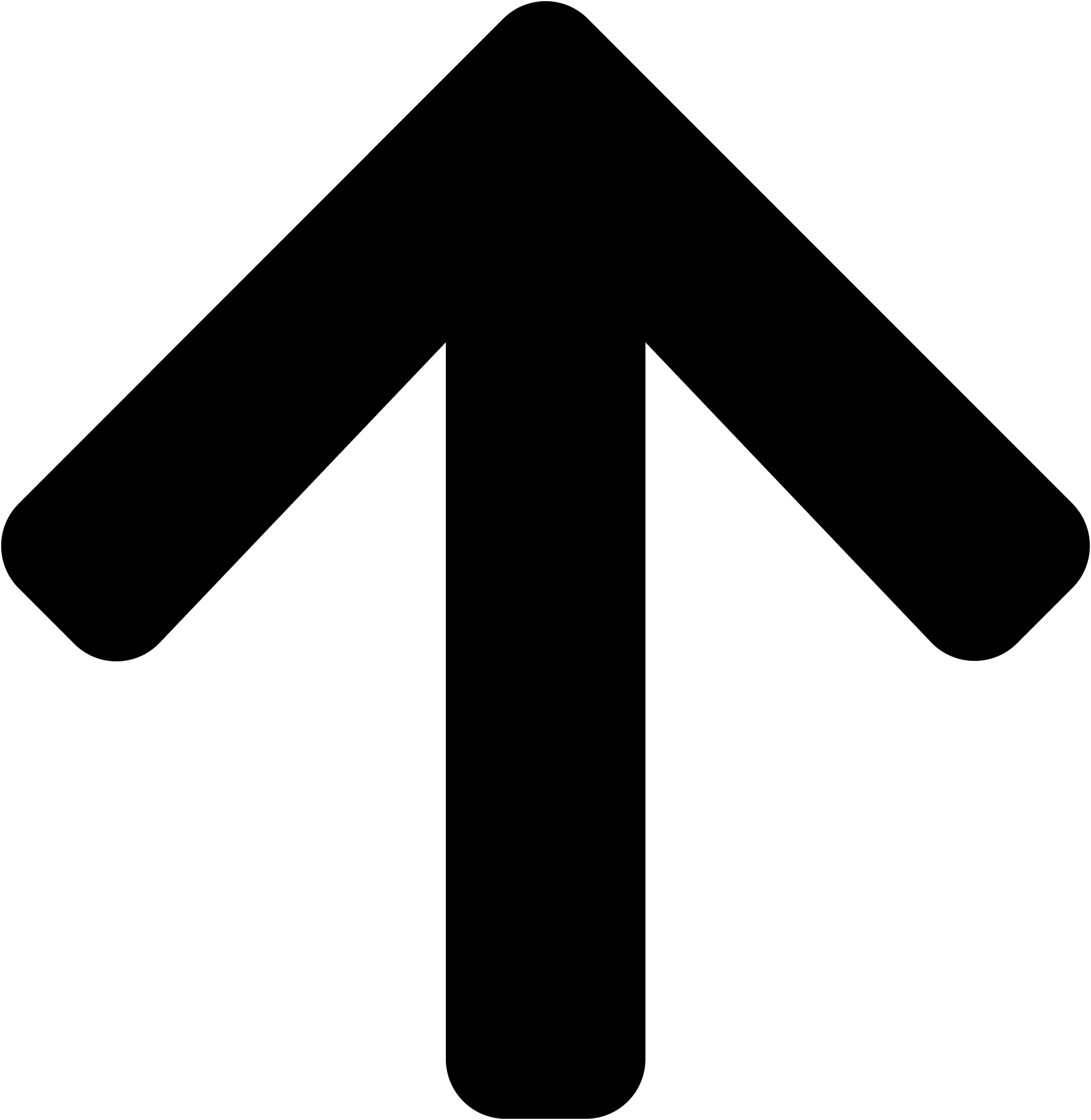
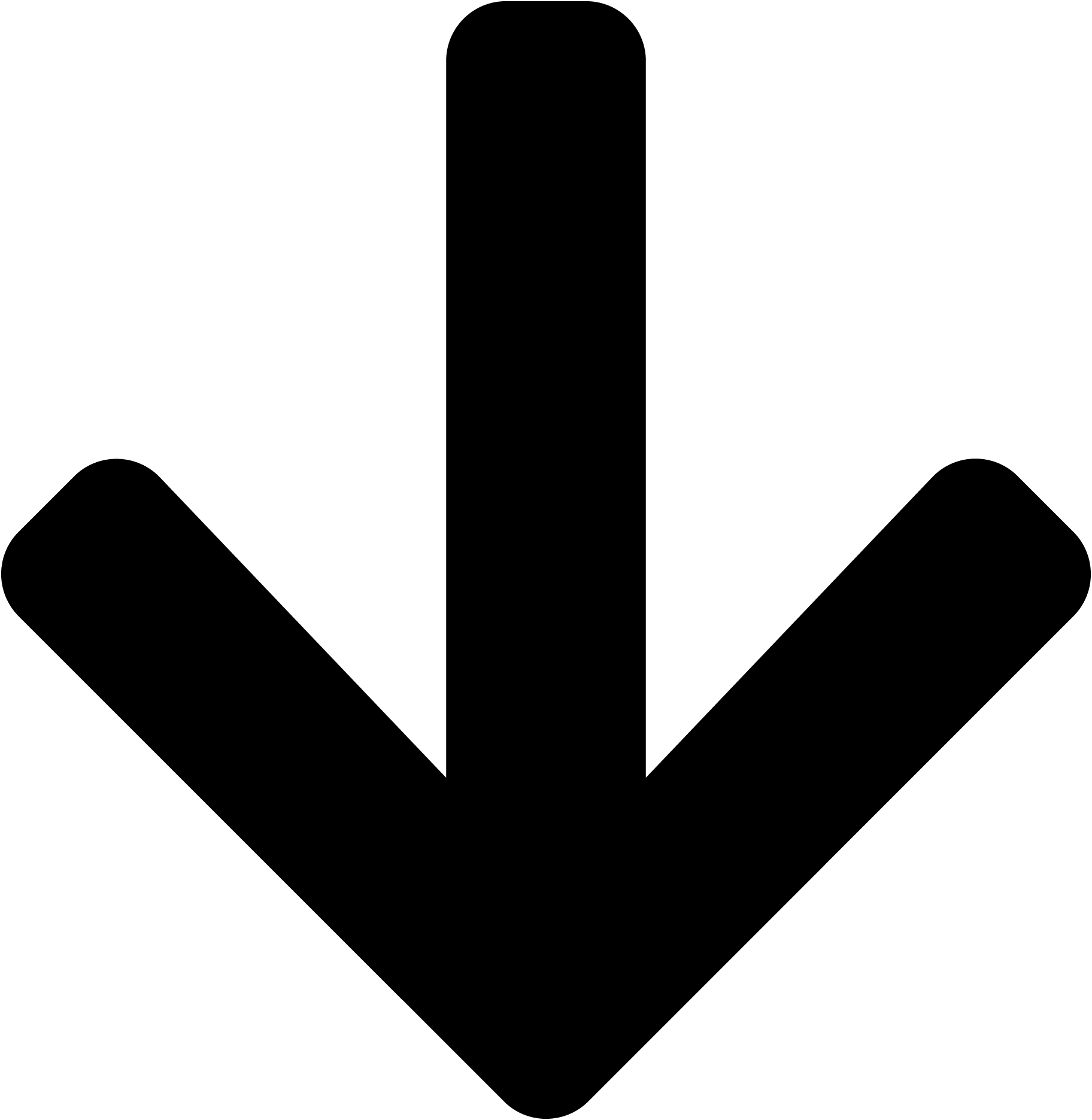
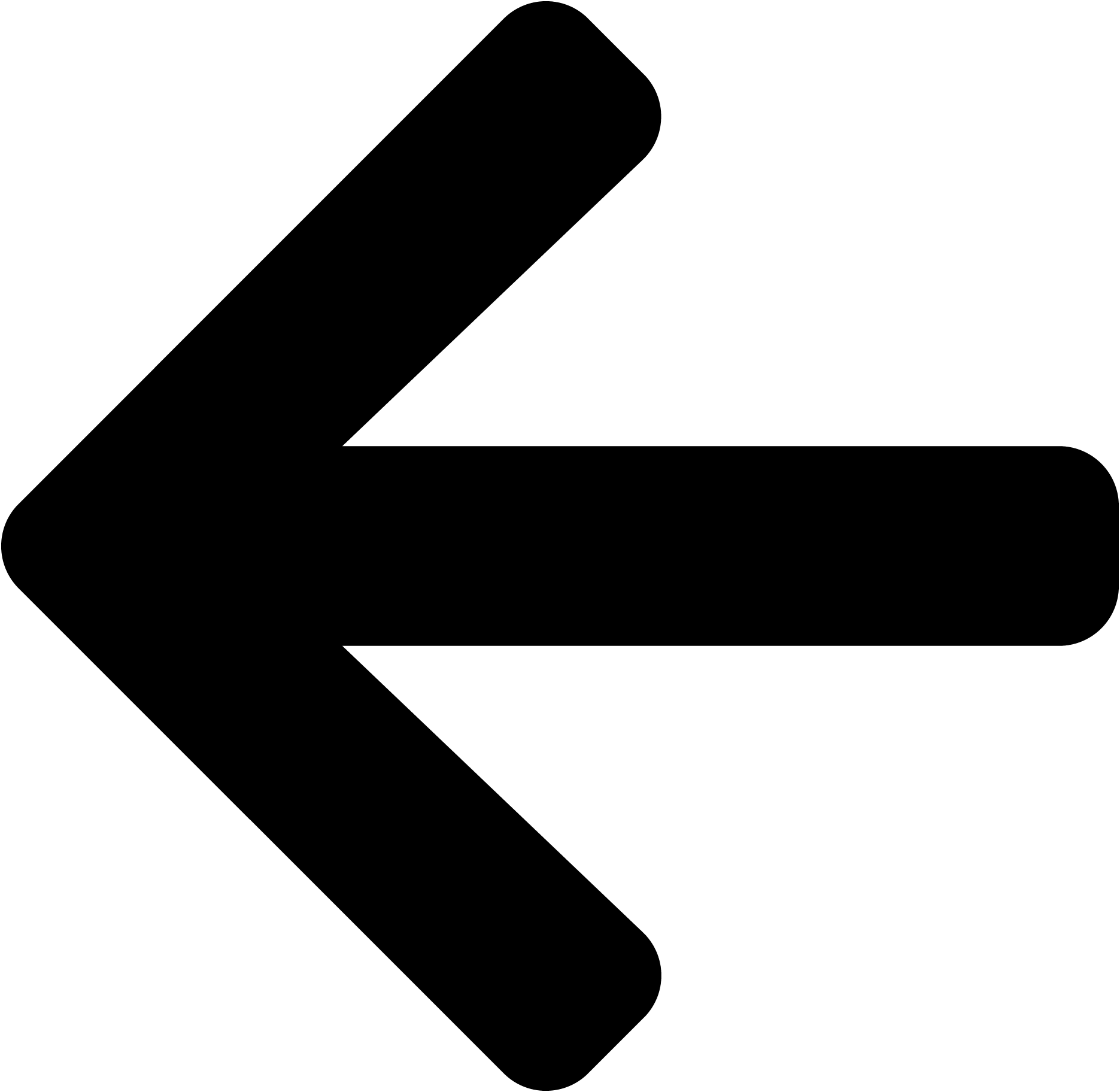
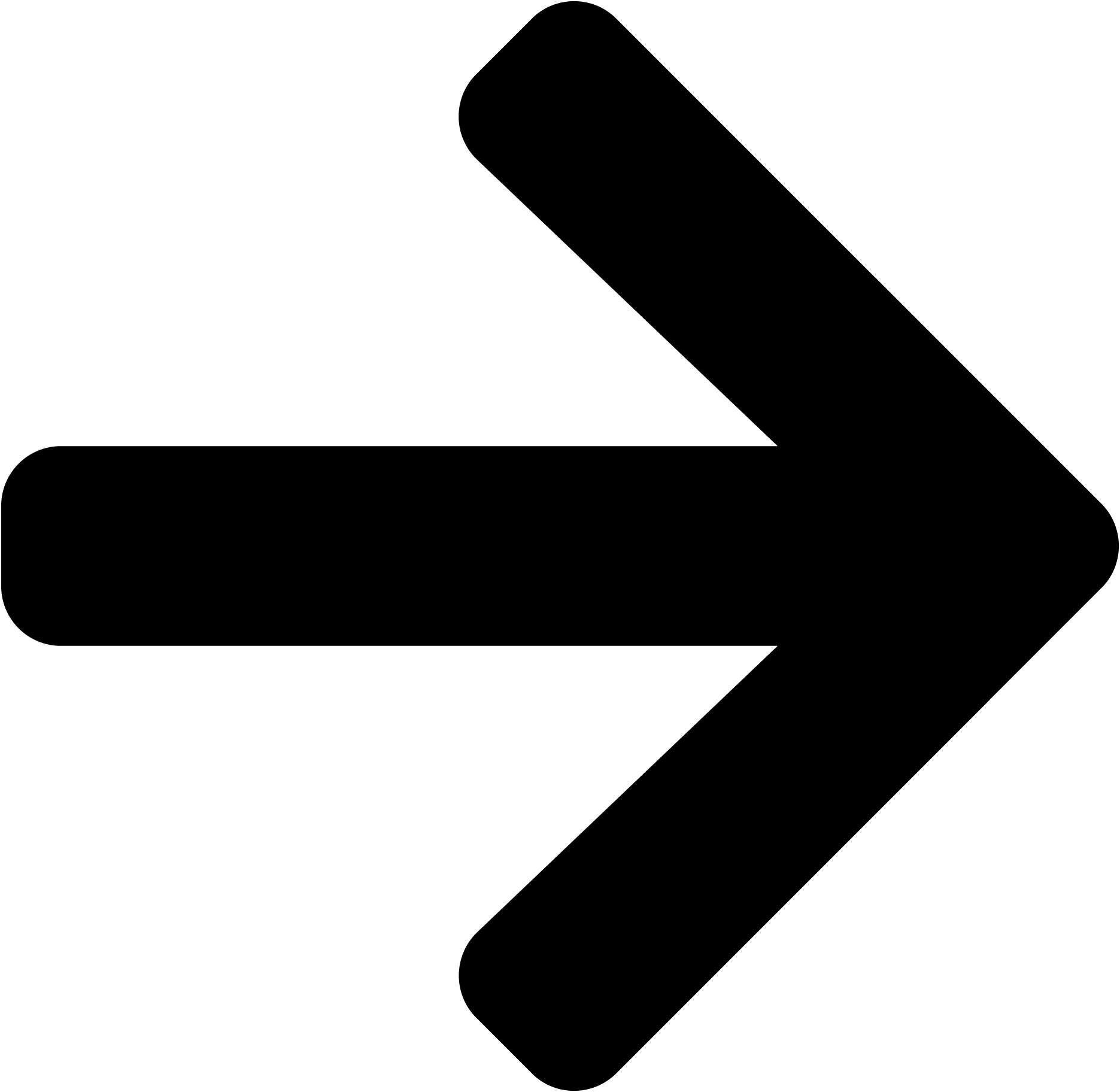
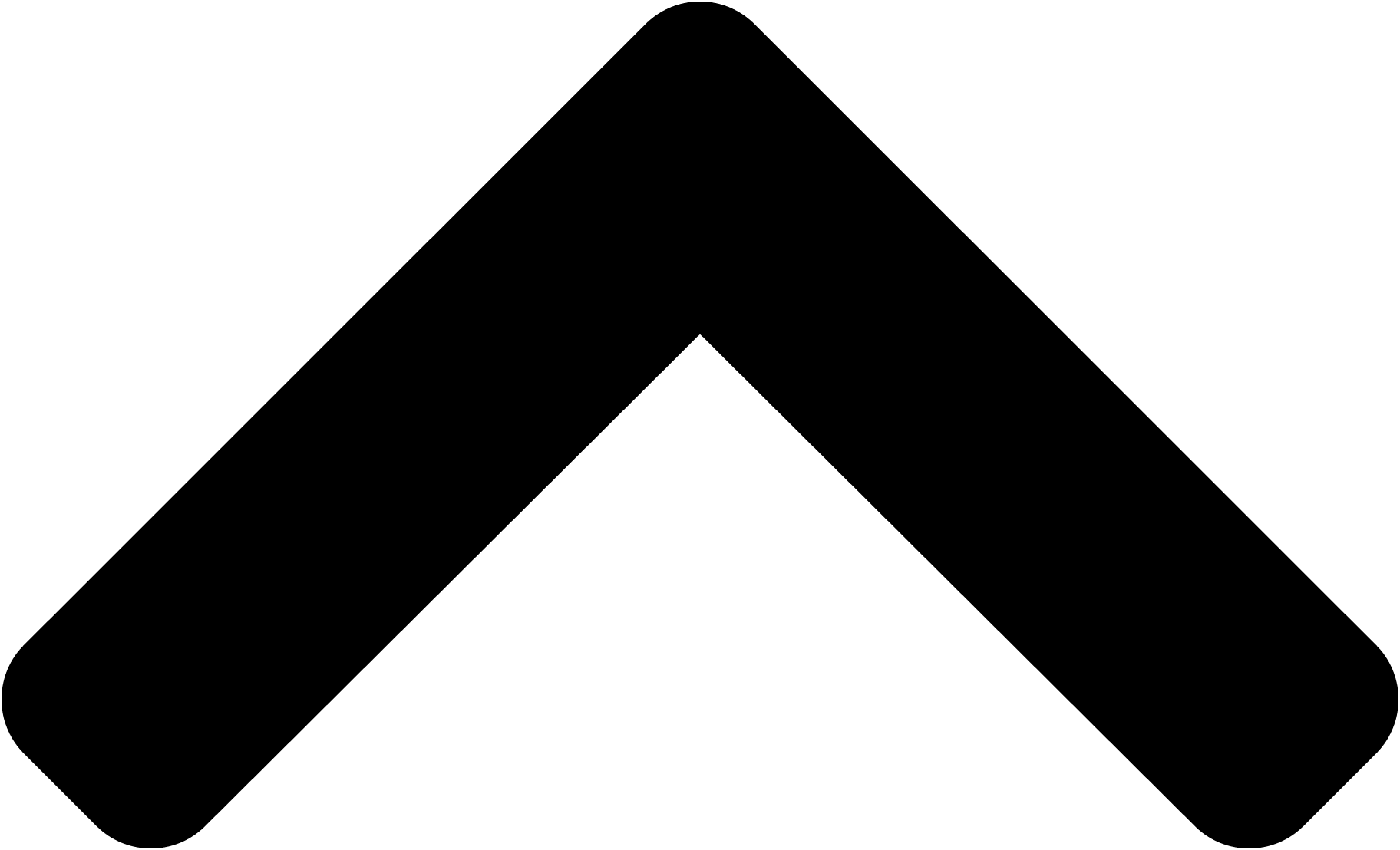
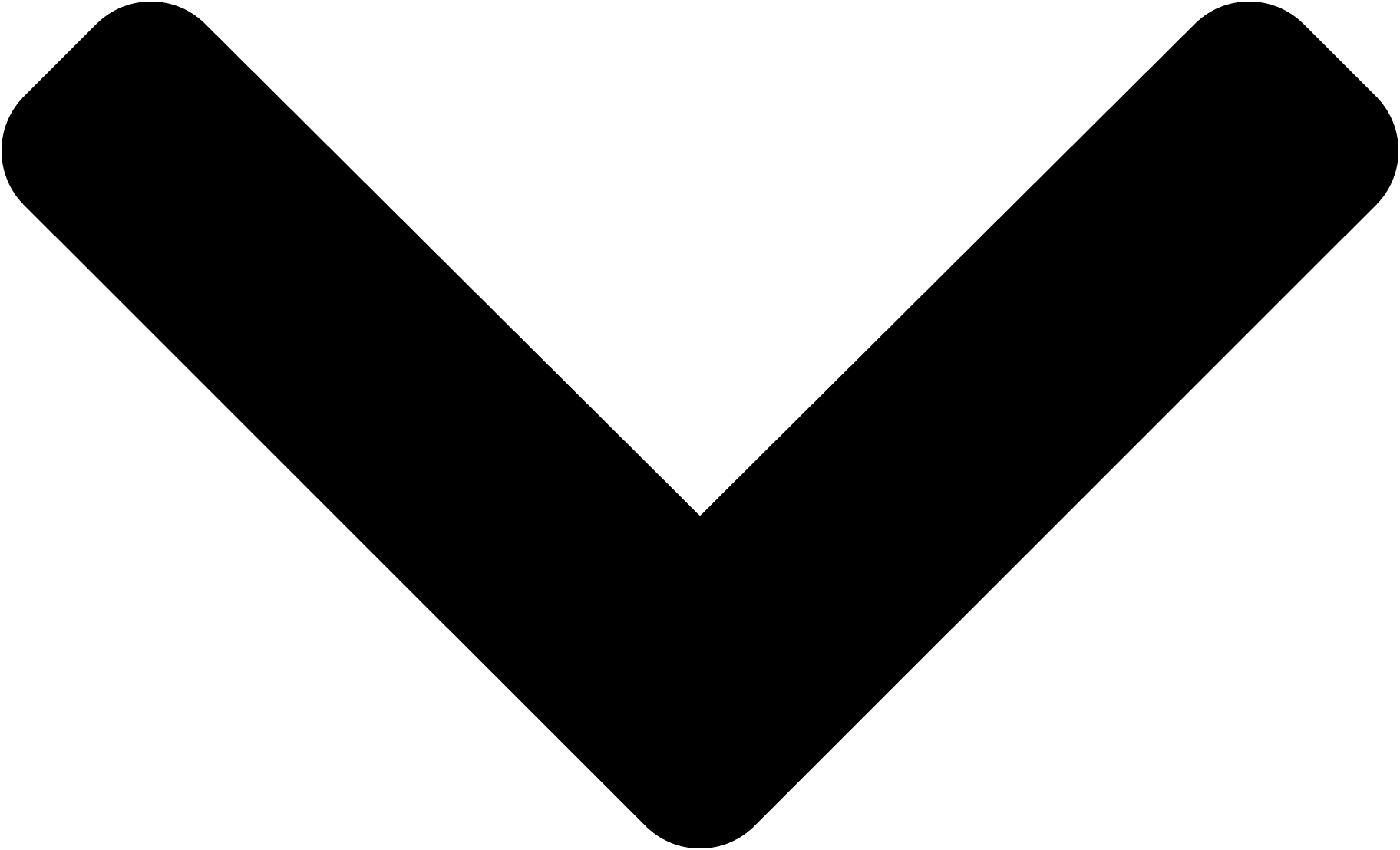
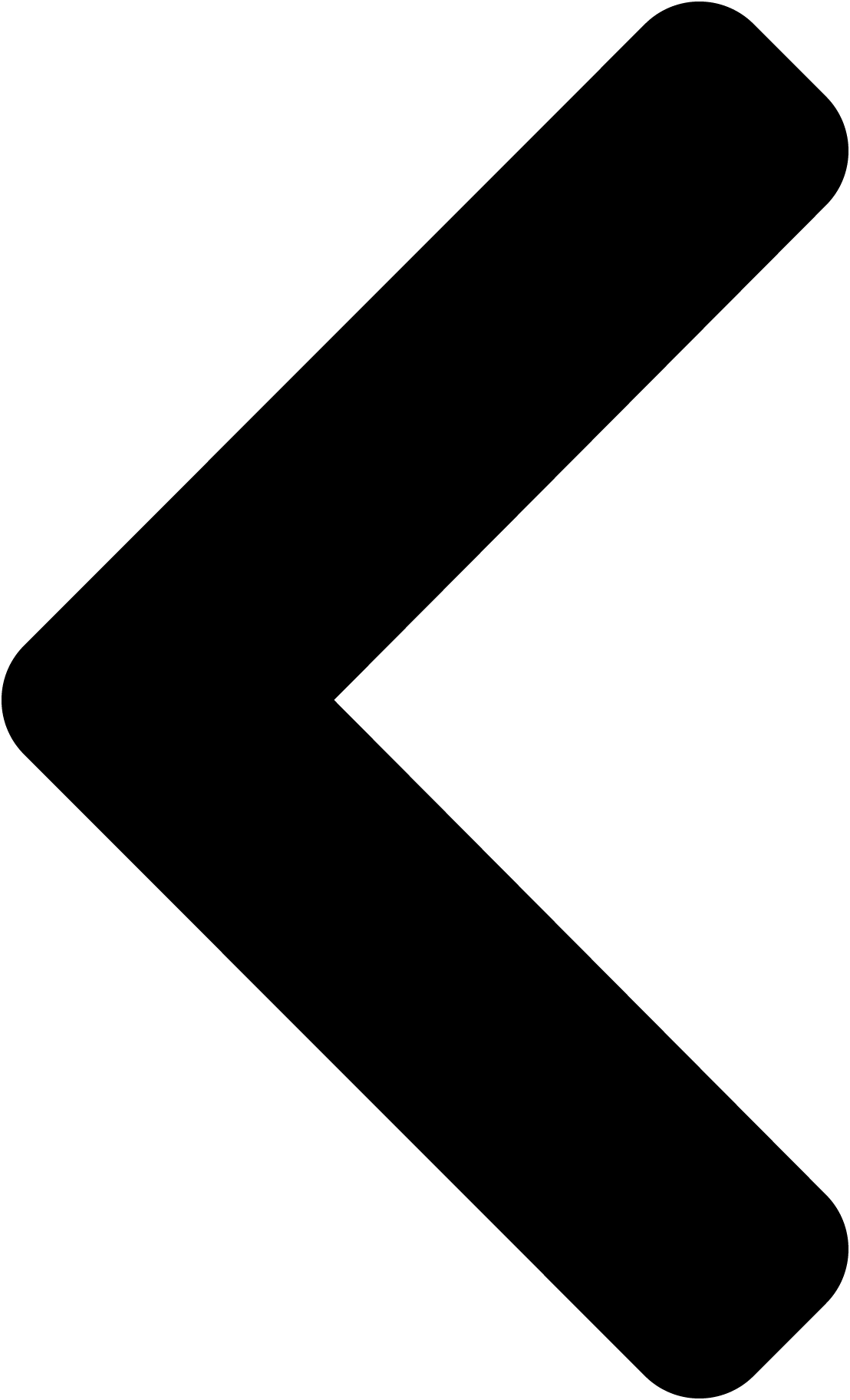
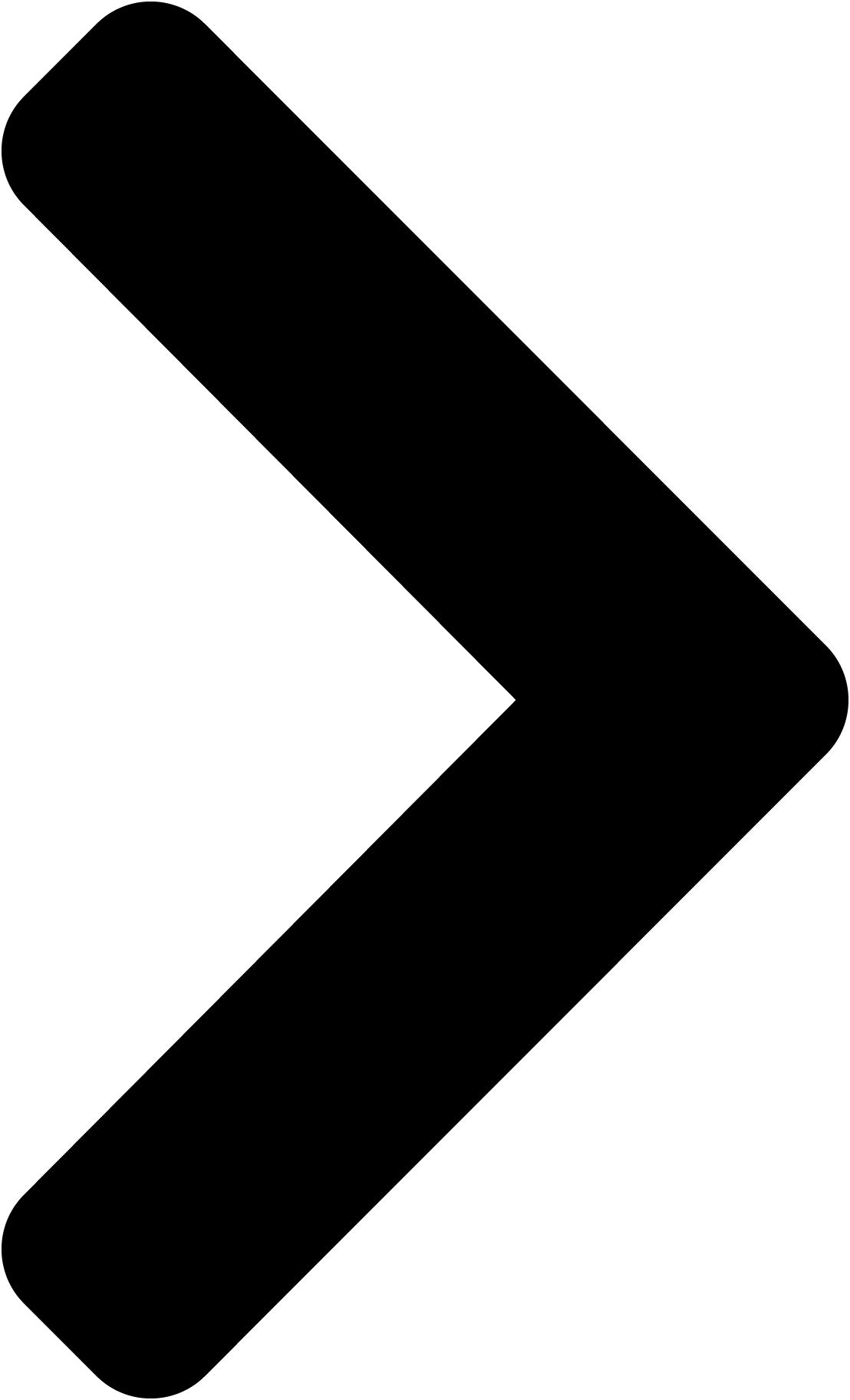
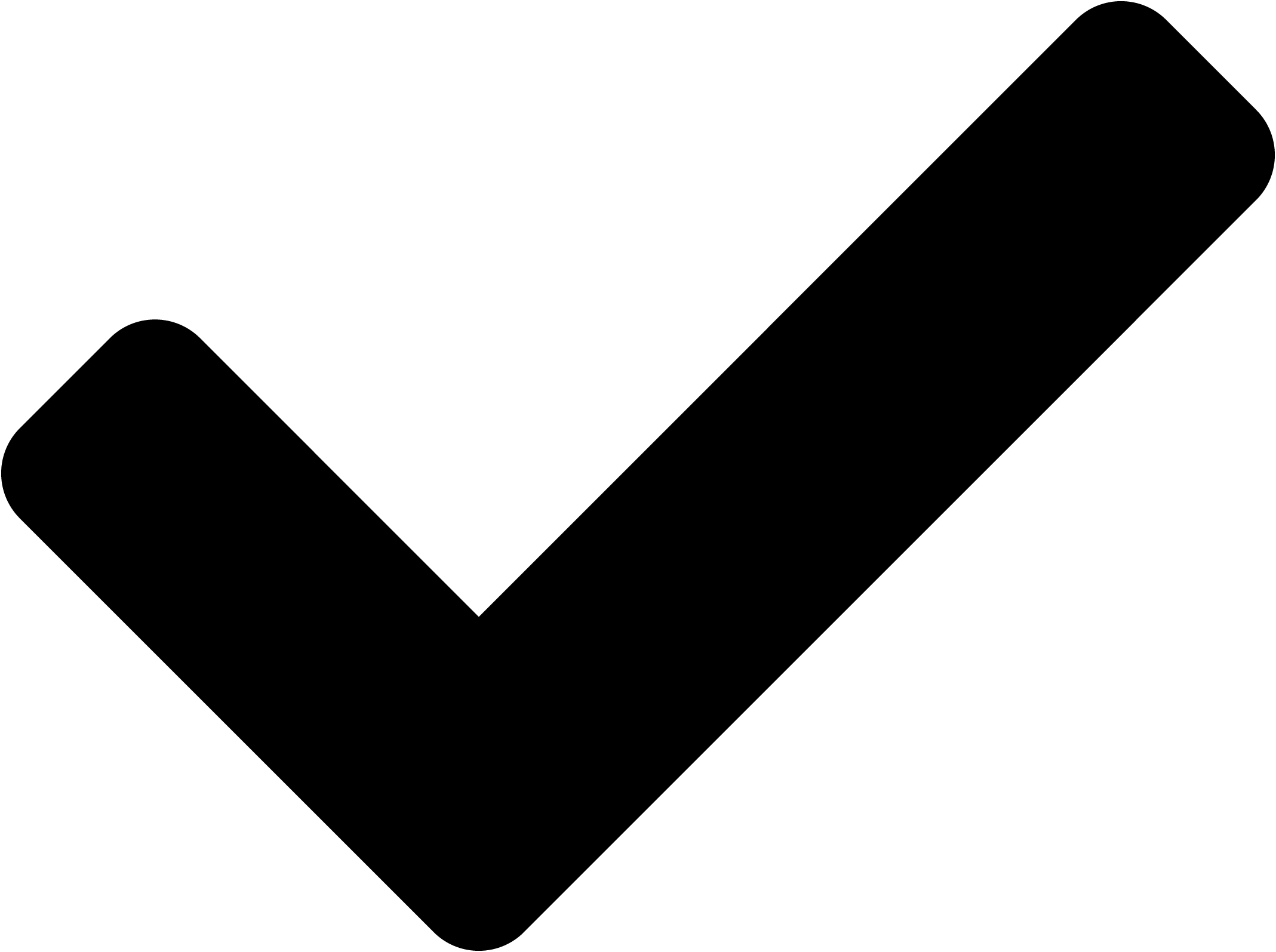
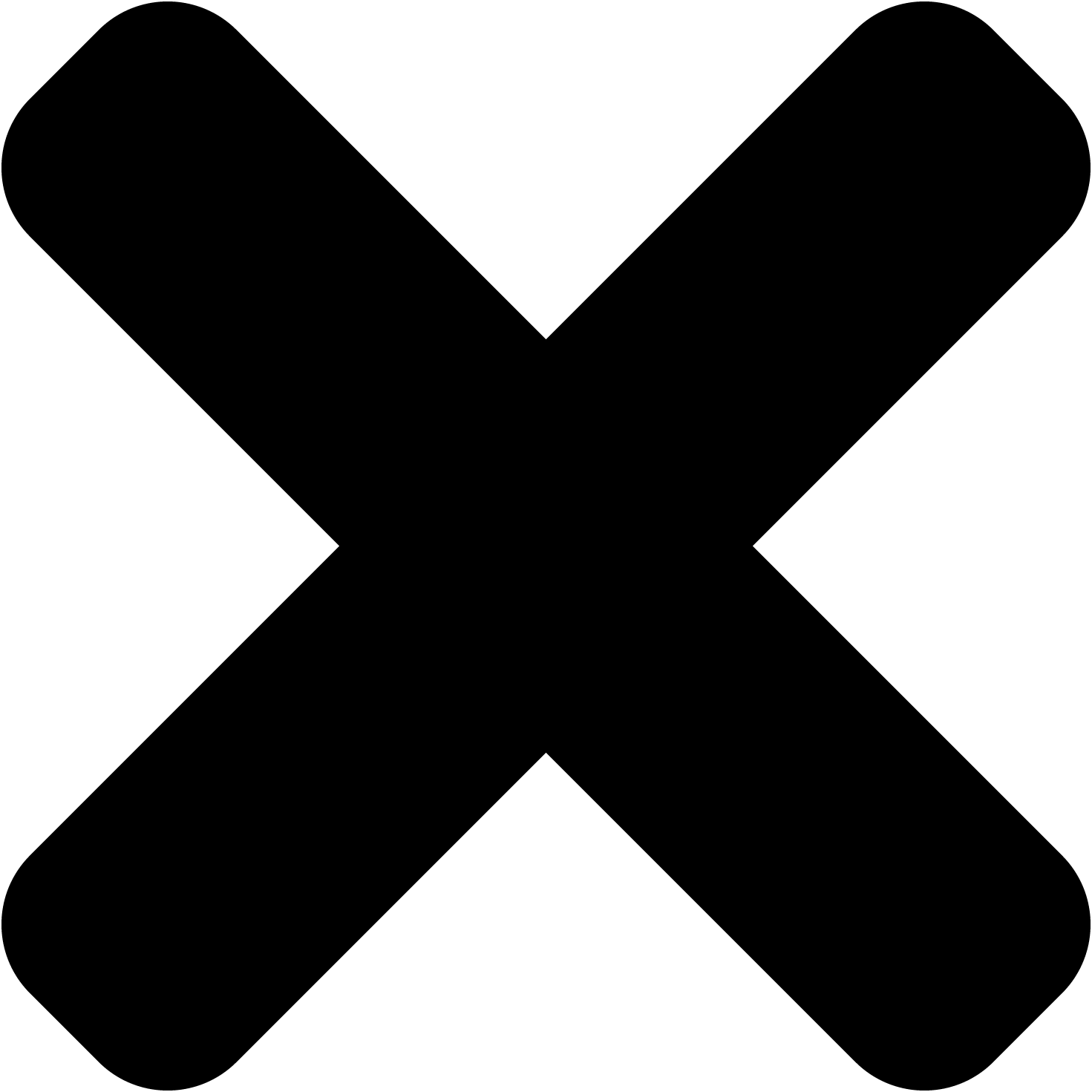
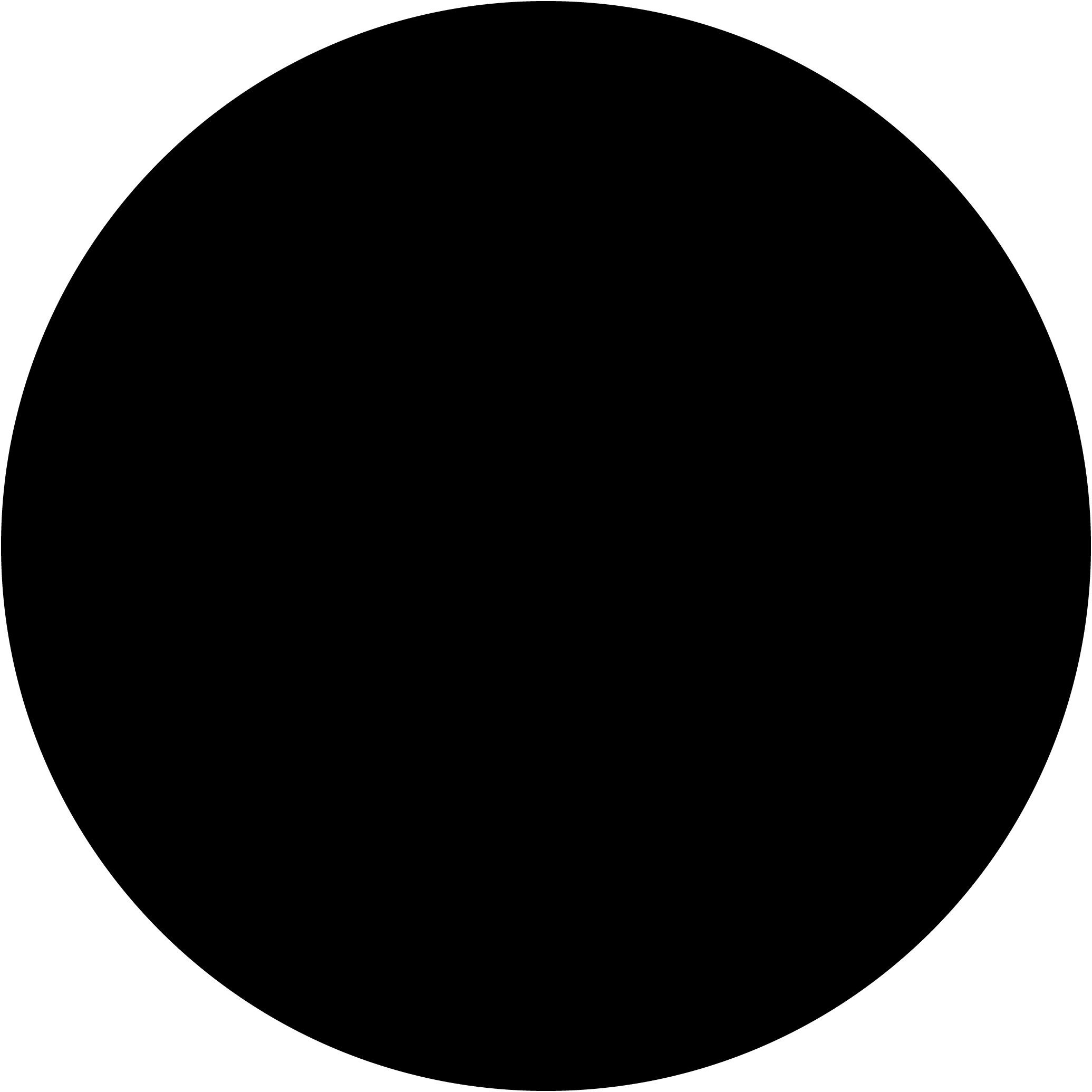
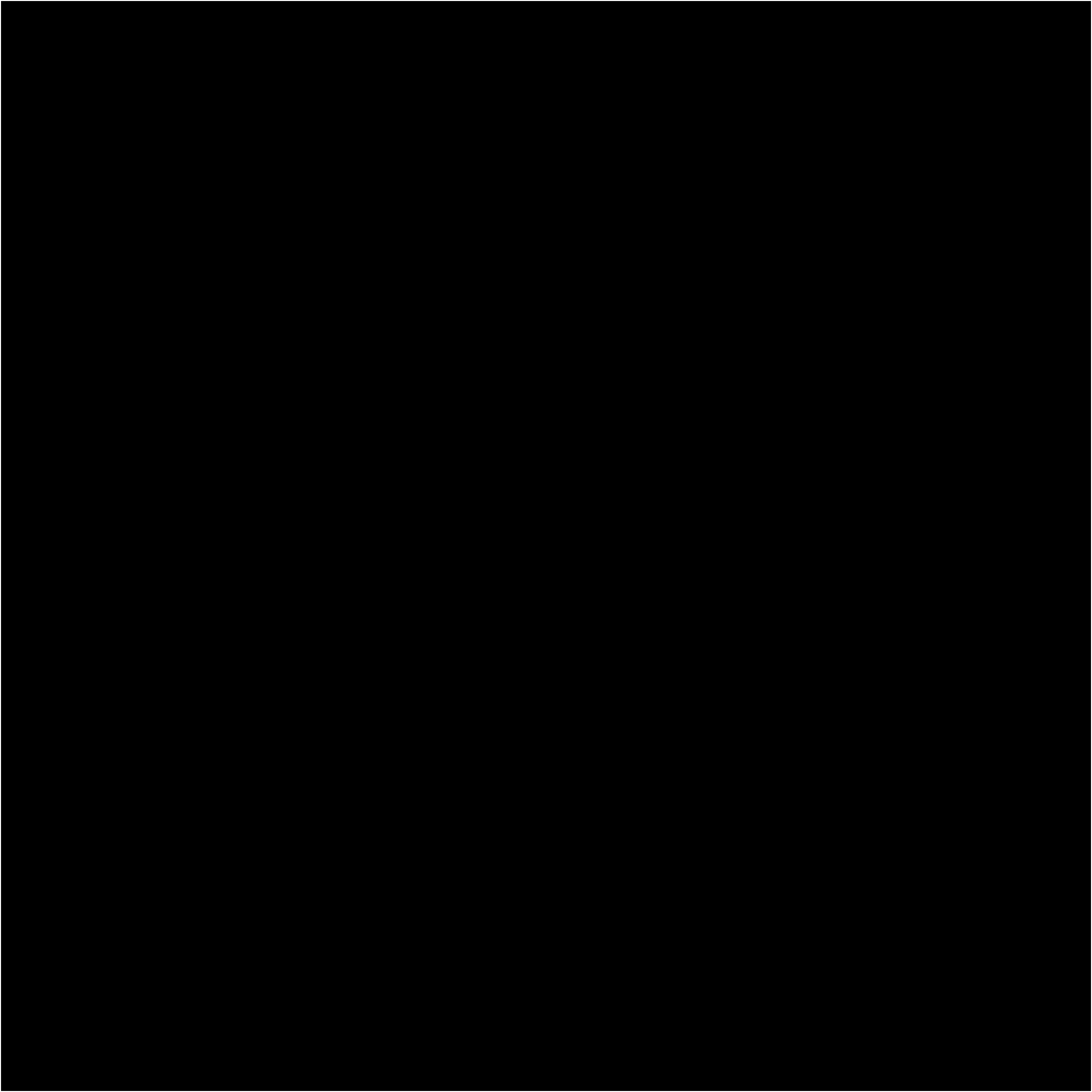
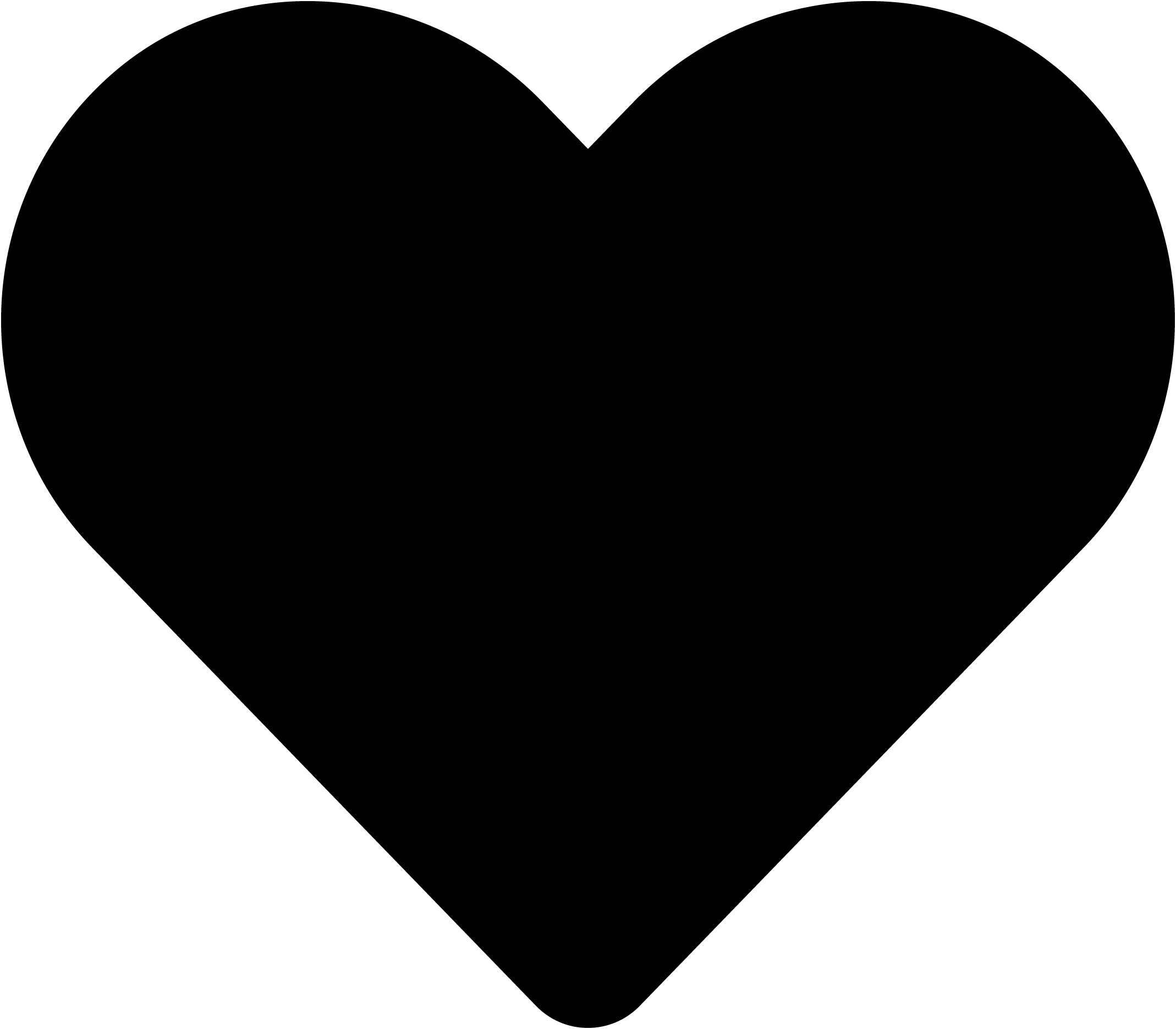
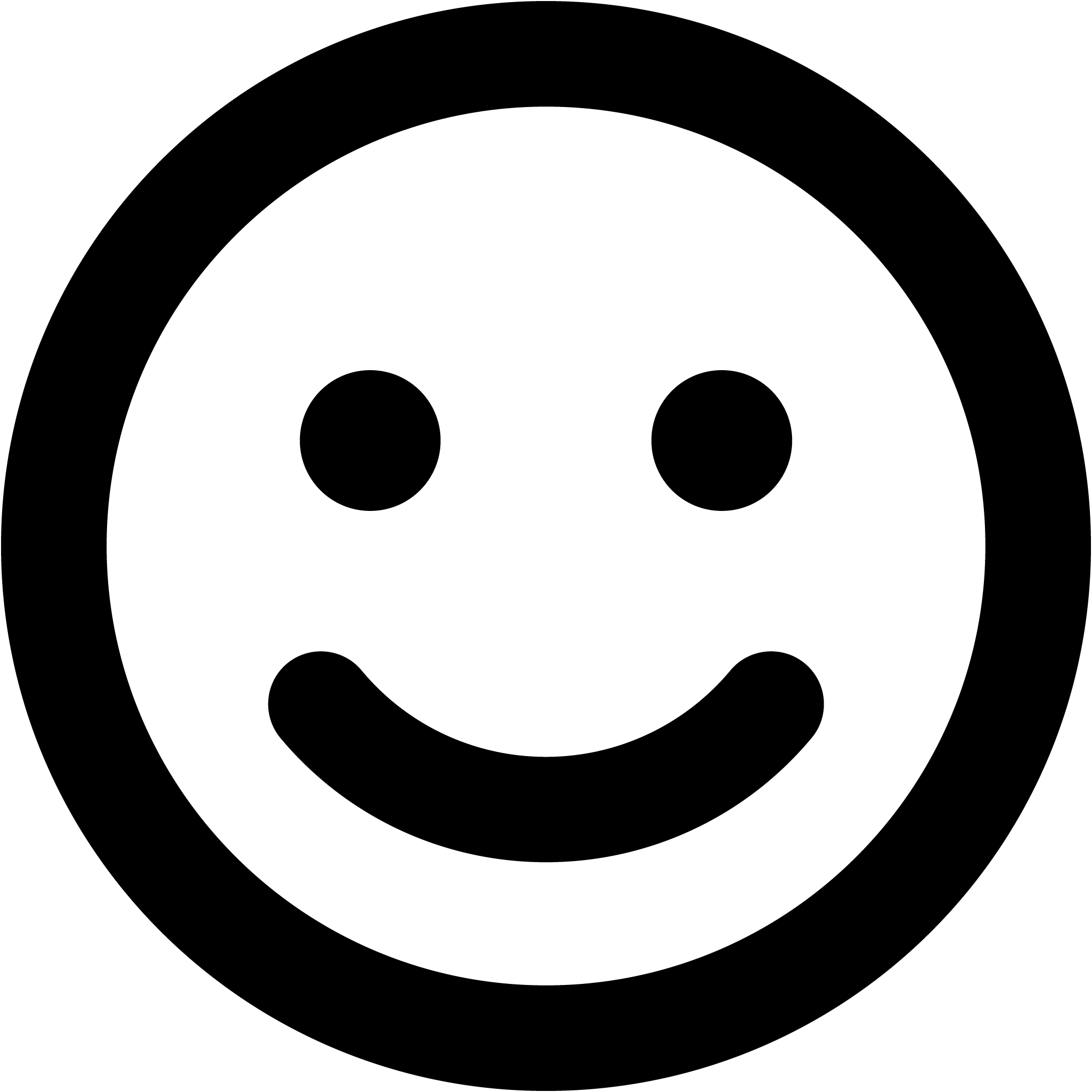
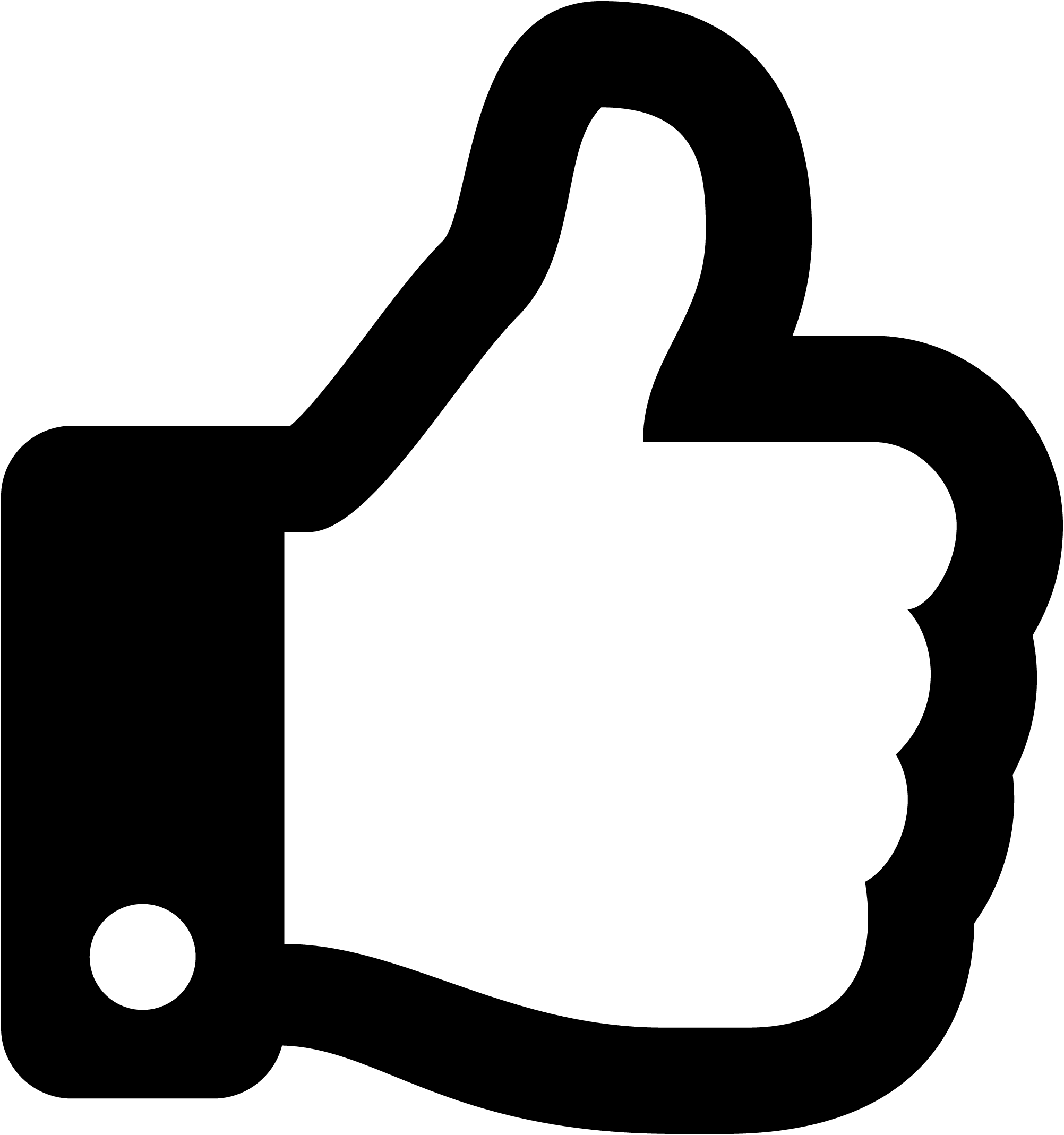
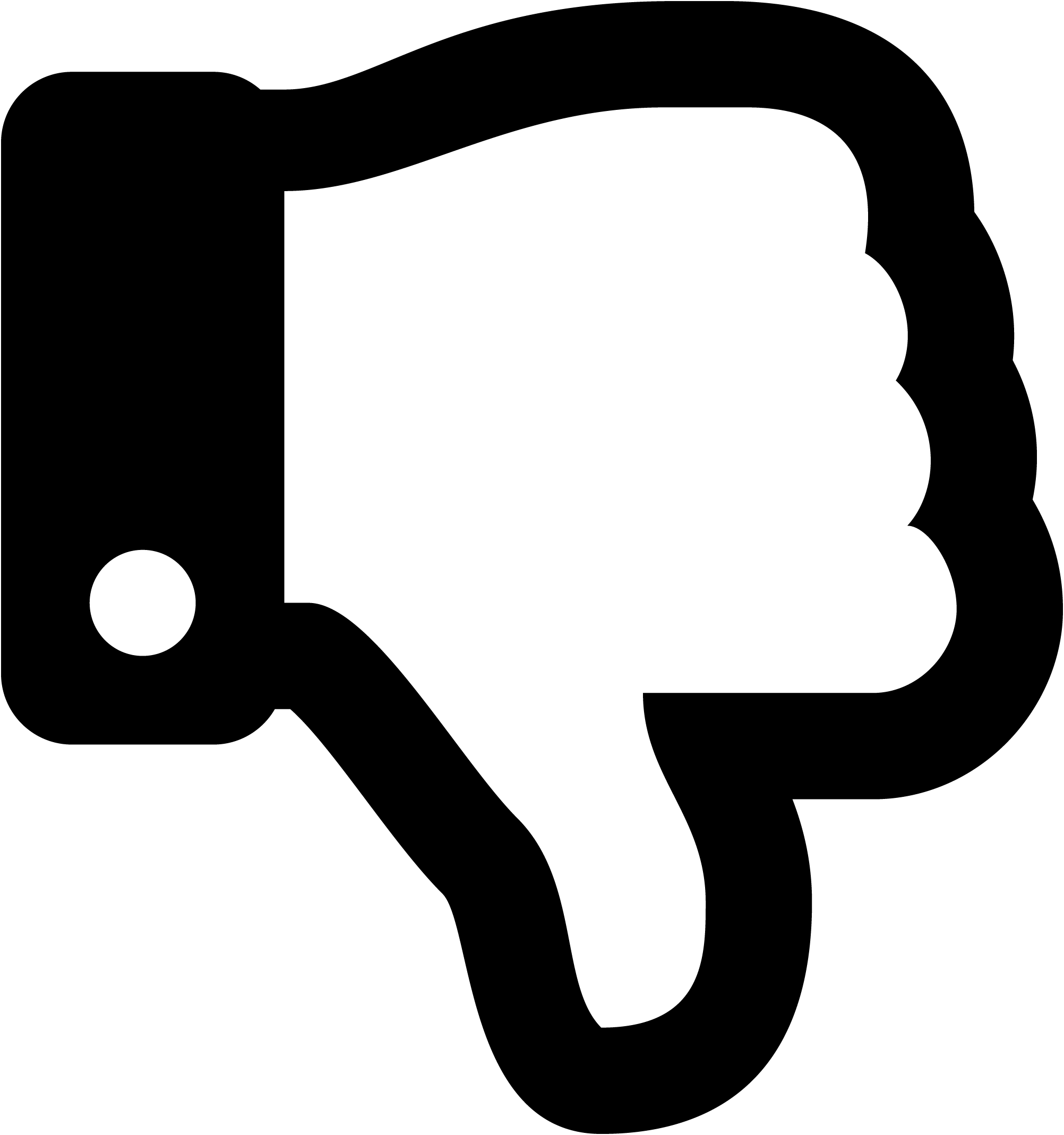
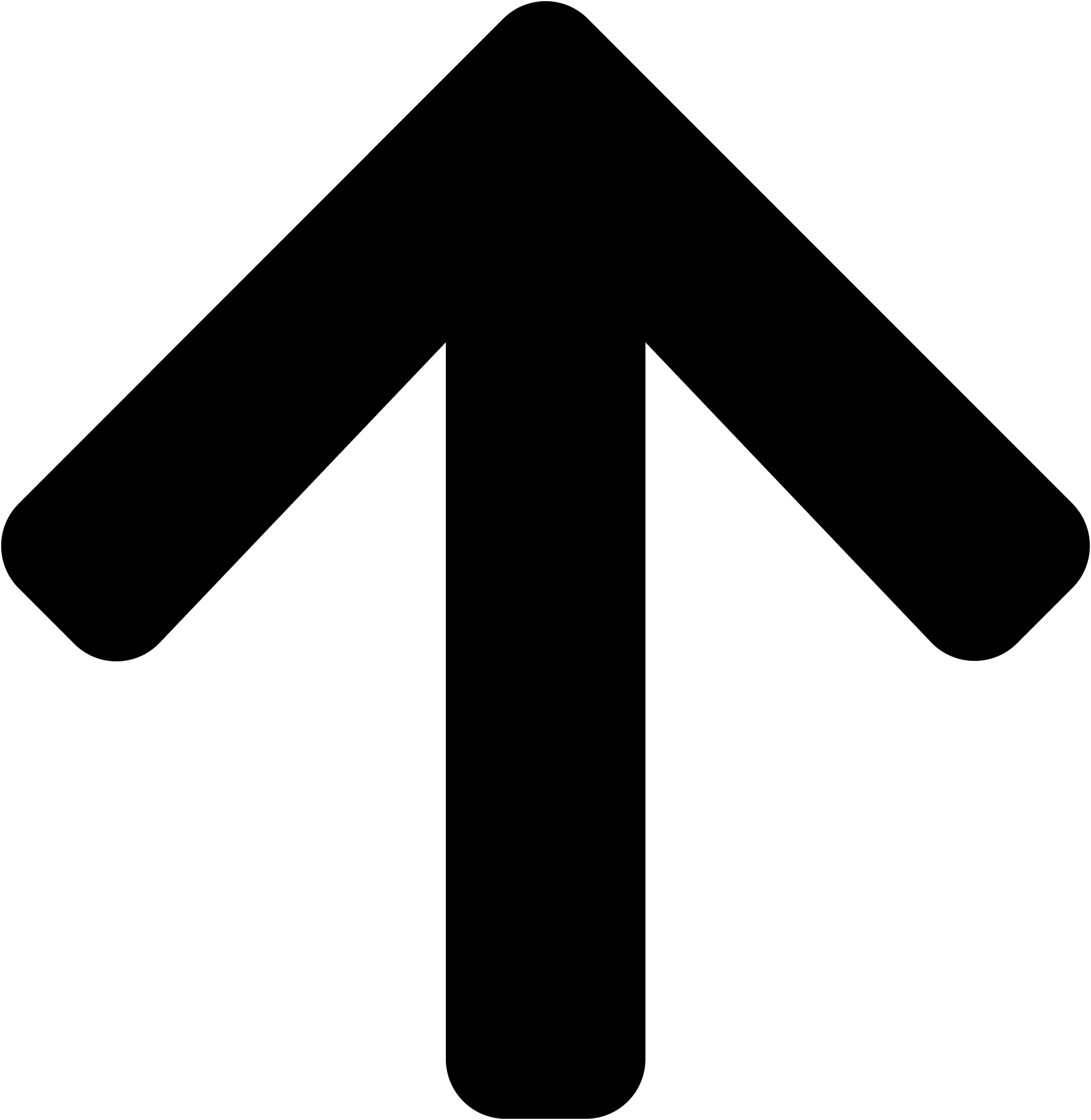
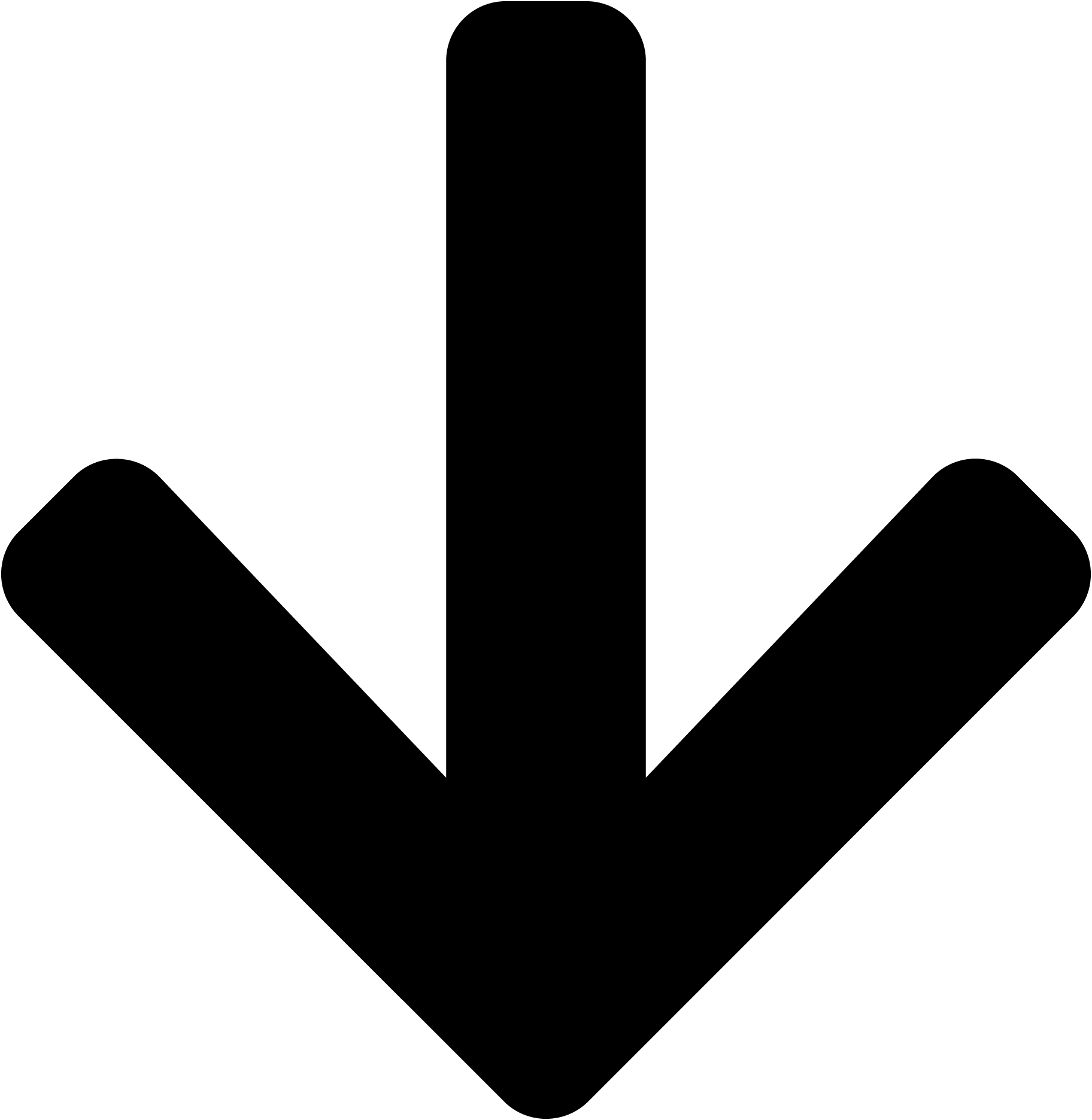
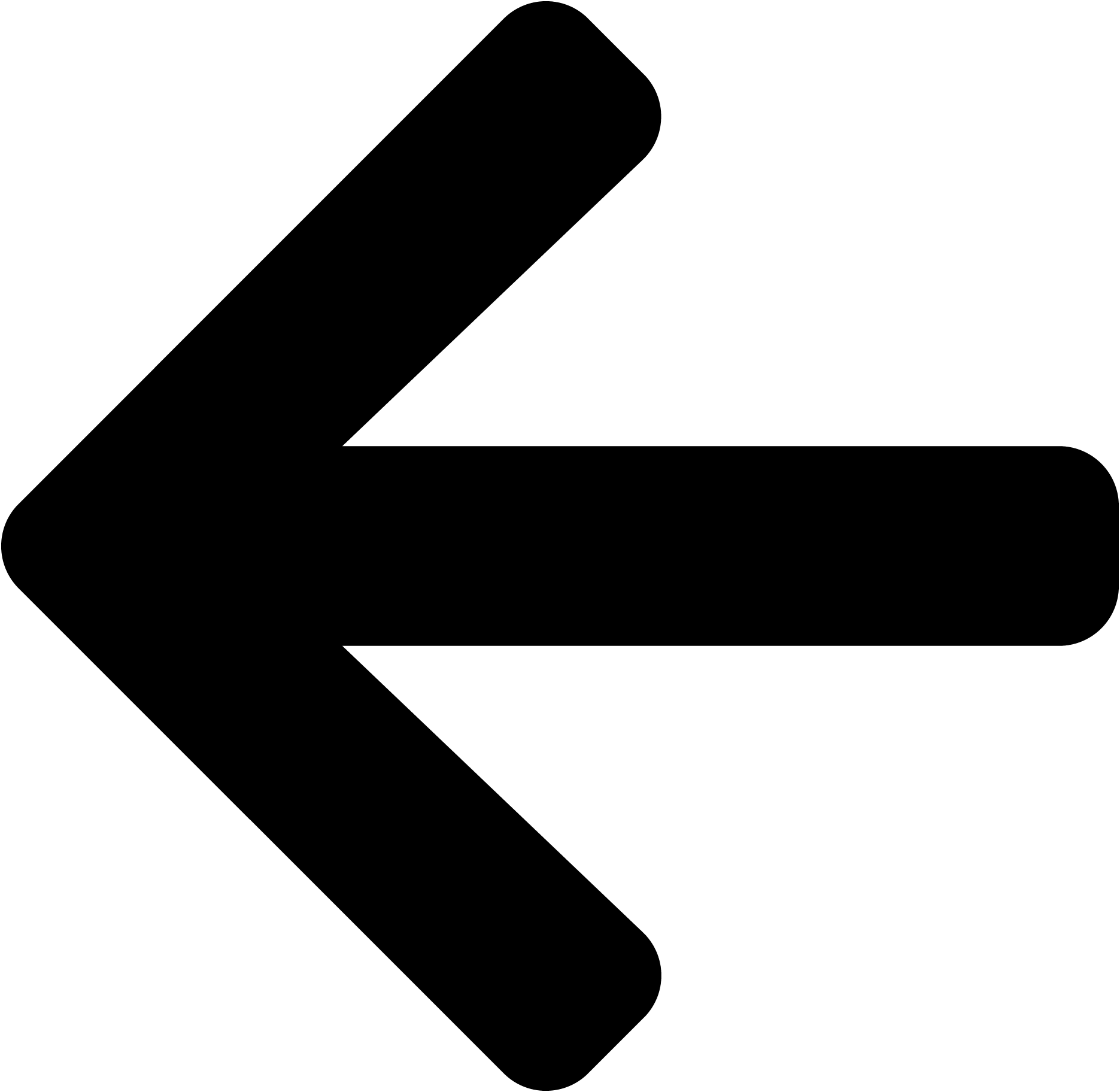
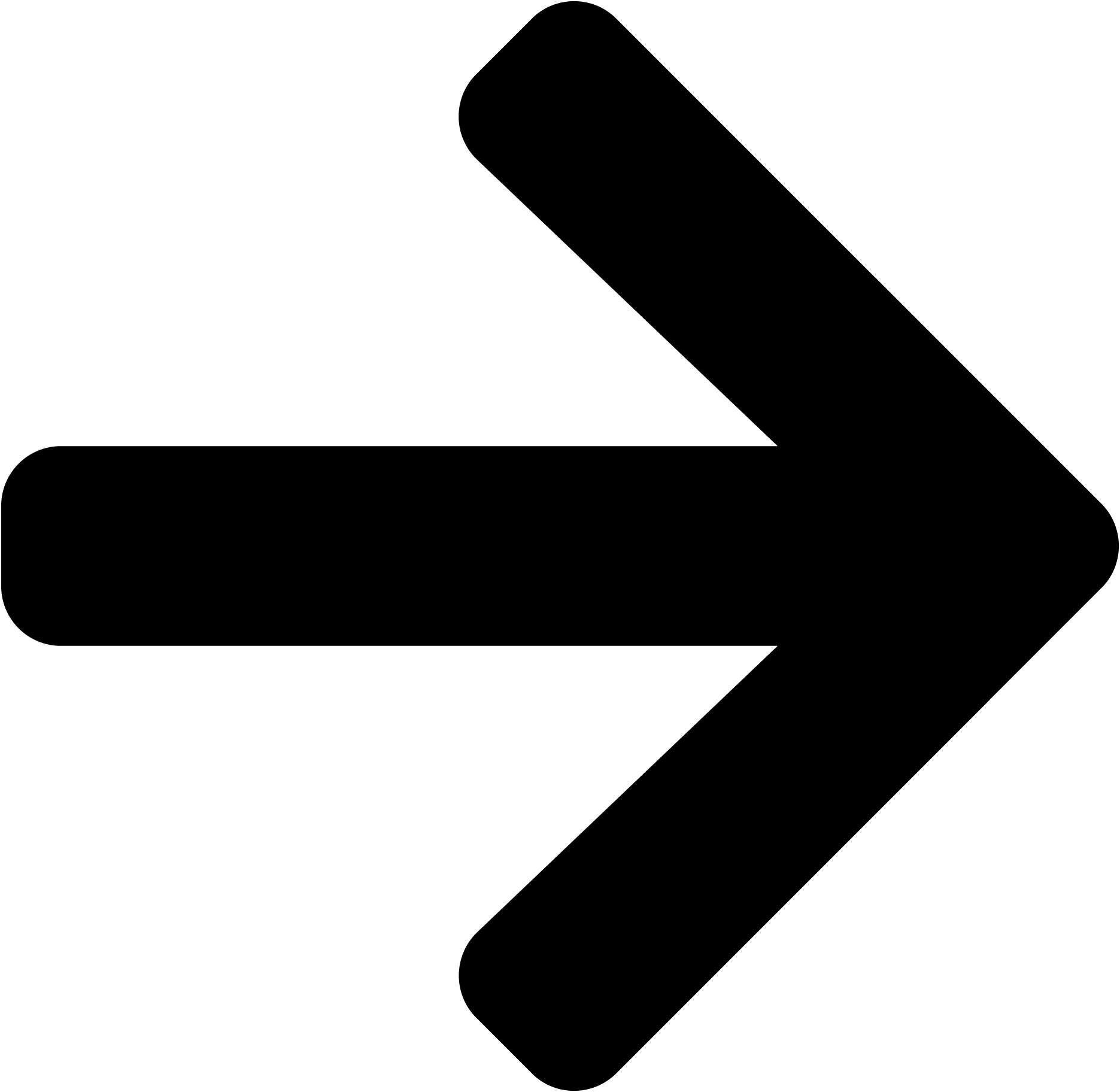
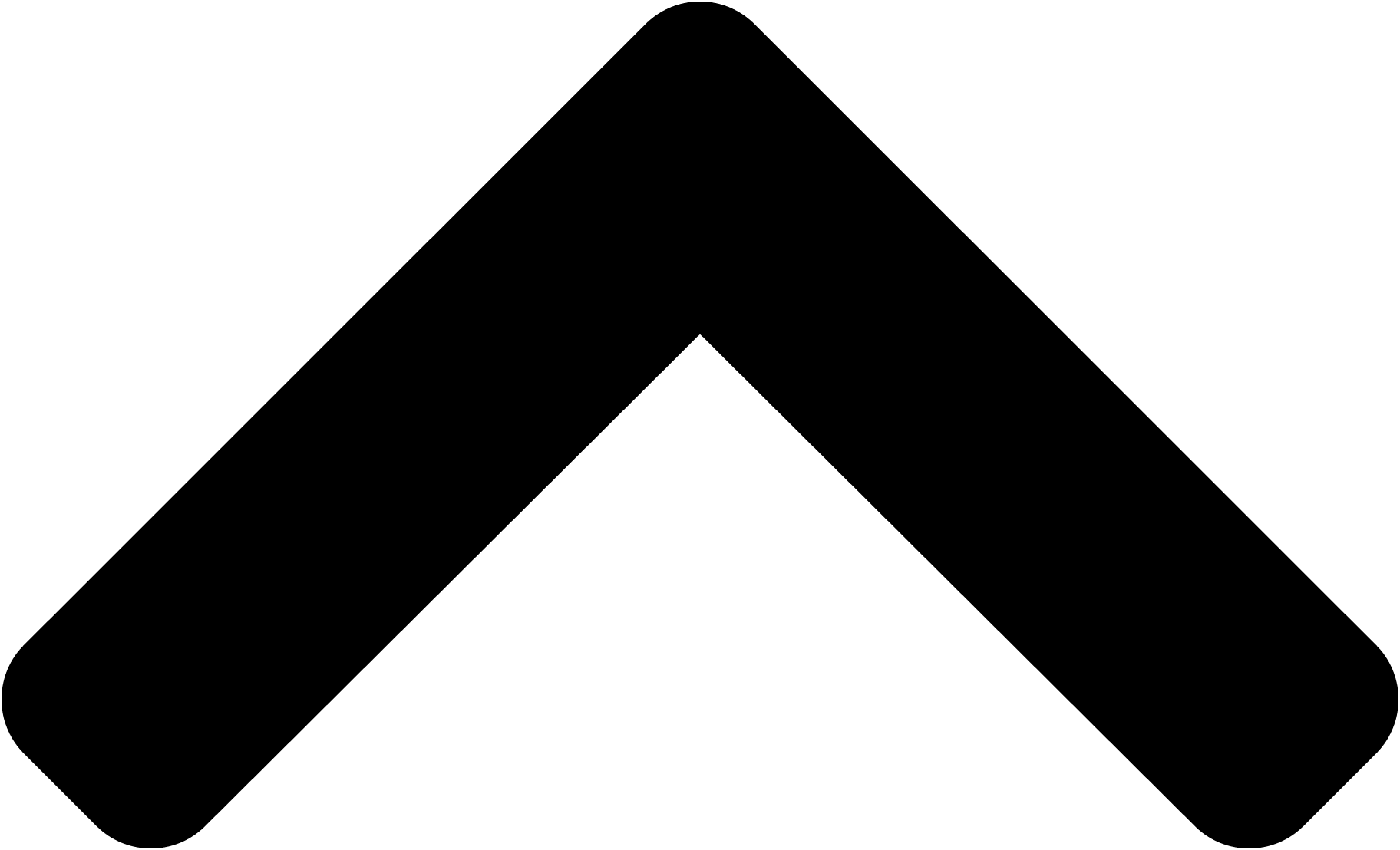
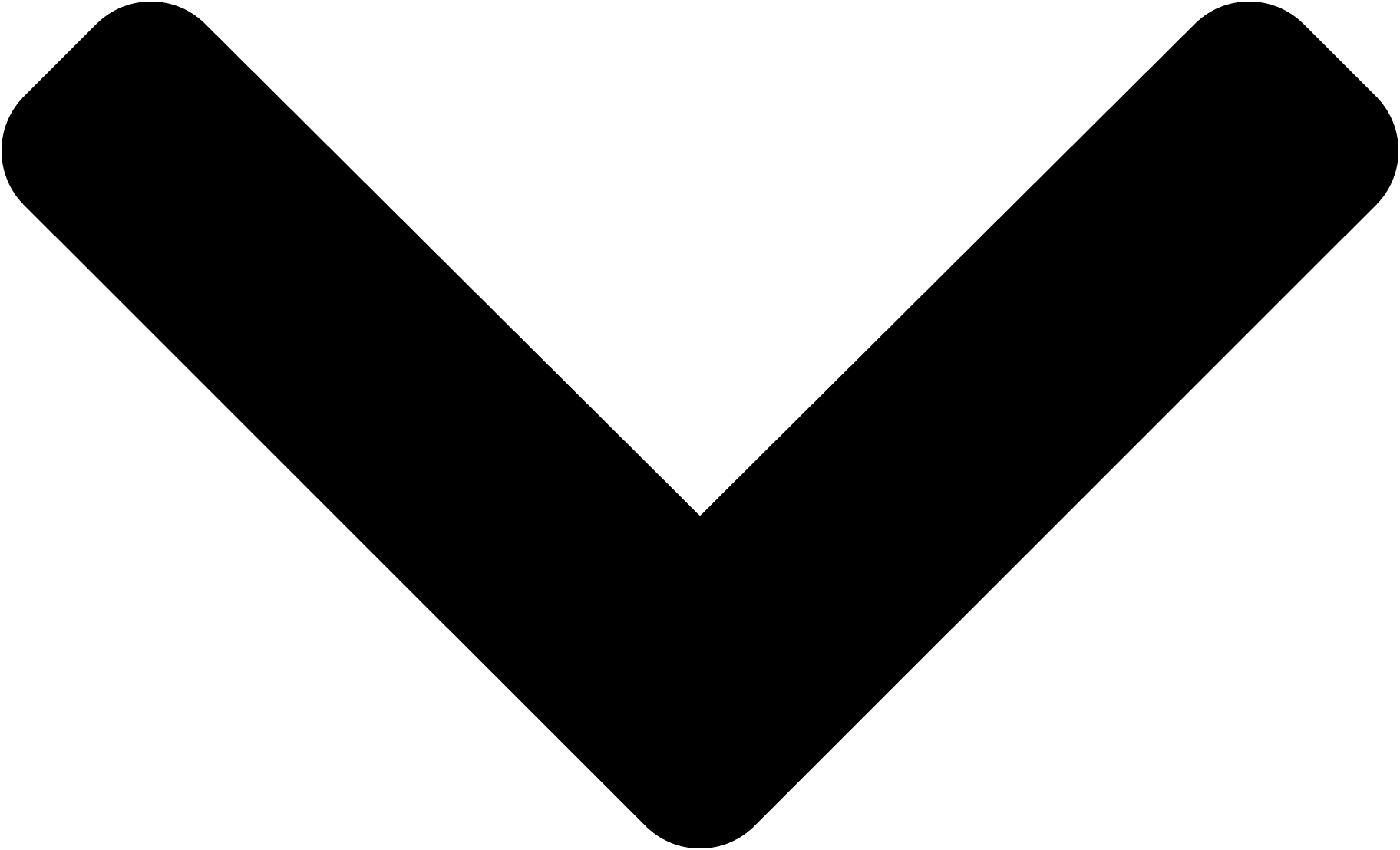
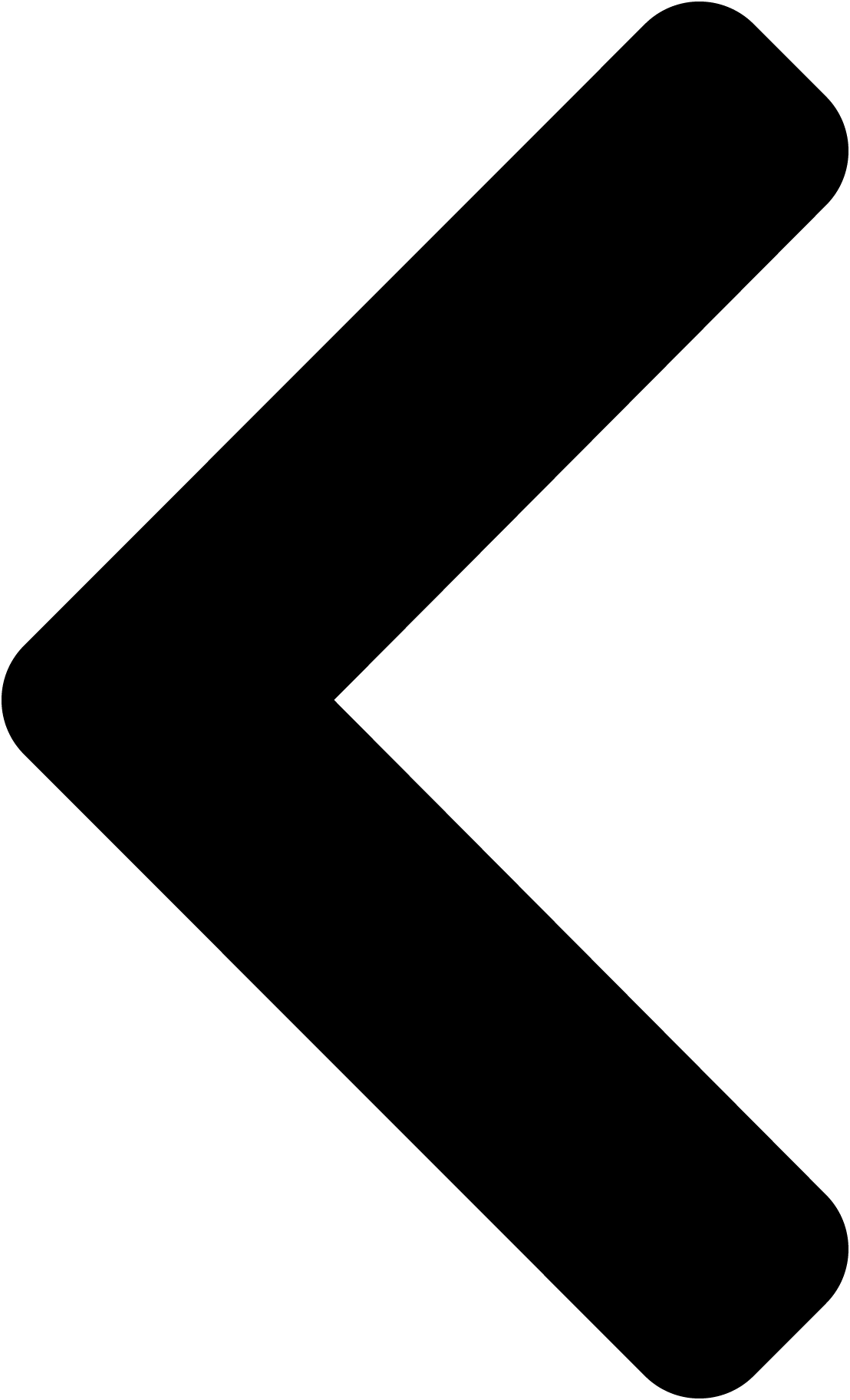
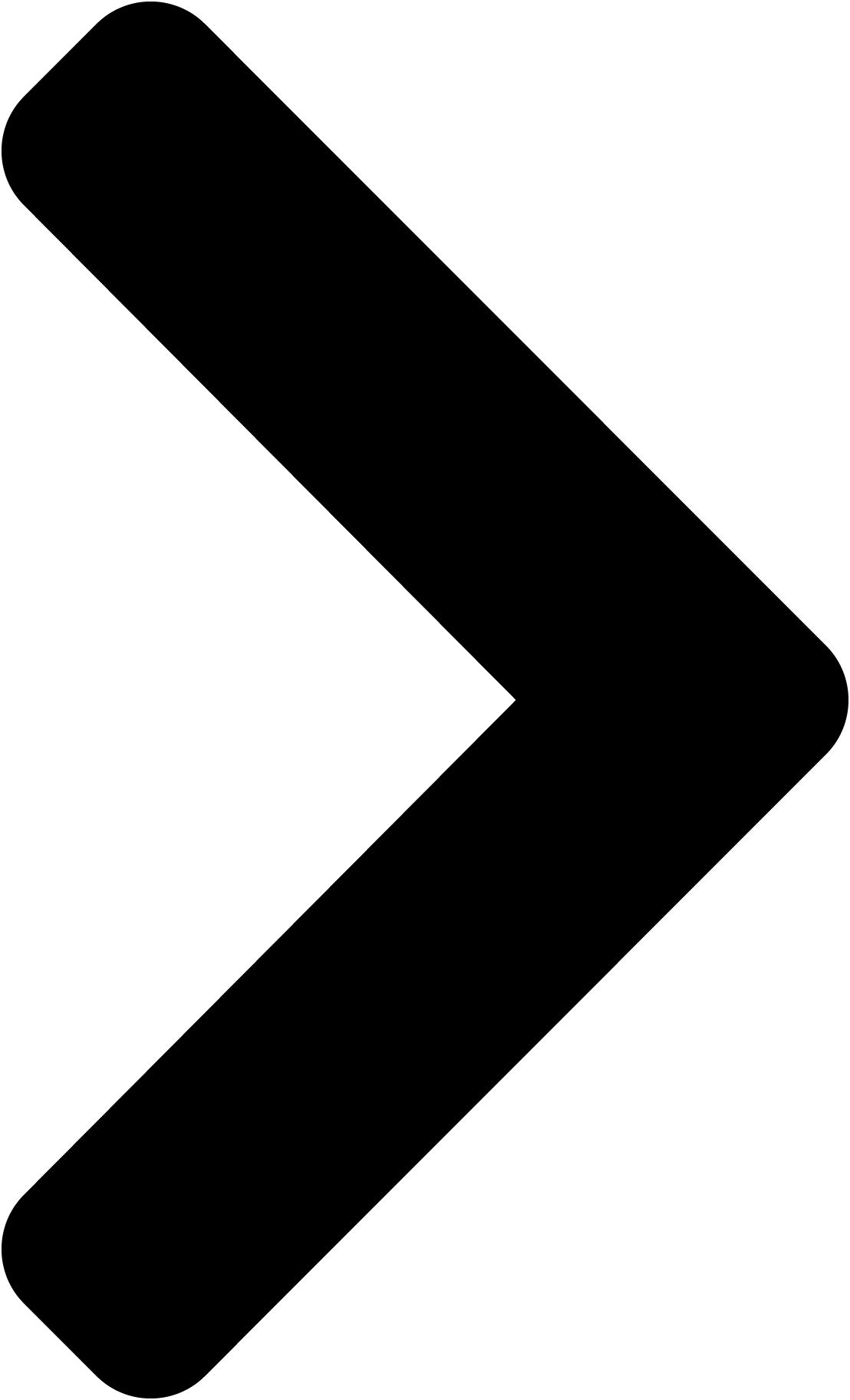
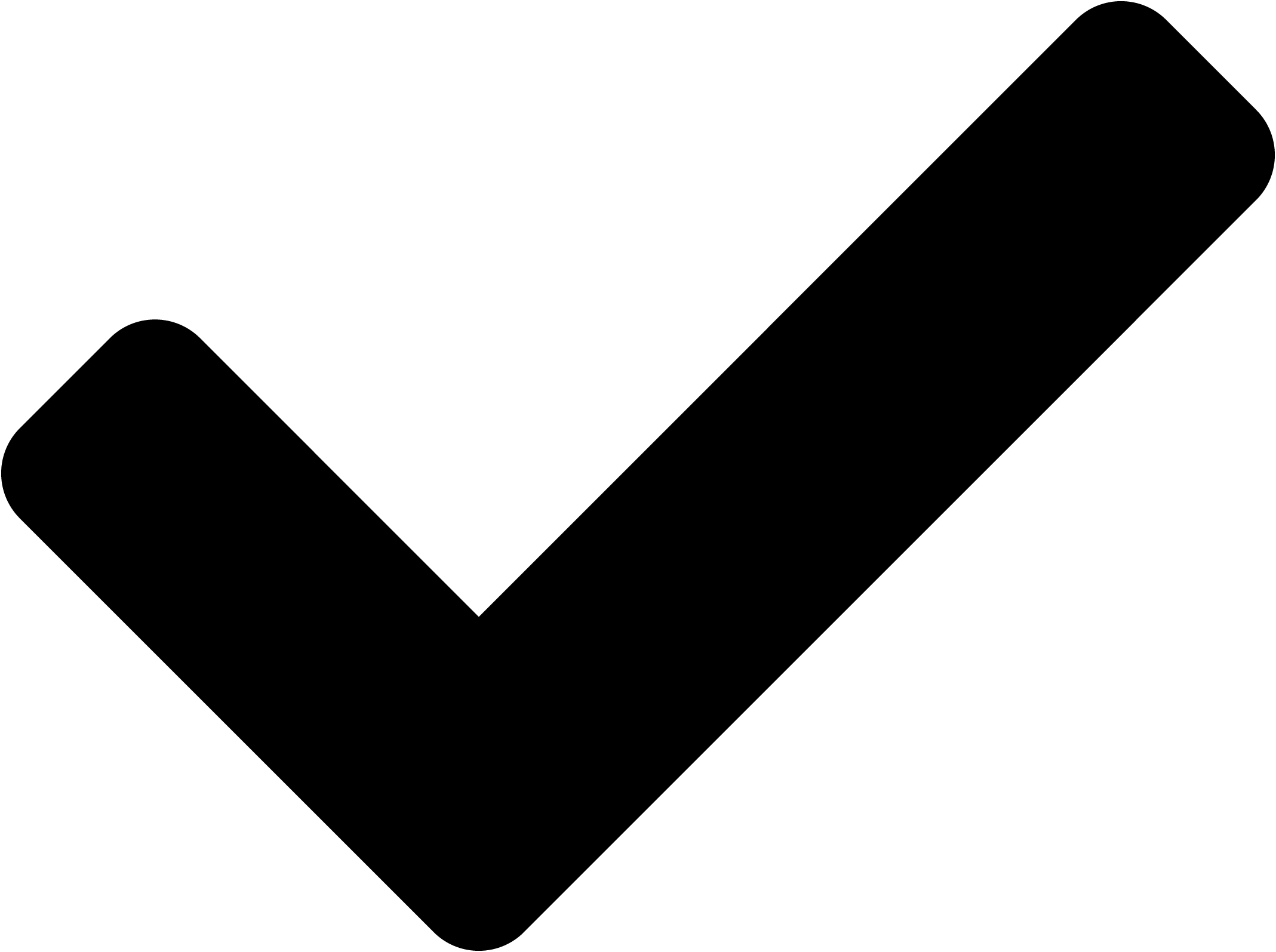
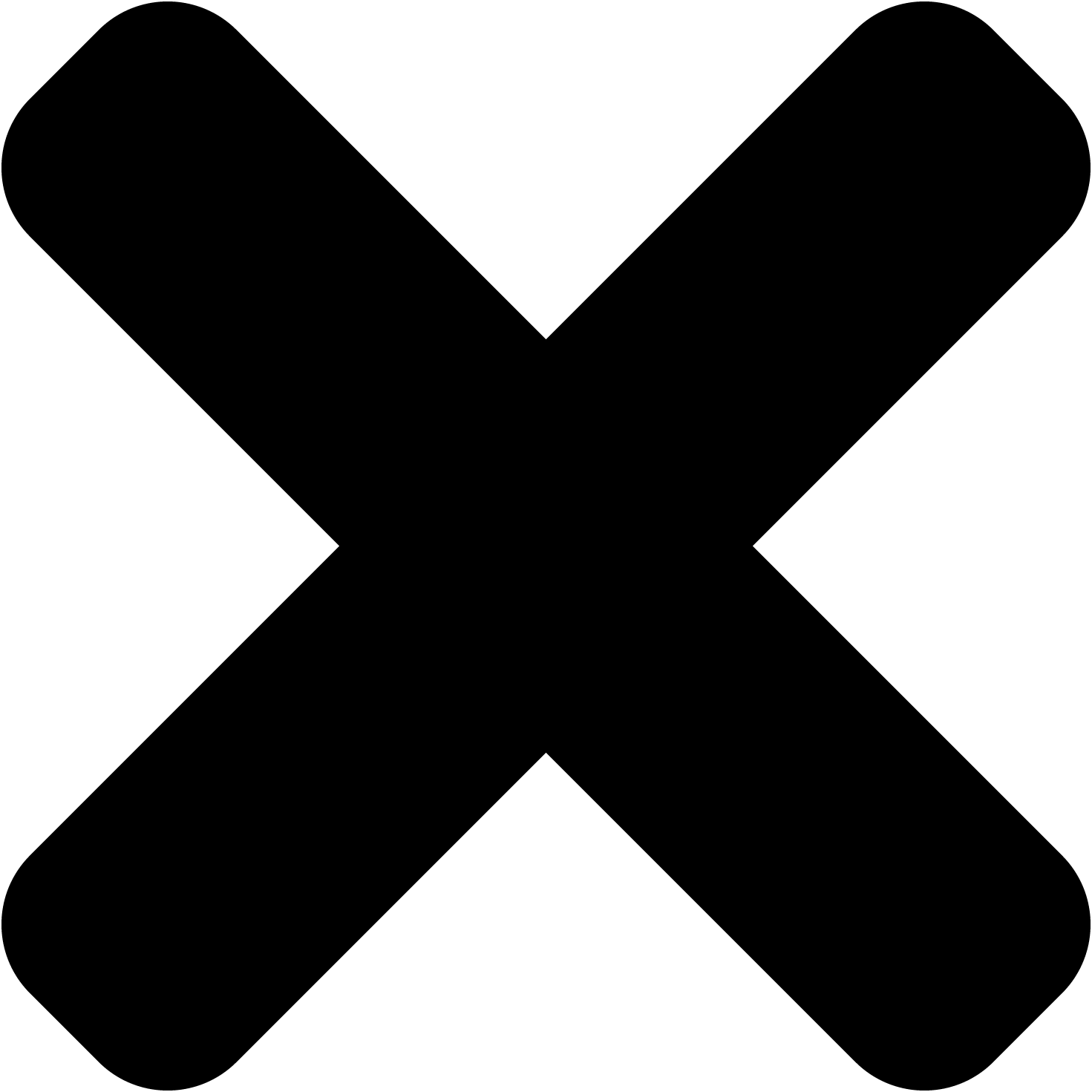
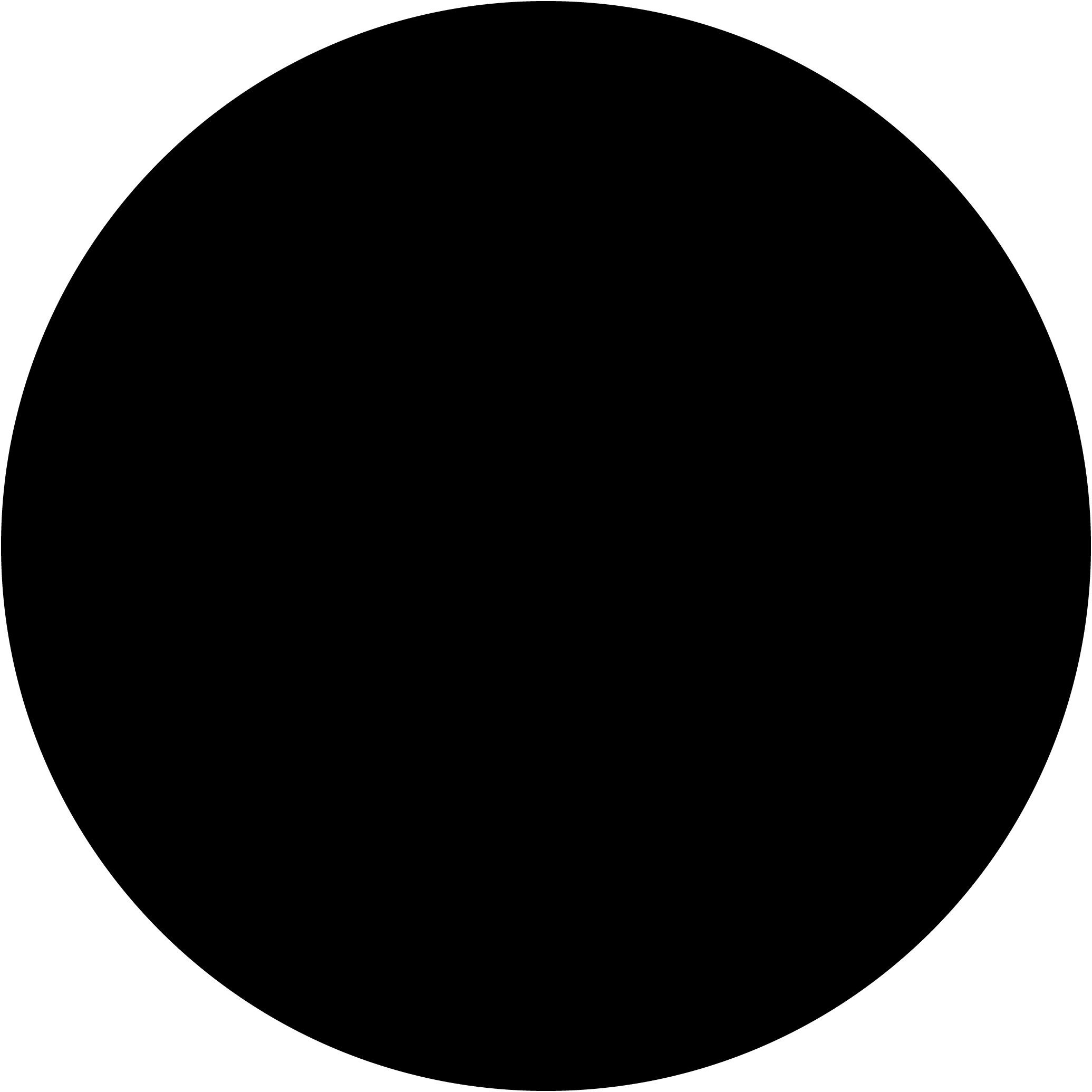
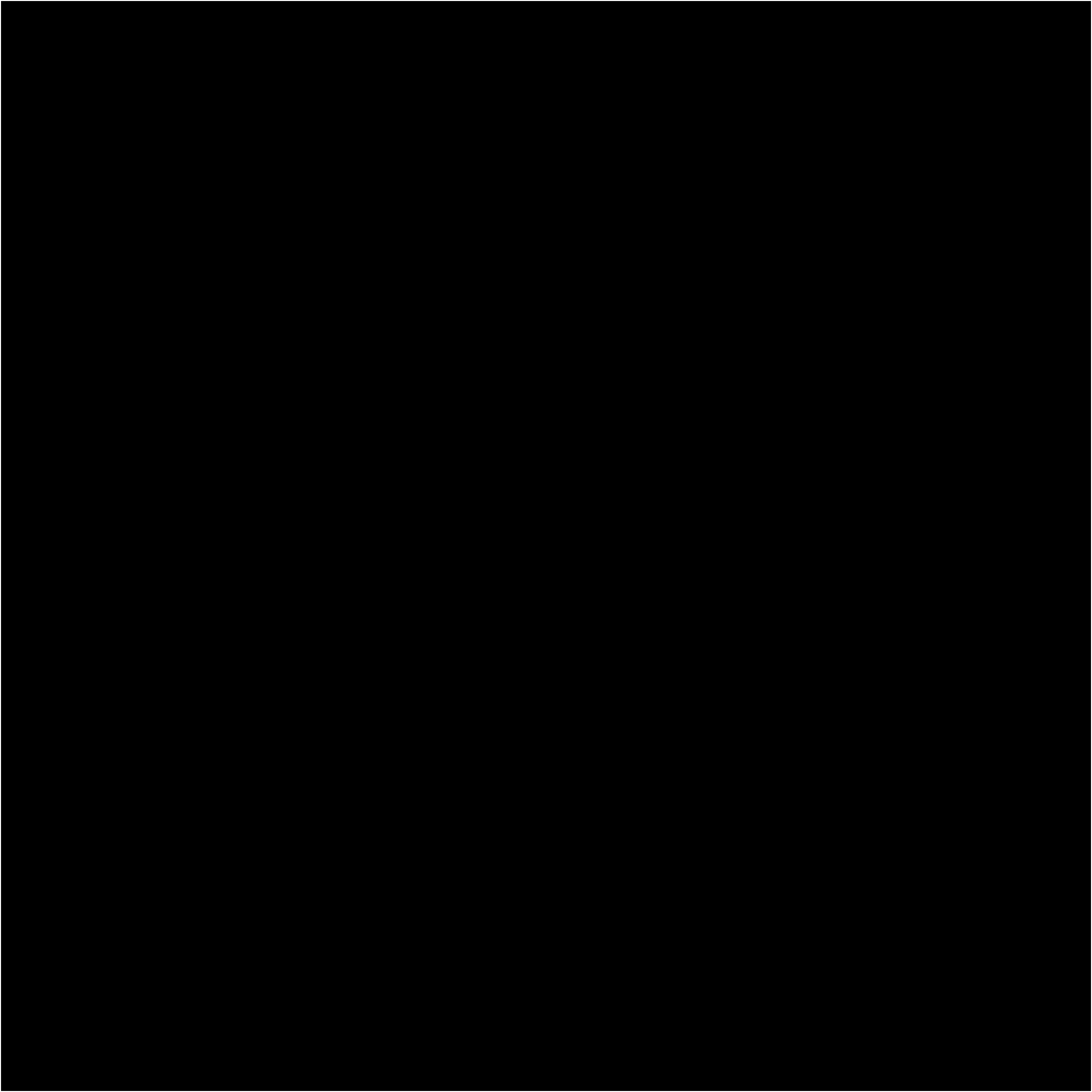
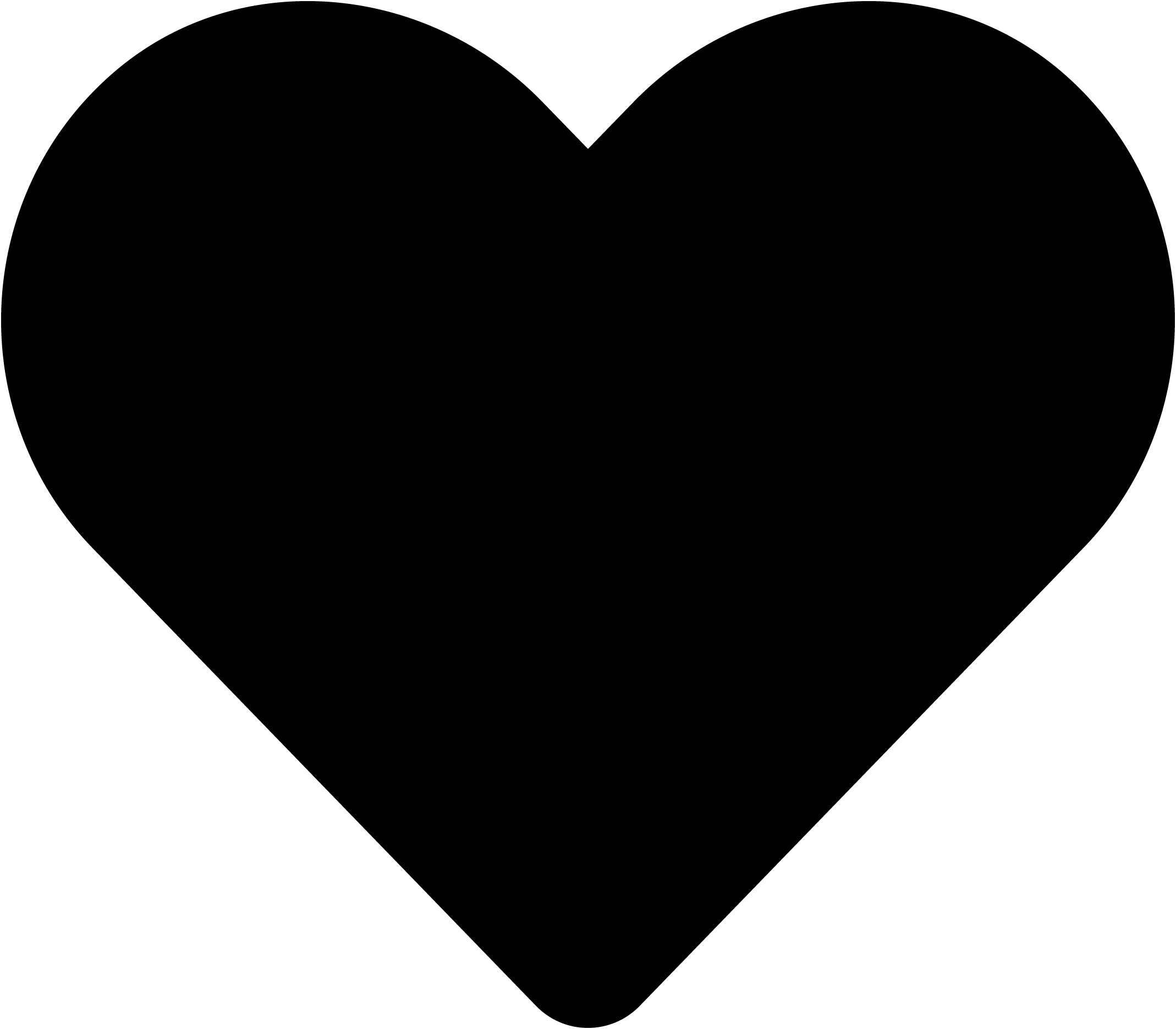
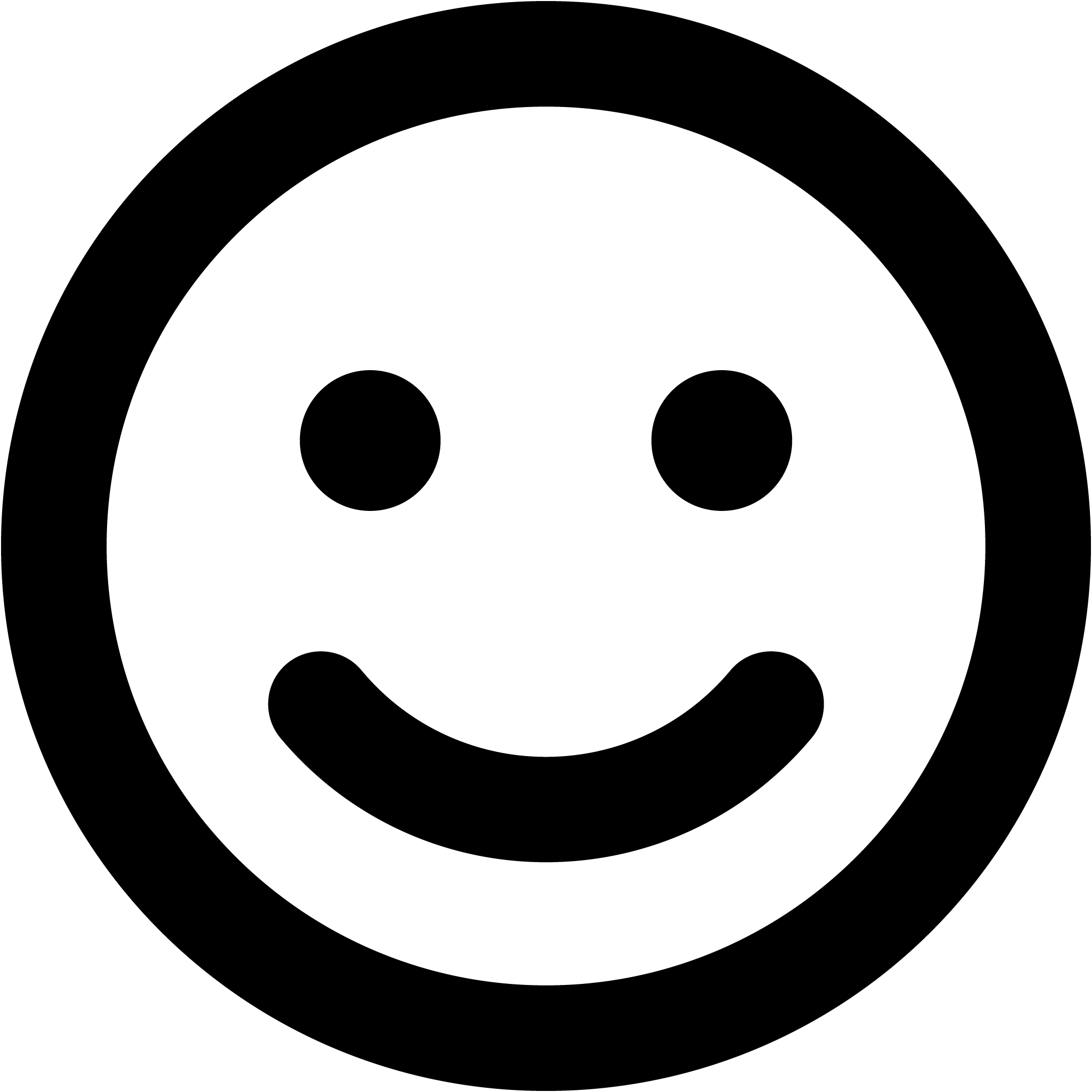
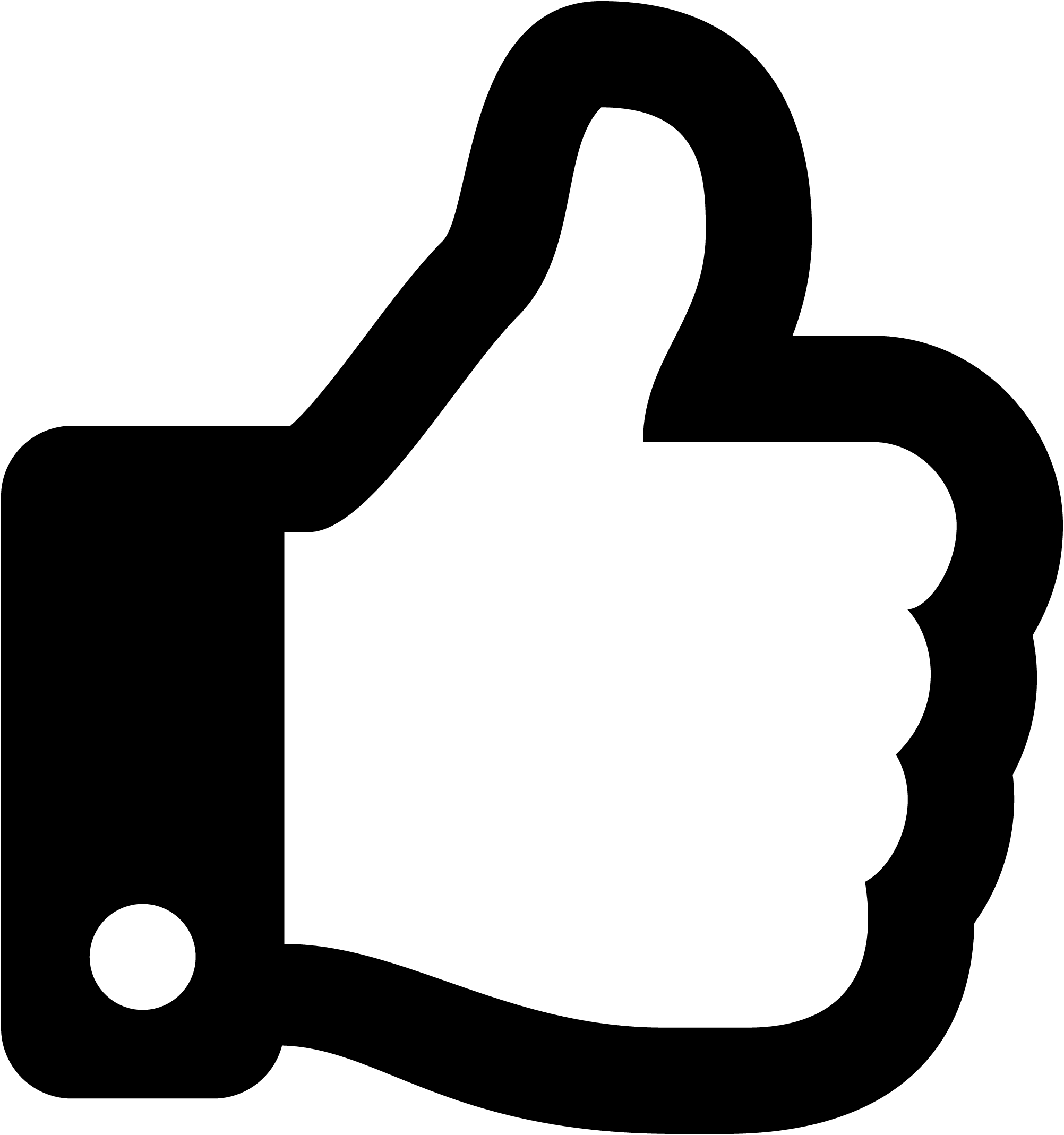
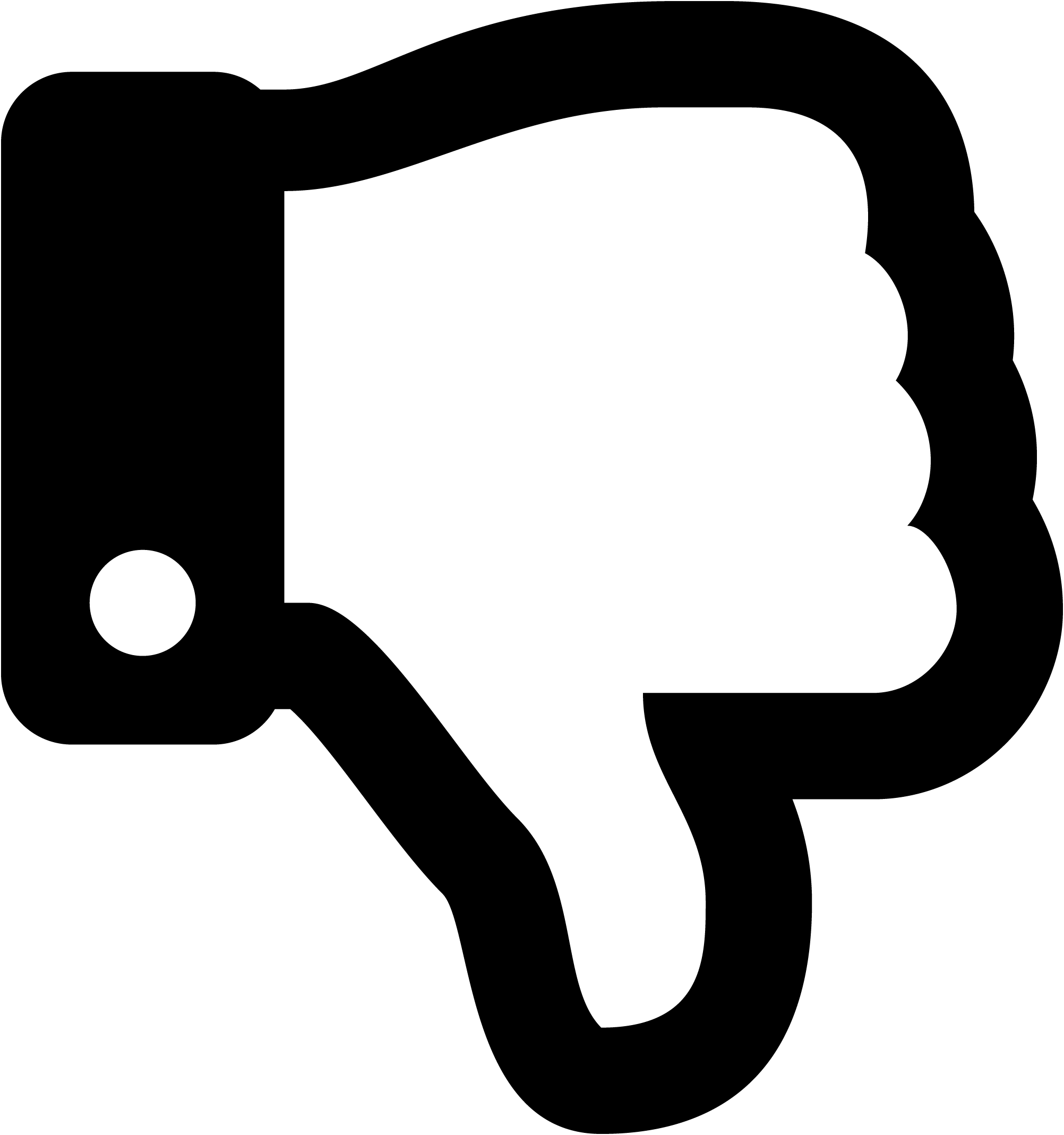
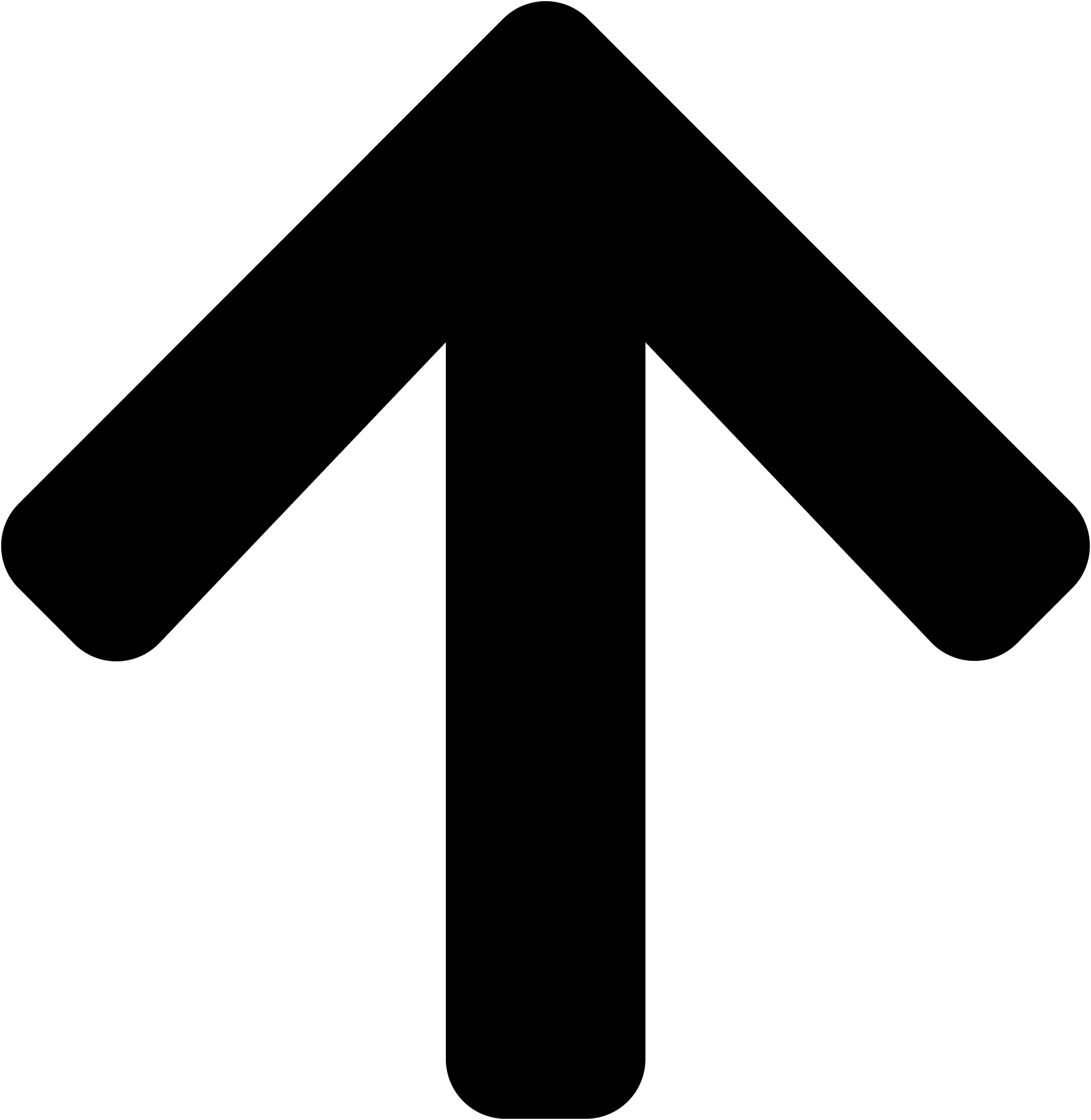
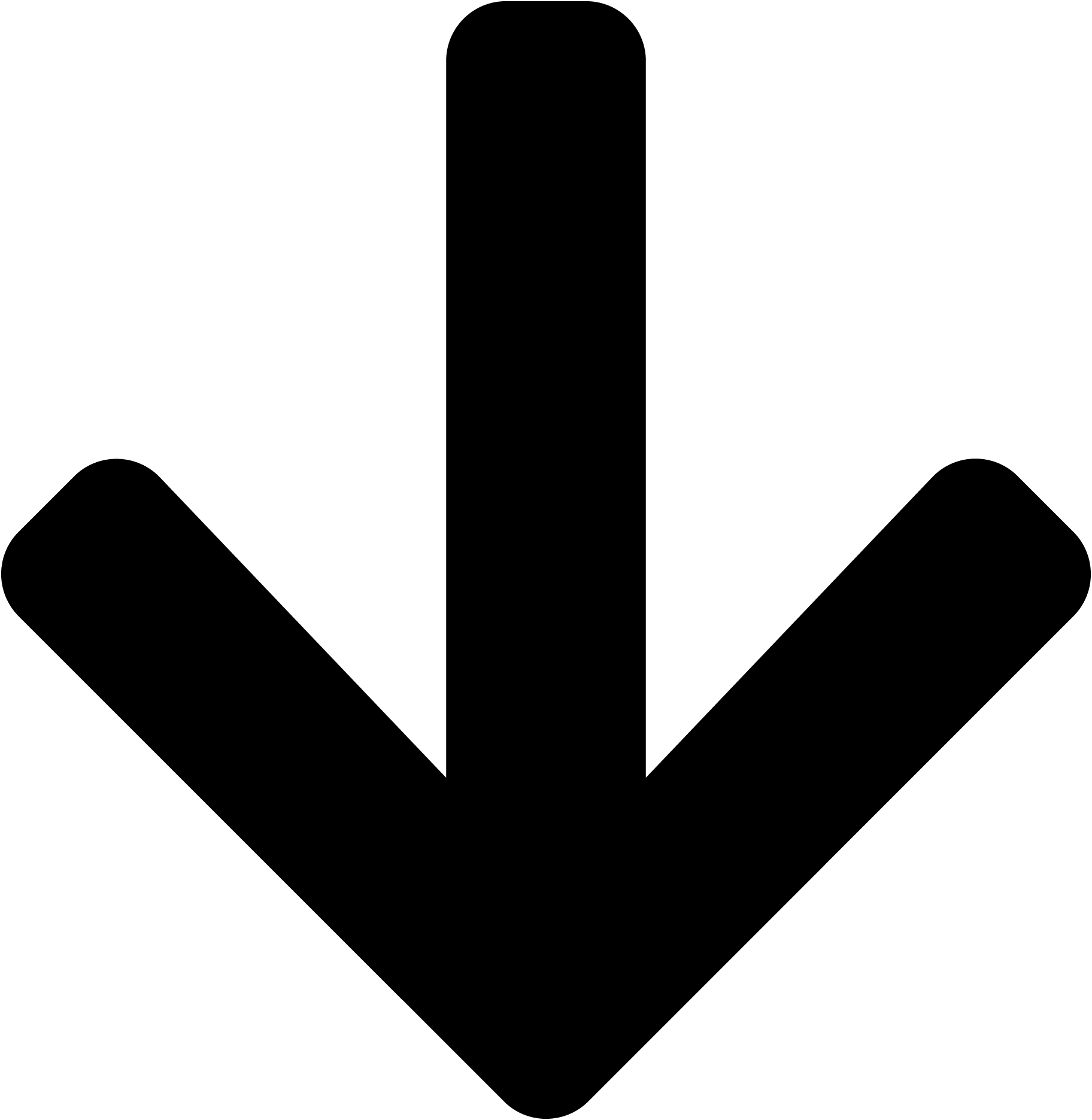
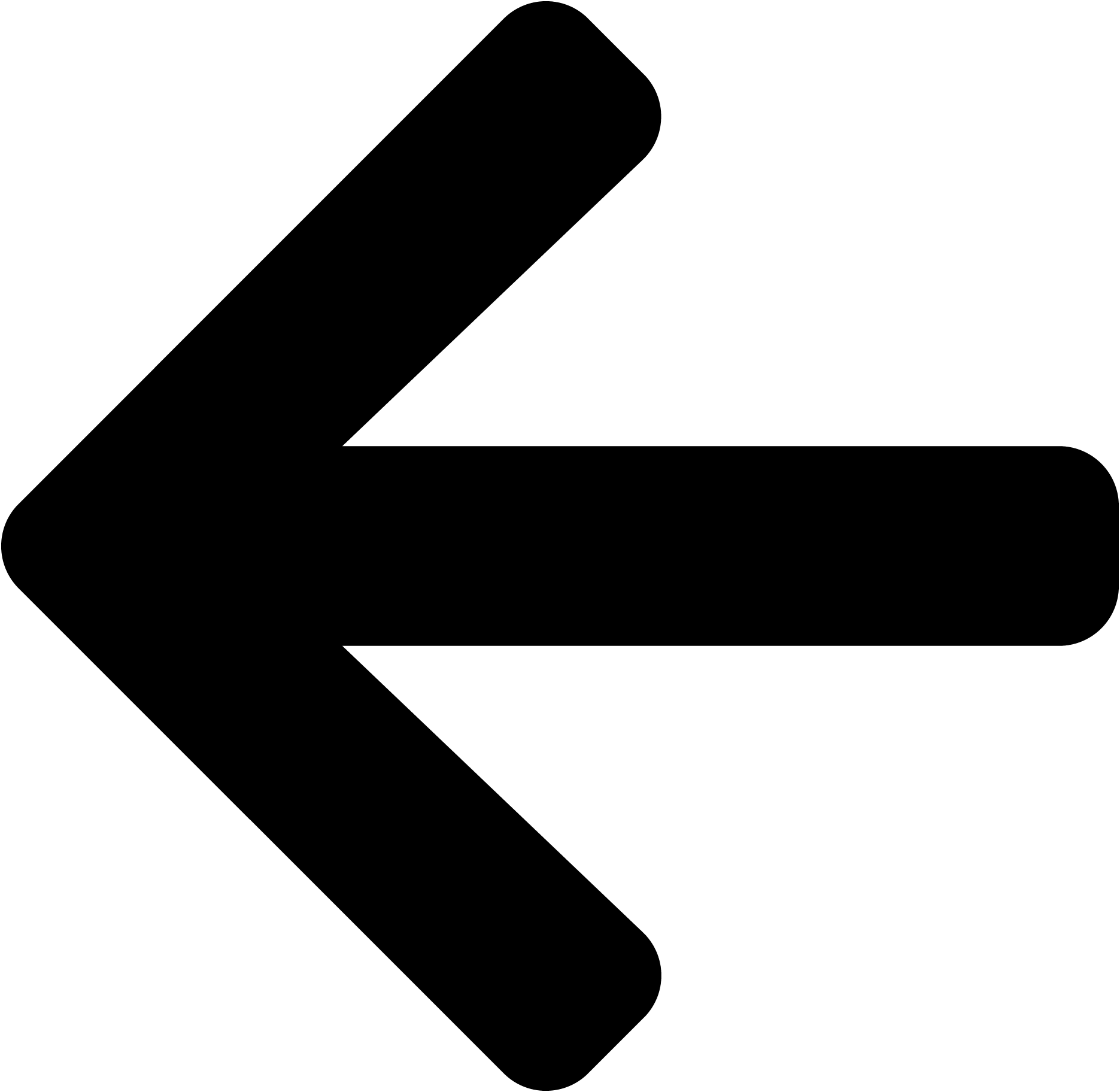
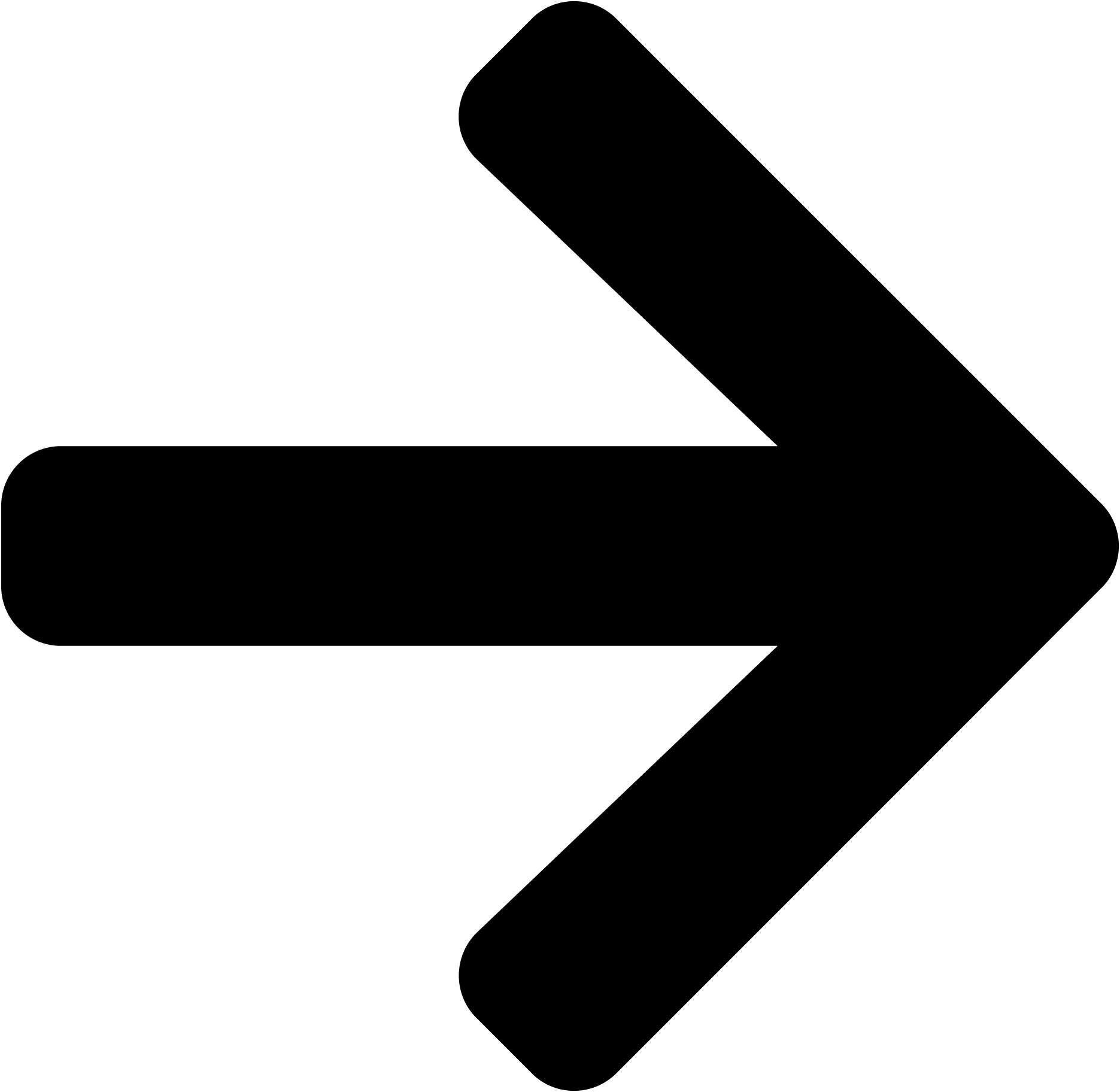
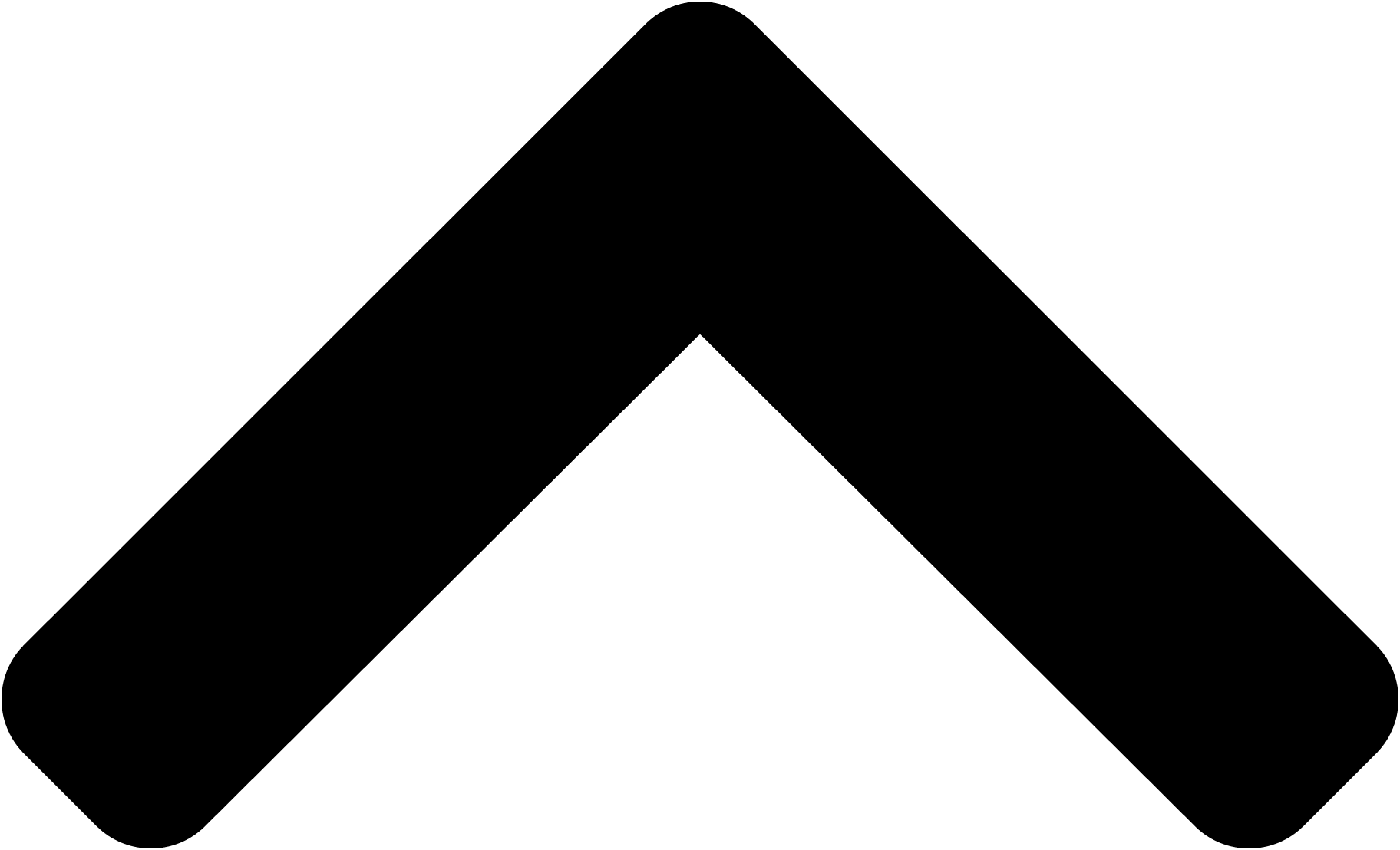
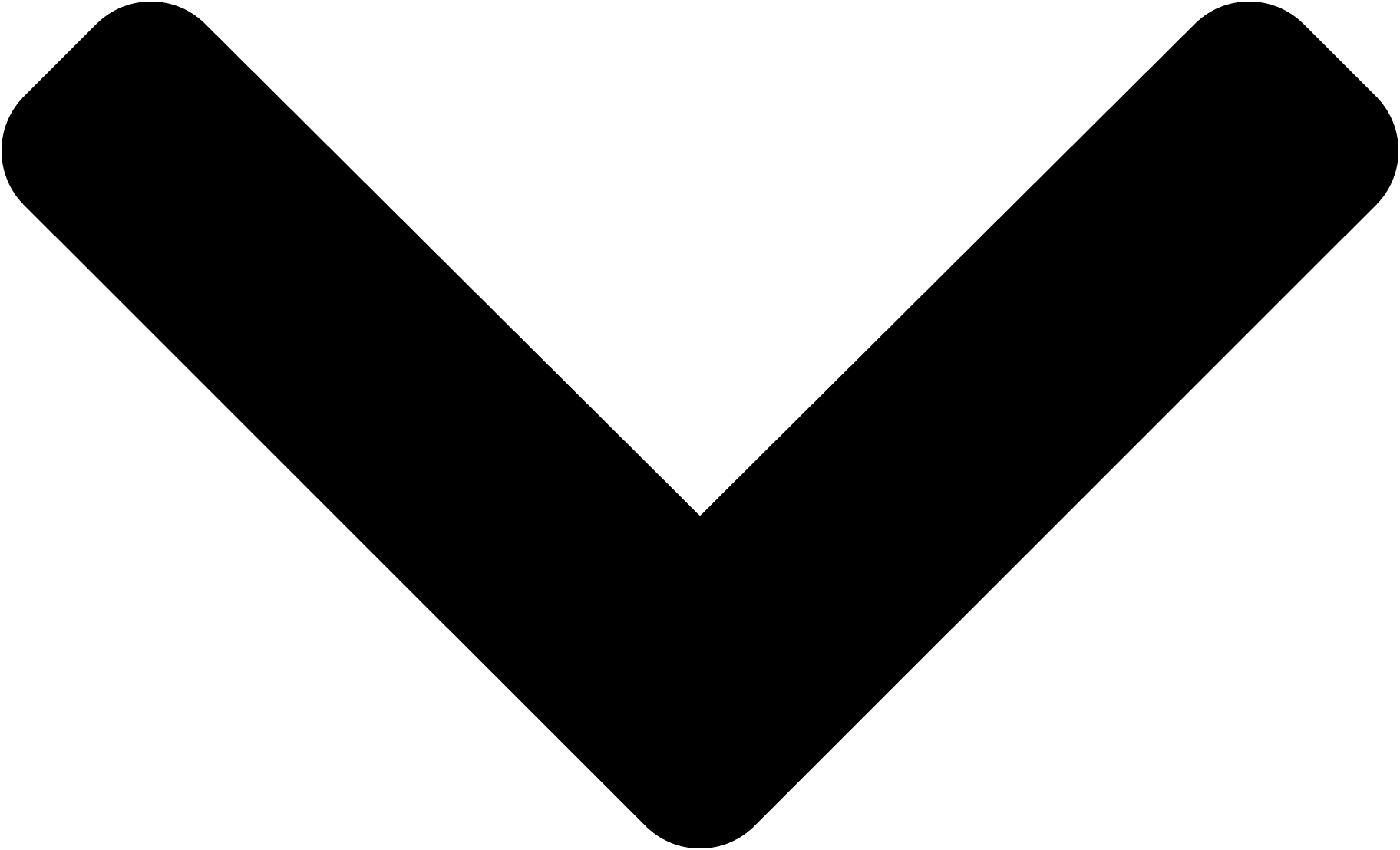
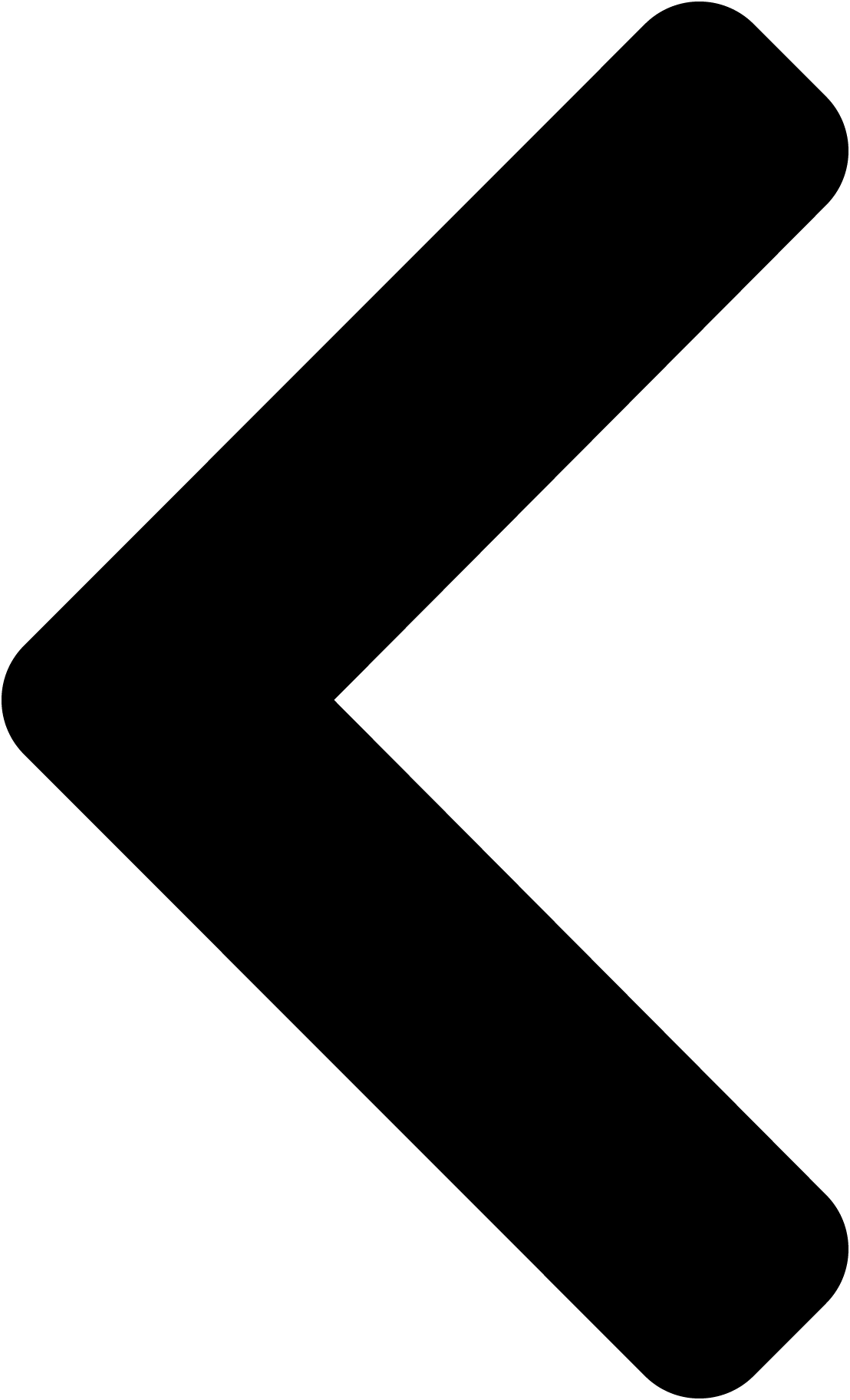
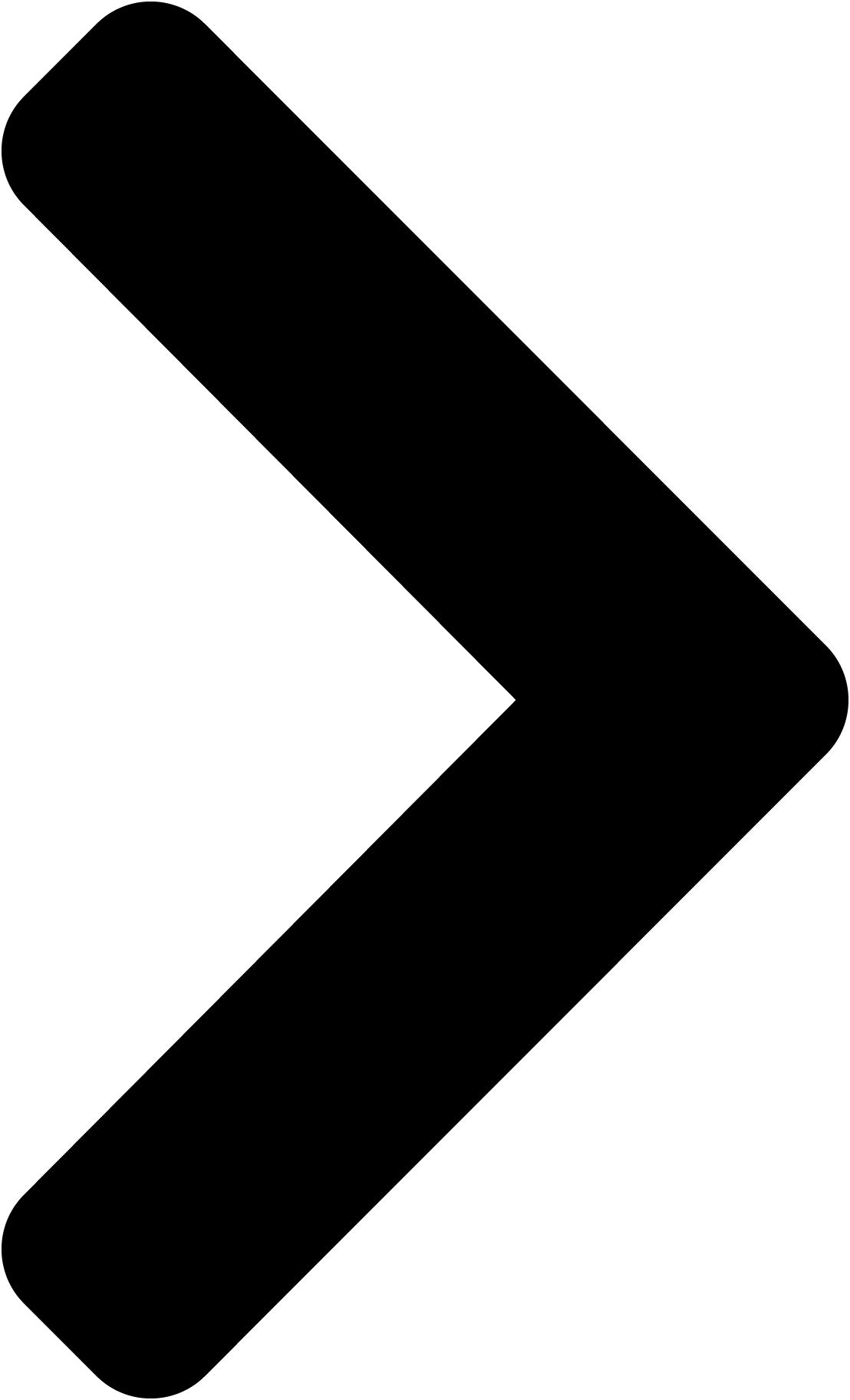
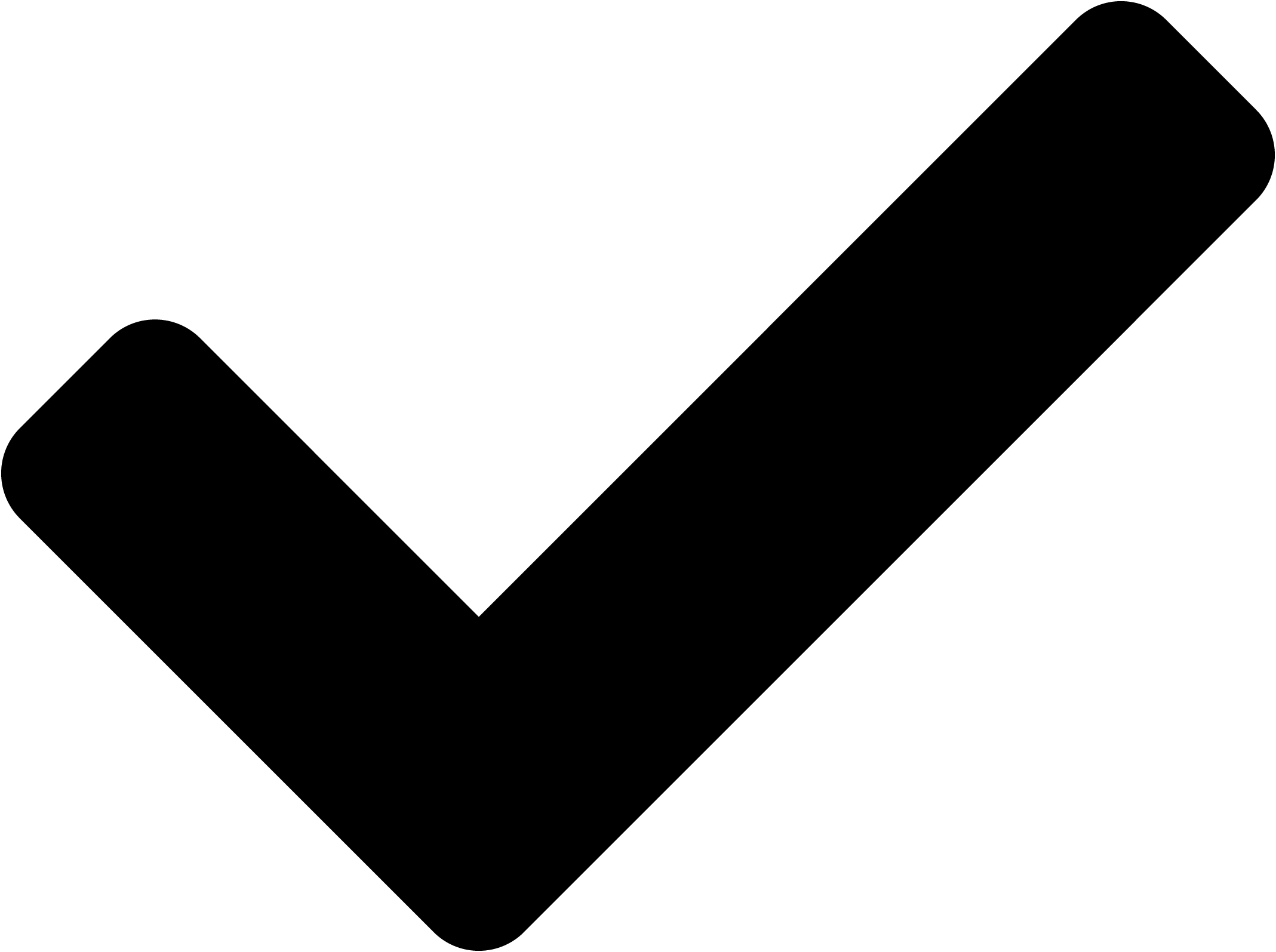
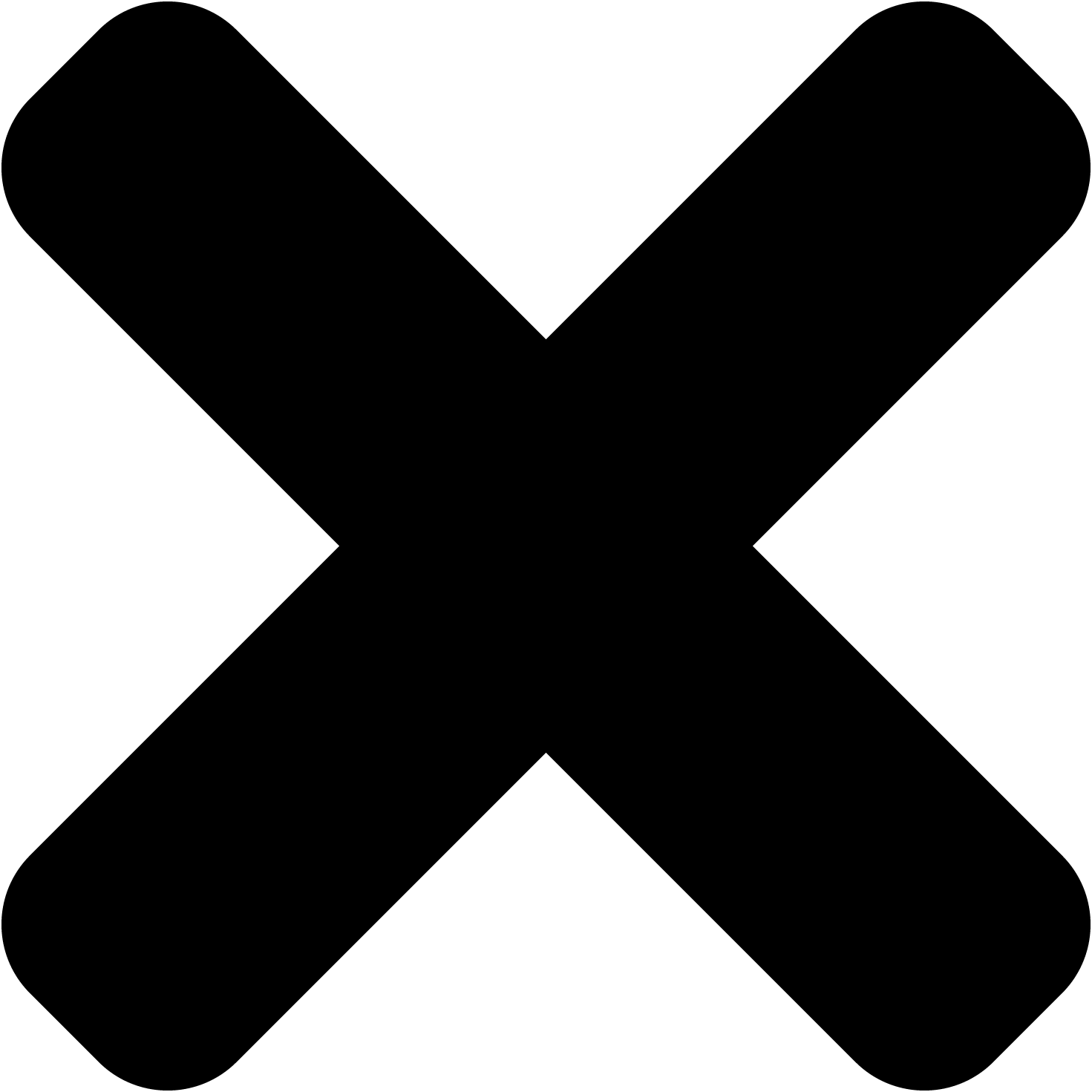
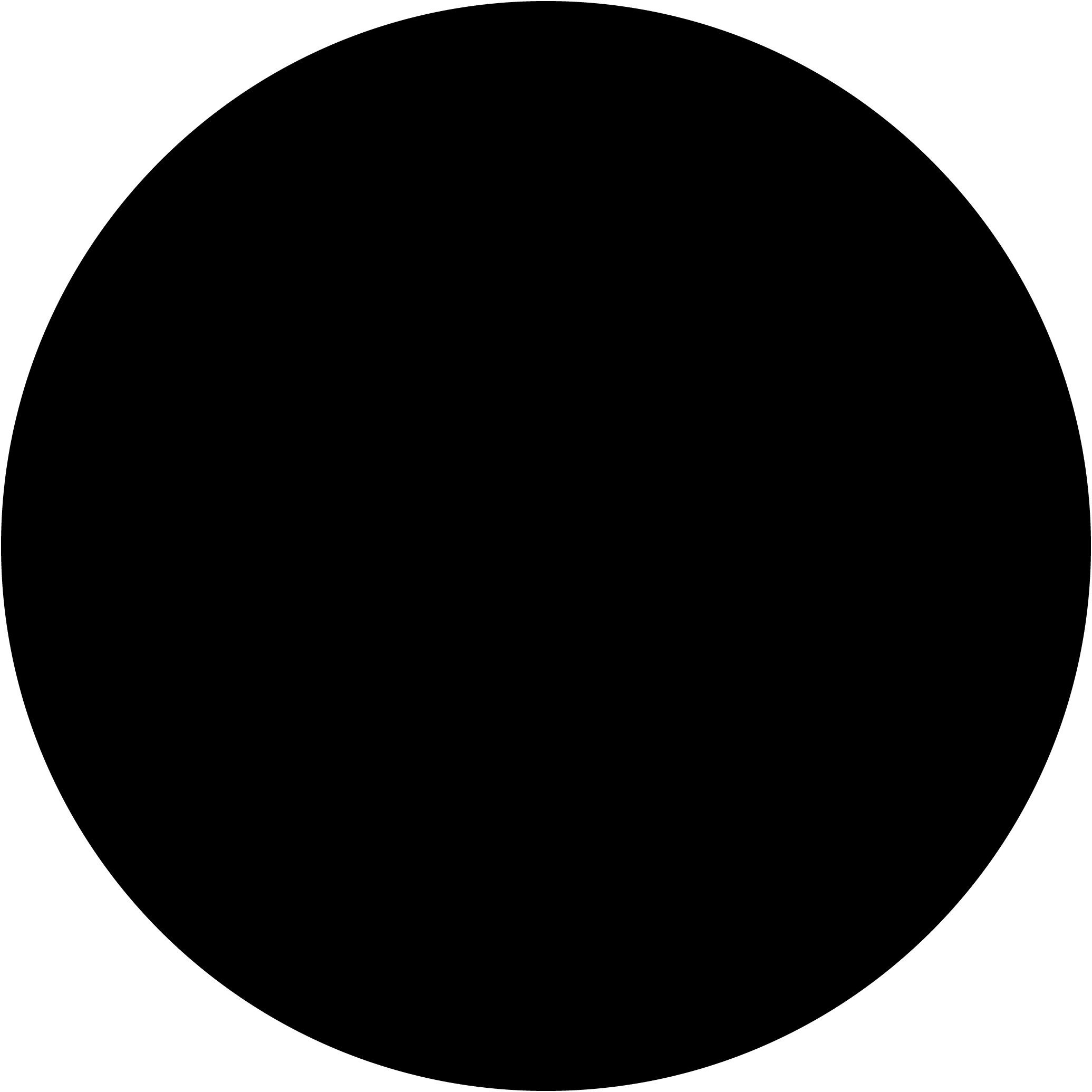
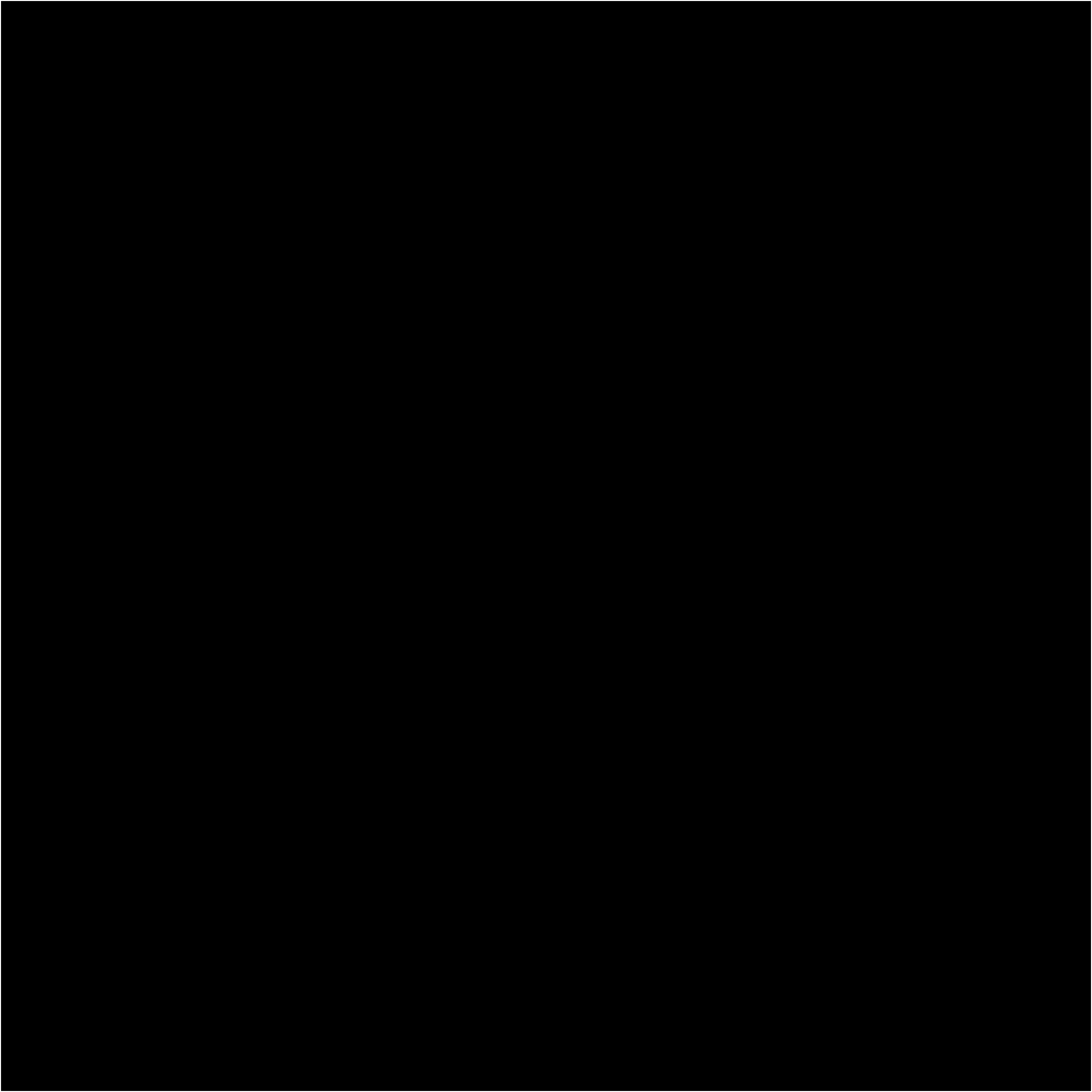
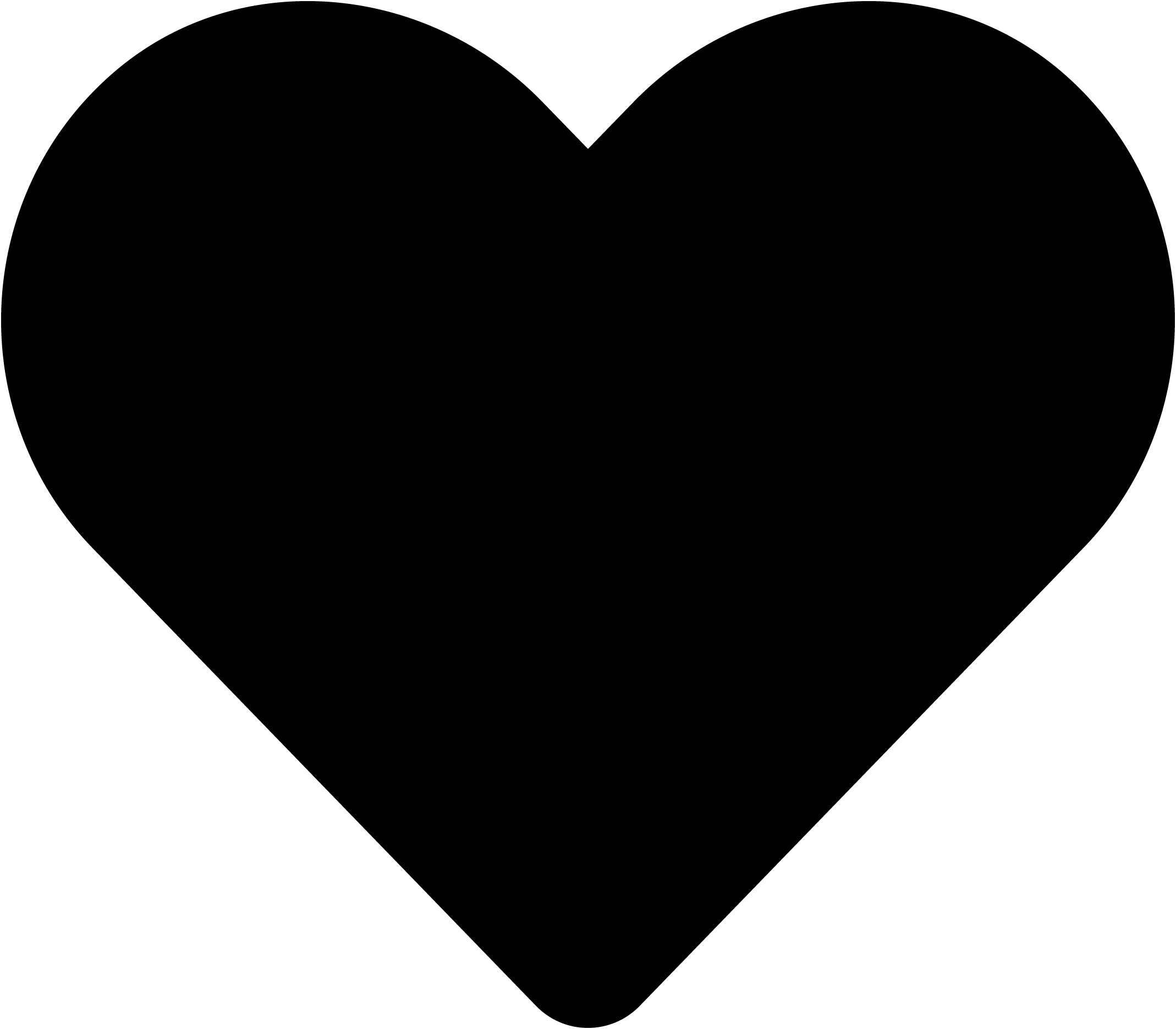
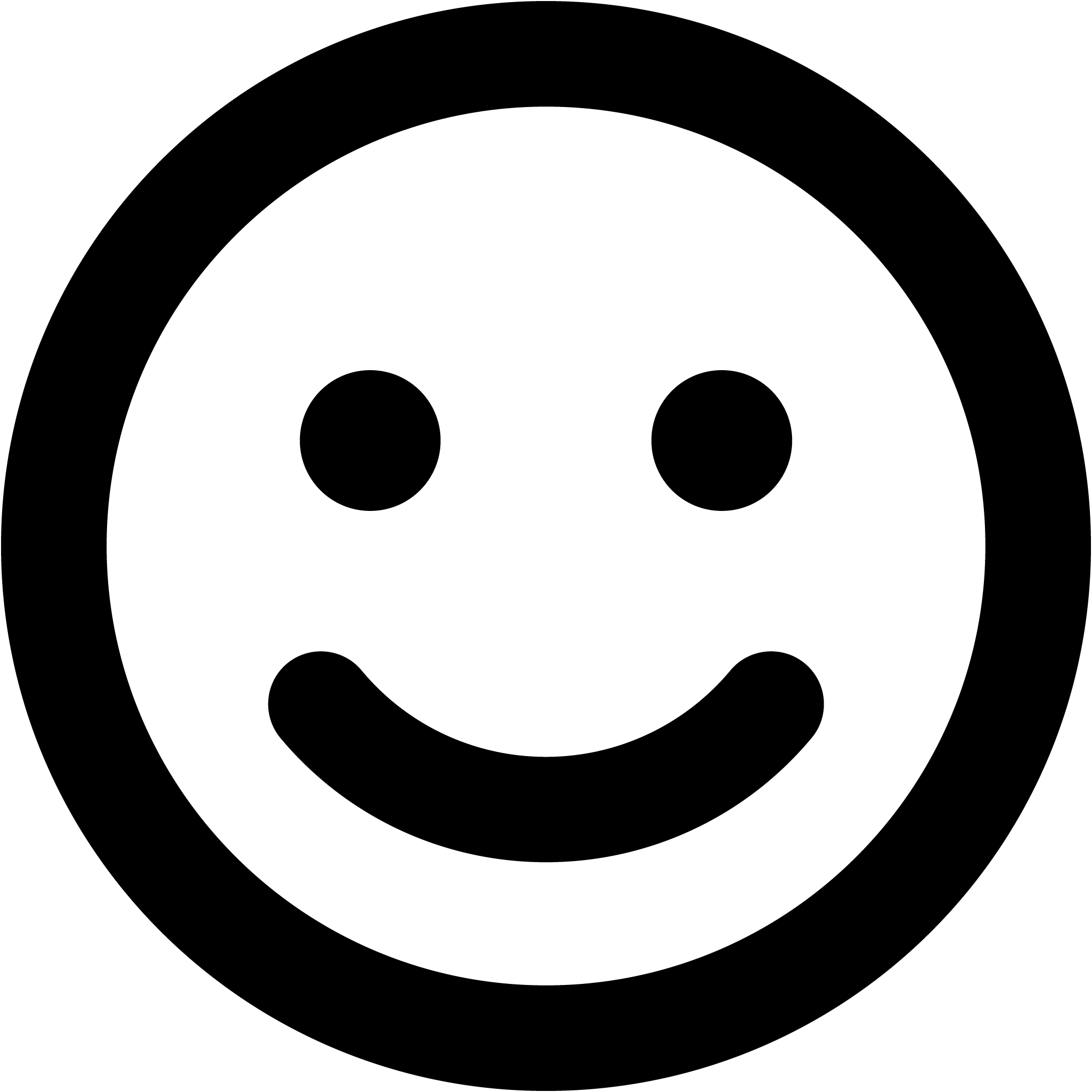
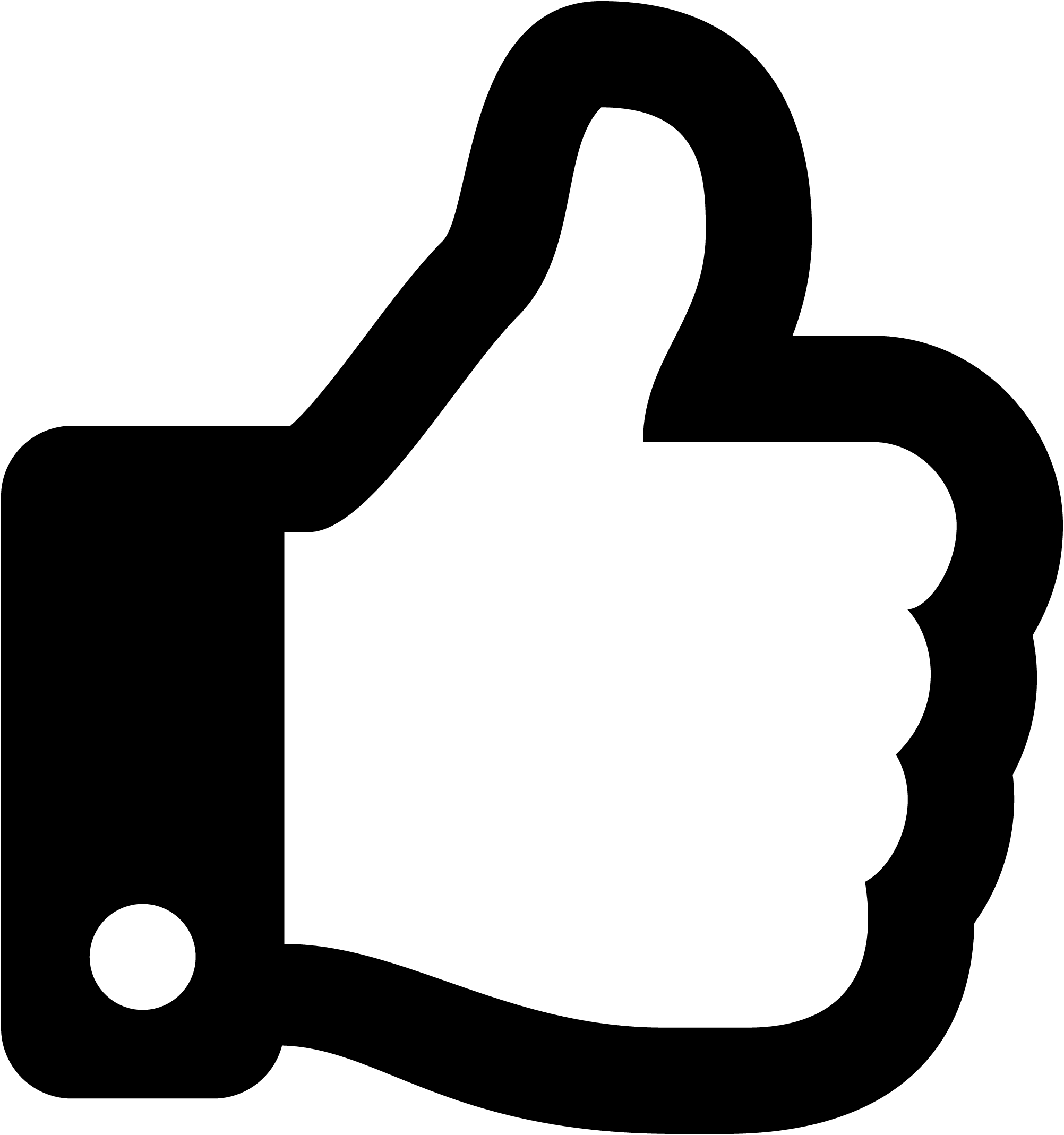
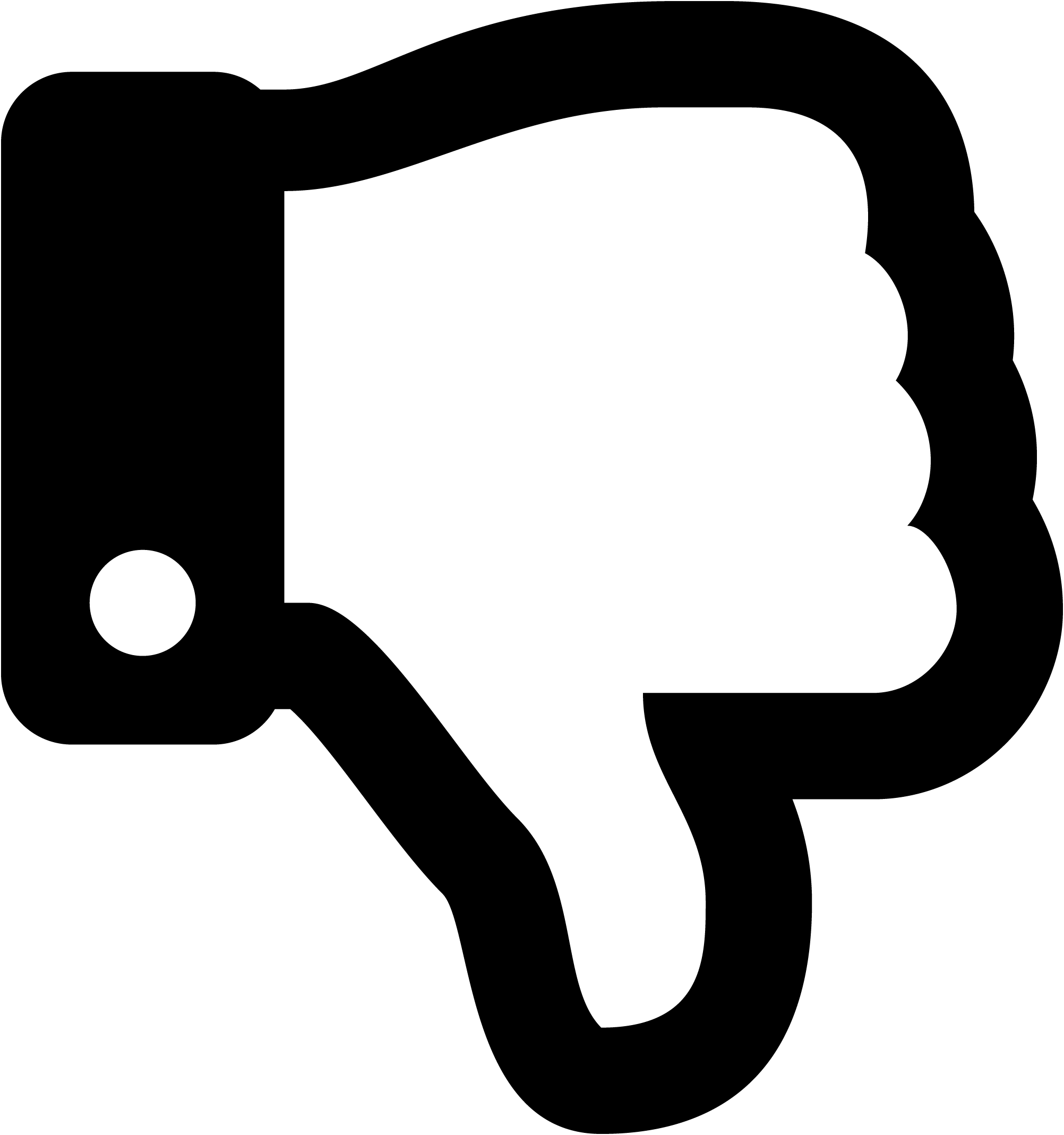